
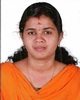
- Open Access
- Authors : Mini Bahuleyan , Sheela Devi Aswathy Chandran
- Paper ID : IJERTV11IS060351
- Volume & Issue : Volume 11, Issue 06 (June 2022)
- Published (First Online): 06-07-2022
- ISSN (Online) : 2278-0181
- Publisher Name : IJERT
- License:
This work is licensed under a Creative Commons Attribution 4.0 International License
Wearable Multifunctional Physiologicl Hybrid Sensing E-Skin
1.Mini Bahuleyan
(PG Scholor), Dept of ECE,
TKM Institute Of Technology, Karuvelil (Po) , ollam ,
2. Sheela Devi Aswathy Chandran
Assistant Professor, Department Of ECE, TKM Institute Of Technology, Karuvelil,
Abstract:- A multi-functional hybrid sensing interface with heterogeneous physiological detection capability, and system- level feasibility, two kinds of wireless system prototypes were also manufactured and functionally verified to provide healthcare signals such as ECG, pulse rate, body activity analysis. The results are monitored and displayed in a PC. The multi-functional system is designed to support physiological detection methodologies like electrometric, opto-metric and their hybrid, utilizing an in-house multi-functional e-skin device, in house flexible electrodes and IMU sensor and pulse sensor. For their functional verification, physiological detection capabilities were demonstrated using wearable device prototypes. Especially, the hybrid detection method includes an innovative continuous measurement of ECG signal and pulse rate while most previous wearable devices are not ready for it. Moreover, for effective implementation in the form of the wearable device, post-processing burden of the hybrid method was much reduced by integrating a proposed analog pre-processing scheme, where only simple counting process and calibration remain to estimate the acquired bio signals. This multi-functional sensor readout circuits and their hybrid- sensing interface are fully integrated into a single readout integrated circuit (ROIC). The materials for E-skin fabrication are analysed and PDMS is selected for E -skin development. E
-skin material is fabricated using PDMS and reduced graphene oxide and check the conductivity using impedance analyser
Keywords: Wearable Device, E-Skin, ECG sensor
,Multifunctional Physiological Detection
Wearable device technologies have been developed mostly as accessory type such as watches, wristbands and headsets. However, they have two major inherent limitations of insufficient functionality and unstable body contact. Most conventional wearable devices work as remote interfaces with smart phones, but their value-added functions are limited to few kinds like heart rate and body activity, where the heart rate is mostly measured by optical devices and the body activity is recognized by inertial sensors. The most fundamental problem of these accessory -type wearable device is that their body contact is unstable and thereby most electrode-based physiological sensing functions cannot be included together Therefore, many recent research interests have removed to a kind of body- attachable devices including patch-type or sticker-type devices, which can provide stable body contact and reliable electrode based physiological detection capability. For implementation of these attachable devices, their sensing electrodes and modules should be made flexible to provide comfortable body wearing. However, most flexible dry
electrodes have high impedance, and many body-oriented noises including motion artefacts are not effectively removed. As efforts to minimize this problem in the following detection front-end, there have been many circuit-level improvements in their readout schemes including impedance boosting , DC-servo loop and chopper modulation .While these circuit-level efforts try to integrate the electrode based physiological sensing functions into wearable devices, there have been another kinds of efforts to provide more functionality by utilizing new flexible sensing devices which include piezo-electric, piezo-resistive, and pyro-resistive devices .However, these researches are focused on device-level studies, and their detection circuits are not developed sufficiently. That is most detection scheme is based on single-ended readout circuits, and their detected signals are weak to various body-oriented or environmental noise . Another technical trendis a hybrid sensing that different kinds of sensors cooperate to provide a new kind of sensing capability. In healthcare applications, a pulse transit time (PTT) is measured by utilizing two different sensing paths of electrocardiogram (ECG) and photoplethysmogram (PPG) and its result is again used to estimate the blood pressure (BP) . related researches have been focused on their detection methodologies or post signal processing algorithms. However, their important signal processing works are performed in digital domain, and they also require lots of processing burdens Therefore, this work presents a flexible electro-physiological sensing interface for wearable healthcare monitoring systems and its multi- functionality and hybrid sensing capability are implemented in single readout integrated circuit (ROIC) which includes an impedometric readout path and two electrometric readout paths, utilizing two in-house sensor devices. The impedometric readout path performs body impedance analysis and resistive electronic skin (e-skin) sensing for pressure and temperature measurement, where a pseudo differential front-end structure with a ripple reduction scheme is proposed to improve ether resistive sensing accuracy. The electrometric readouts are designed to support low-noise measurement of the bio-potentials and the PPG using flexible electrodes and an optical device pair. Their pre-processing peak-detection scheme is also proposed to allow simultaneous peak extraction of the ECG and the PPG in analog domain, which enables the hybrid sensing capability on the PTT and the BP. Two system- level prototypes of a wireless smart phone monitoring and
a prosthesis control system are manufactured and functionally verified to capture eight representative healthcare signals: ECG, PPG, BP, electromyogram, body fat and skin temperature.
MULTIFUNCTIONAL SENSOR SYSTEM
Multi-functional healthcare interface architecture which consists of three input devices , common ROIC, a microcontroller (MCU), a Bluetooth module and application devices. Its flexible sensor module is manufactured on top of a flexible printed circuit board (PCB) to have hinged interconnect structures for comfortable wearing. In order to process eight kinds of healthcare signals, the input devices of an e-skin sensor, flexible electrodes, and a pair of LED and photodiode are utilized, and their device outputs should be converted to have electrical characteristics such as voltage, current, and resistance. For detection of pulse wave, respiration and skin temperature, the device outputs in the form of pressure and temperature are converted into resistances by utilizing an in-house e-skin sensor which is composed of polyvinylidenefluoride (PVDF) and reduced graphene oxide (r -GO). For the bio potential signal detection of ECG and EMG, in-house flexible electrodes are manufactured to deliver electrical signals through stable contacts with the skin. The PPG signal are converted into a voltage using a pair of LED and photodiode, and the body impedance is supported by the tetra-polar electrode measurement with the flexible module in direct contact with the skin. In order to accommodate these different kinds of healthcare signals, the ROIC is designed to provide one impedometric channel and two electrometric channels. These channels are selectively sampled by utilizing a multiplexer and a successive approximation register (SAR) analog-to-digital converter (ADC). The MCU controls these multi- functional detection modes of the ROIC, receiving digital conversion output of the ADC. It executes appropriate digital processing schemes for each signal kind, and also operates application devices such as a smartphone via Bluetooth Low-Energy (BLE) wireless communication. For the purpose of physiological monitoring, it is designed that various signals are selectively observed by controlling the sensor module through operation mode selection buttons in the smartphone application software. Figure 2.1 shows the Multifunctional physiological sensing system.
SYSTEM DESIGN REQUIREMENT IN HOUSE SENSOR DESIGN
PVDF rGO film for e- skin device, which is composed of flexible and micro structured ferroelectric composites. It allows discrimination of multiple stimuli through inherent multifunctional characteristics of pyro -resistive and piezo- resistive. Since this multifunctional device is in response to both temperature and pressure, the film was fabricated in planer and interlocked microdome structures in order to minimize interferences with each other. The measured pyro- resistive characteristic without pressure, where the measured resistance is changed from 1.85 M to 0.95 M as the temperature varies from 25 to 65 °C. Figure 3.1 shows the Microdome-patterned PVDF-rGO composite film and their
measurement. The pyro- resistive characteristic under different humidity conditions, resistances are almost the same for humidity variations. The measured piezo-resistive characteristic changes over a wide range of pressure, where the resistance range can be adjusted by tuning the rGO concentration. In low pressure range up to 2.5 KPa , the piezo resistive characteristic shows high sensitivity. Under different temperature and humidity conditions, the piezo- resistive characteristic changes, where its variation amount is much less than piezo-resistive changes in multi-functional physiological sensing system. In direct contact with human skin for physiological sensing, the pyro-resistive characteristic changes the resistance by 22.5 K per degree, and its sensitivity is sufficient in impedometric readout path.
Fig. 1:Simplified block diagram of proposed multi-functional ROIC.
Flexible electrodes based on metallic Nano wires have been used intensively for a variety of optoelectronic applications because of excellent conductivity, transparency, and flexibility. fabricated by highly conductive and robust silver Nano wire (AgNW)-based flexible electrode that is coated on a polydimethyl siloxane (PDMS) substrate, where the AgNW network is randomly oriented on the surface. For usage answerable electrodes, it should be flexible and sufficiently robust to be applied for sensing interfaces. Negligible resistance changes of the fabricated flexible electrode under bending radius from 10 mm to 1.5 mm, and repetitive bending of 1000 times in worst distortion of 1.5 mm bending radius was also performed to produce negligible resistance variations. Conventional wet electrodes have excellent signal quality due to low electrode impedance, but they rapidly deteriorate the contact condition
when their moisture orgel dries. Conventional dry electrodes may experience unstable contact with human movement because they are mainly made of a metallic material. Simplified block diagram of proposed multi-functional ROIC is totally flexible, so that it can stabilize the signal quality on wearing condition and its durability can be maintained even after quit times.
MULTIFUNCTIONAL ROIC DESIGN
The multi-functionalities from the PVDF-Rgo device and the flexible electrodes are embodied in the ROIC to support their desired physiological sensing capabilities of the proposed fully differential multi-functional healthcare ROIC, which is made up of two electrometric readout paths and one impedometric readout path. The electrometric readout path processes bio-electrical signals from the flexible electrodes or the LED photodiode pair, and the impedometric readout path detects the body impedance or the e-skin devices resistance which can be changed by physical stimuli such as pressure (piezo-resistive)and temperature (pyro resistive).For their digital conversions
,One of these readout paths is sequentially selected and digitally converted via a 12-bit SAR ADC which has the signal to noise and distortion ratio(SNDR) of 63.3 dB and effective number of bits (ENOB) of10.22bits[19]. The ROIC includes the serial peripheral interface(SPI) for data transfer with the MCU and also a low-dropout regulator, a clock generator, and a bandgap reference. A peak detector output of each channel is also available through this SPI, which supports a hybrid sensing and reduces a post-processing of sensor signals.
IMPEDOMETRIC READ OUT IMPLEMENTATION
The impedometric readout consists of a pseudo-differential resistance-to-voltage converter (PDRVC), a pseudo- sinusoidal current generator (PSCG), a peak detector, a rectifier, and an active band-pass filter (BPF) which can be reconfigured as a programmable-gain amplifier (PGA). These sub-blocks are partially activated or reconfigured to provide an appropriate path depending on the sensing targets. For detecting the e-skin devices resistance, the PD- RVC, the BPF, and the peak detector are activated to provide its readout path. For functionality of the body impedance analysis (BIA), the PSCG, the PGA, and the rectifier are utilized. For supporting various kinds of resistance or impedance detections, it is necessary to provide wide detection range while it maintains their detection accuracy. Front-end circuit implementation of the e-skin sensor readout path. conventional wide-range readout circuits that is based on the resistive divider have single-ended structure, the chopper modulated pseudo- differential converting technique in adopted in the PD- RVC to obtain wide-range differential output signals, where 3-bit tunable resistors (RREF) are included for wide- range operation. For this wide-range resistance detection, two amplifiers are arranged in parallel to get a differential output from single-ended sensing resistance input by alternating the signal path through chopper switches. However, the usage of the chopper modulation causes output ripples due to amplifier.
ELECTROMETRIC READOUT IMPLEMENTATION
In electrometric readout path different kind of sensors co- operate to provide the sensing capability. ECG sensor – ecg100c amplifier provide ECG from human. TSD 200 PPG transducer operates with PPG 100c amplifier record blood volume and pulse wave via optical method. EEG sensor directly provide result. Body fat sensor send electrical signal to the body . Speed of the signal indicates resistance corresponds body fat. When measuring bio potential signals such as ECG and EMG, there are many non-ideal factors that seriously degrade signal quality upon signal acquisition, electrode offset, amplifier offset, and electrode impedance mismatch. a low noise amplifier (LNA) for the electrometric readout front-end, where its fully-differential circuits are simplified as single-ended. State-of-the-art artefact- reduction techniques are adopted in this work as follows: the flicker noise is avoided by using the chopper stabilization technique, and the chopper-induced ripples from amplifier offset are reduced by adding the ripple reduction loop Additionally, non-ideal effects on the body-electrode contact are minimized by including the DC-servo loop (DSL) and the impedance boosting loop. For implementation of the DSL, its low frequency filter requires high-resistance which costs large area. For smaller area, previous studies have used pseudo-resistors or switched resistors. Considering one research summary on Giga ohm- level on-chip resistors we adopted the switched resistor because its variations are much smaller than other high- resistance implementation techniques and also its equivalent resistance (Req)can be easily adjusted by changing the duty- cycle (D) of the switching pulse. Following this LNA, the PGA is implemented as switched-capacitor type to provide adjustable gain stage for supporting multiple bio-potential sign.
PHYSIOLOGICAL CHARACTERISATION PROCESS
Among multiple physiological signals measured from the proposed ROI, the body impedance and the peak information of the ECG-PPG hybrid sensing should be converted to estimate the body fat and the blood pressure through appropriate post-processing procedures, while most other signals including ECG, BP , body fat and skin- temperature would be meaningful only by their signal monitoring
ELECTRONIC SKIN USING PDMS
The skin is one of the main organs of the human body and as such it implements many different and relevant functions,
-
- protection of the inner body organs, detection of cutaneous stimuli, etc. Due to its complexity, the development of artificial, or better, electronic skin (e-skin) is a very challenging goal which involves many different and complementary research areas. Nonetheless, the possible application areas are many and very relevant: e.g. humanoids and industrial robotics, artificial prosthetics, biomedical instrumentation, cyber physical systems, for naming a few. Many research groups are addressing the development of e-skin and the research scenario is exciting and continuously evolving. Due to its very peculiar features, the development of electronic skin can be effectively tackled
using a holistic approach. Starting from the system specification definition, the mechanical arrangement of the skin itself (i.e. soft or rigid mechanical support, structural and functional material layers, etc.) needs to be designed and fabricated together with the electronic embedded system, to move toward aspects such as tactile data processing algorithms and the communication channel interface. In this paper, we present and assess the achievements of our research group in this field focusing on the following aspects:
- The manufacturing technology of sensor arrays based on piezoelectric polymer (PVDF) transduction
- The mixed-mode interface electronics
- The tactile data processing algorithms
- The electronic embedded system. Future trends and research perspectives will be also presented.
- protection of the inner body organs, detection of cutaneous stimuli, etc. Due to its complexity, the development of artificial, or better, electronic skin (e-skin) is a very challenging goal which involves many different and complementary research areas. Nonetheless, the possible application areas are many and very relevant: e.g. humanoids and industrial robotics, artificial prosthetics, biomedical instrumentation, cyber physical systems, for naming a few. Many research groups are addressing the development of e-skin and the research scenario is exciting and continuously evolving. Due to its very peculiar features, the development of electronic skin can be effectively tackled
Fig .2:. Components of E-Skin
Electronic skins (e-skins) with monitoring capabilities have attracted extensive attention and are being widely employed in wearable devices for medical diagnosis. In particular, e-skins based on strain sensors have been reported extensively due to their simple structure and efficient performance in collecting human physiological information. Flexible sensors with high sensitivity, simplified fabrication, and low-cost are highly desired for human signal monitoring; this work provides a novel strain-sensing e-skin with micro-structures, which is simply made of modified polydimethylsiloxane (PDMS) and silver nano wires (Ag NW). The fabricated e-skin has great sensitivity towards strain changes, and its mechanical properties and sensitivity could be regulated by varying the micro-structures. Furthermore, the e-skin demonstrated significant capacity monitoring human body movements, temperature changes, and spatial resolution, highlighting its great potential in personalized medicine.
MATERIALS USED IN E SKIN
Polydimethylsiloxane (PDMS), also known as dimethylpolysiloxane or dimethazone, belongs to a group
of polymeric organosilicon compounds that are commonly referred to as silicones. PDMS is the most widely used silicon-based organic polymer, as its versatility and properties lead to many applications.
Reduced graphene oxide (RGO) is the form of GO that is processed by chemical, thermal and other methods in order to reduce the oxygen content, while graphite oxide is a material produced by oxidation of graphite which leads to increased interlayer spacing and functionalization of the basal planes of graphite.
RESULT
Fig 3: ECG sensor connection with Arduino
Fig 4: E skin material
Acquisition of signal using fabricated e skin.
CONCLUSION
Electronics skin is one such device that depicts the beauty of electronics and its uses in daily life. The electronic devices gain more demand in the market when they are in compact in size and best in functioning. Multi-functional hybrid sensing used to detect physiological detection. It also provide mental and physical relaxation. multi- functional hybrid sensing interface with heterogeneous physiological detection capability ,where two kinds of in- house flexible sensor devices are manufactured and their reconfigurable readout circuits with digital conversions are fully integrated in a single ROIC. Two kinds of readout front-end circuits such as impedometric and electrometric were integrated together, embedding their inherent reduction schemes of noises and ripples. They also included their own peak detectors for hybrid-sensing capability and post processing burden reduction. For system-level feasibility, two kinds of wireless system prototypes were also manufactured and functionally verified to provide eight representative healthcare signals of ECG, EMG, PPG, BP, body fat, pulse wave, respiration,
and skin temperature, whose results are wirelessly monitored on a smartphone. This work would contribute to advancement of continuous BP monitoring and e-skin combined tactile sensing systems.
REFERENCES
[1] M.Konijnenburg et al., A multi(bio)sensor acquisition system with integrated processor, power management, 8 × 8 LED drivers, and simultaneously synchronized ECG, BIO-Z, GSR, and two PPG readouts, IEEEJ. Solid-State Circuits, vol. 51, no. 11, pp. 2584 2595, Nov. 2016. [2] S.H.Woo,Y.Y.Choi,D. J.Kim, F.Bien, and J. J.Kim, Tissue- informative mechanism for wearable non-invasive continuous blood pressure monitoring,Sci. Rep., vol. 4, 2014, Art. no. 6618. [3] M. Etemadi, O. T. Inan, J. A. Heller, S. Hersek, L. Klein, and S. Roy, A wearable patch to enable long-term monitoring of environmental, activity and hemodynamics variables, IEEE Trans. Biomed. Circuits Syst., vol. 10, no. 2, pp. 280288, Apr. 2016. [4] S. P. Lee et al., Highly flexible, wearable, and disposable cardiac biosensors for remote and ambulatory monitoring, npj Digit. Medicine, vol. 1,2018, Art. no. 2. [5] jang, K.-I.; Han, S.Y.; Xu, S.; Mathewson, K.E.; Zhang, Y.; Jeong,J.-W.; Kim, G.-T.; Webb, R.C.; Lee, J.W.; Dawidczyk, T.J.; et al. Rugged and breathable forms of stretchable electronics with adherent composite substrates for transcutaneous monitoring. Nat. Commune. 2014, 5, 4779. [CrossRef] [PubMed] [6] Yu, R.J.; Park, H.; Jin, S.W.; Hong, S.Y.; Lee, S.S.; Ha, J.S. Highly
stretchable and sensitive strain sensors using fragmentized graphene foam. Adv. Funct. Mater. 2015, 25, 42284236.
[7] Rogers, J.A.; Someya, T.; Huang, Y. Materials and mechanics for stretchable electronics. Science 2010, 327, 16031607. [CrossRef] [PubMed] [8] Song, J. Mechanics of stretchable electronics. Curr. Opin. Solid State Mater. Sci. 2015, 19, 160170. [CrossRef] [9] Park, S.-I.; Ahn, J.-H.; Feng, X.; Wang, S.; Huang, Y.; Rogers, J.A. Theoretical and experimental studies of bending of inorganic electronic materials on plastic substrates. Adv. Funct. Mater. 2008, 18, 26732684. [CrossRef] [10] Kim, D.H.; Ahn, J.H.; Choi, W.M.; Kim, H.S.; Kim, T.H.; Song,J.; Huang, Y.Y.; Liu, Z.; Lu, C.; Rogers, J.A. Stretchable and foldable silicon integrated circuits. Science 2008, 320, 507511. [CrossRef] [PubMed] [11] Huang, X.; Cheng, H.; Chen, K.; Zhang, Y.; Zhang, Y.; Liu, Y.; Zhu, C.; Ouyang, S.C.; Kong, G.W.; Yu, C. Epidermal impedance sensing sheets for precision hydration assessment and spatial mapping. IEEE Trans. Biomed. Eng. 2013, 60, 28482857. [CrossRef] [PubMed] [12] Chen, Y.; Lu, B.; Chen, Y.; Feng, X. Breathable and stretchable temperature sensors inspired by skin. Sci. Rep. 2015, 5, 11505. [CrossRef] [PubMed] [13] Kim, D.H.; Song, J.; Choi, W.M.; Kim, H.S.; Kim, R.H.; Liu, Z.;
Huang, Y.Y.; Hwang, K.C.; Zhang, Y.W.; Rogers, J.A. Materials and noncoplanar mesh designs for integrated circuits with linear elastic responses to extreme mechanical deformations. Proc. Natl. Acad. Sci. USA2008, 10, 1867518680. [CrossRef] [PubMed] [14] Khang, D.Y.; Jiang, H.; Huang, Y.; Rogers, J.A. A stretchable form of single-crystal silicon for high-performance electronics on rubber substrates. Science 2006, 311, 208212.