
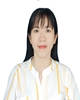
- Open Access
- Authors : Le Tran Uyen Tu, Nguyen Thi Tuyet Trinh, Dung Thi Hoai Trang, Vo Thanh Tung
- Paper ID : IJERTV9IS040249
- Volume & Issue : Volume 09, Issue 04 (April 2020)
- Published (First Online): 24-04-2020
- ISSN (Online) : 2278-0181
- Publisher Name : IJERT
- License:
This work is licensed under a Creative Commons Attribution 4.0 International License
Influence of K/ Na Component Ratio on Physical Properties of KNN Ceramics
Le Tran Uyen Tu, Nguyen Thi Tuyet Trinh, Dung Thi Hoai Trang, Vo Thanh Tung
Physics Faculty, University of Sciences, Hue University Hue City, Vietnam
Abstract In recent years, considerable efforts have been made toward the control of processing techniques, volatile mass and component ratio of Potassium and Sodium in order to improve physical properties of KNN ceramics. In this paper, the influence of physical properties of the (KxNa1-x)NbO3 ceramics, fabricated using conventional solid state reaction method, on K/ Na component ratio was examined. The densities of ceramic sample changed depending on the variation of x parameter from
-
- to 0.52 mol %. The highest value of density is 4.26 g/cm3 at potassium content x of 0.48 mol %, coresponding with the uniform in microstructure of ceramic surface. Effects of K/ Na ratios in the vicinity of morphotropic phase boundary on microstructure, ferroelectric and piezoelctric properties are also examined. The (K0.48Na0.52)NbO3 system sintered at 1090 oC shows the highest values of remanent polarization Pr=3,19
C/cm2 at the applied coercive electric field Ec=7,16 kV/cm. At x=0.48 mol %, electromechanical coupling factor kp of 0.26 and the piezoelectric constant d33 of 69 pC/N also can be obtained, related to homogenous grains size of orthohombic phase..
Keywords: Ratio of K/ Na, piezoelectric, KNN-based lead-free ceramics.
- INTRODUCTION
In previous decades, piezoelectric ceramic systems basing on titanium zirconate lead (PZT) are widely studied and applied in many fields[1-3]. However, due to the toxicity of lead [4, 5], the development of lead-free piezoelectric ceramic systems with exellent electric and piezoelectric properties to replace lead based ceramics in different devices is necessary [6].
Among lead-free systems, ceramics based on (Na, K)NbO3 (KNN) have attracted a lot of attention due to their prominent ferroelectric properties, high Curie temperature (about 420 oC) and environmentally friendly, capable of replacing the lead based ceramics. However, it is difficult to manufacture KNN ceramics with a high density and good electrical properties using conventional sintering process due to the high volatility of alkaline elements at high sintering temperature [7, 8]. In additional, the ratio of K/ Na in the vicinity of morphotropic phase boundary (MPB) is also one of the main reasons affecting the properties of KNN ceramics. Some reports suggested that the KNN ceramic with the ratio of K/ Na components at 0.5/ 0.5 has exellent dielectric and piezoelectric properties due to the existence of morphological phase boundary between two phases [9, 10]. Meanwhile, other reports issued physical properties of the KNN system are dominated by presence of not morphotropic phase boundary but polymorphic phase boundary [11, 12]. In addition, the
exact K/ Na component ratio to obtain KNN ceramics with prominent characteristics is sensitive with raw compositions. That is, the effect of K/ Na ratio on the physical properties of KNN ceramics has been still controversial.
In this paper, ceramics with the chemical formula (KxNa1- x)NbO3 are made using conventional solid state reaction methods. The changes in structural characteristics, dielectric, ferroelectric and piezoelectric properties of fabricated ceramics when potassium component (x) varies from 0.47 to
0.52 mol % has been carried out. Results of this paper hope to contribute the understanding about microstructure and physical properties of KNN ceramics depending on the change of ratio potassium/ sodium component.
- EXPERIMEMTAL
The general formula of the studied material was (KxNa1- x)NbO3, where x are 0.47, 0.48, 0.49, 0.50, and 0.52 mol %; respectively. Reagent grade oxide powders (purity 99 %) of K2CO3, Na2CO3, Nb2O5 were used as raw materials. Before being weighed, the K2CO3 and Na2CO3 powders were dried in an oven at 200 °C for 2 h to minimize the effect of moisture. Mixed powder was milled in 8 hours to obtain an appropriate distribution of the particle size, the small particle size will improve the reactivity of the powders [13]. Two calcinations at temperature 850 °C for 2 h were then performed to obtain compositionally homogeneous powders and the single phase formation [14]. Thereafter the calcined, powders were ball milled again for 16 h to create a more uniform distribution of powders and the reaction occurs completely [14]. The ground materials were pressed into disk 12 mm in diameter and 1.5 mm in thick under 100 MPa. In order to limit the evaporation of alkaline elements, these pellets were covered by the powders with the same composition and then were sintered in a sealed alumina crucible at the temperature of 1090 °C for 2 h
The crystal structures of the sintered samples were examined by X-ray diffraction with CuK radiation, = 0.15406 nm (XRD, D8 ADVANCE-Bruker). The grain morphology of the samples was examined by scanning electron microscopy (Novanano SEM 450-Fei). The densities of samples were measured by Archimedes method. The ceramic samples were poled in a silicone oil bath at 80 °C by applying dc field of 30 kV/cm for 20 min then cooling under the same electric eld. They were aged for 24 h prior to testing.
The dielectric constant at room temperature is calculated by measuring the capacitance C of samples using RLC HIOKI 3532 at frequency of 1 kHz. The ferroelectric property was measured by Sawyer-Tower method. The piezoelectric properties were determined from the resonance and antiresonance frequency by using an Impedance Analyzer HP 1493A and RLC HIOKI 3532.
- RESULTS AND DISCUSSION
Fig. 1(a) shows the density of the (KxNa1-x)NbO3 ceramic samples sintered at 1090 °C as a function of the Potassium (K) content. The (K0.47Na0.53)NbO3 ceramics shows low density,
4.08 g/ cm3. With increasing of K content, the density initially increases and reaches the maximum value of 4.26 g/ cm3 at
0.48 mol % Potassium, then decreases. These results are consistent with microstructure of fracture surfaces of the samples as shown in Fig.1(b)-1(f). As shown in Fig. 1(b), the microstructure of the (K0.47Na0.53)NbO3 consisted of irregular large and small grains, the discrete distributions, porous. However, the microstructure of samples becomes denser and more uniform as the Potassium content increases from 0.47 to
0.48 mol % (Fig. 1(c)).
Fig. 1. The Potassium content dependence of the density of KNN ceramics measured at room temperature and the SEM micrographs of (KxNa1-x)NbO3 ceramics with x=0.47 (b), x=0.48(c), x=0.49 (d), x=0.50 (e) and x=0.52 (f).
Fig. 1(d), (e) and (f) show that with further increasing Potassium content to 0.52 mol %, the particle size increased sharply. In addition, the shape and size of particles were no onger uniform. Such with the 0.48 mol % Potassium, homogeneous microstructure of ceramic was obtained.
Fig. 2 shows the XRD patterns at room temperature with the 2 range from 20 o to 80 o of (KxNa1-x)NbO3 ceramic samples sintered at 1090 °C. It can be seen that all samples with x from 0.47 to 0.52 (mol %) exhibited single phase structure of perovskite ABO3, without trace of secondary phases. In order to distinguish whether the fabricated samples
have orthorhombic or tetragonal structure, the intensities of the
Fig. 2. X ray diffraction patterns of the (KxNa1-x)NbO3 ceramics
double peaks in the X-ray diffraction patterns are further considered. The corresponding XRD patterns characterized by the double peaks in which intensity of (110) and (220) peaks higherthan that of (001) and (020) peaks respectively. According to Skidmore and co.authors report, this indicated that all ceramic samples have orthorhombic phase [15]. In addition, the intensity of the double diffraction peaks in Fig. 2 is the same through all x values. This result shows that the phase structure of KNN system is completely unaffected by the changes of K/ Na component ratio in the range x = (0.470.52) mol %. The changes of potassium content also significantly affect electric properties of (KxNa1-x)NbO3 ceramics.
Fig. 3 shows the room temperature dielectric constant and dielectric loss tan measured at 1 kHz frequency of (KxNa1-x)NbO3 ceramics as a function of the potassium contents (x). The dielectric constant increases with the rise in potassium content and reaches value (391) at x=0.48. However, when x 0.49, the dielectric constant decreases. This is related to density and microstructure of ceramics. The varying tendency of dielectric loss tangent with the potassium contents is inverse to that of the measured dielectric constant. The minimum value of dielectric loss obtained at x=0.48 mol
% potassium is 0.07.
Fig. 3. Room-temperature dielectric constant and dielectric loss tan of KNN ceramics as a function of the potassium (x mol %) content
Fig. 4. Hysteresis loops of (K0.48Na0.52)NbO3 ceramic sample measured at room temperature
Fig. 4 shows the shape of ferroelectric hysteresis loops of (K0.48Na0.52)NbO3 sample measured at room temperature. Then, the remanent polarization Pr and the coercive field EC of ceramics as a function of the potassium contents (x) were determined, as shown in Fig. 5. Experimental results show that pure KNN ceramics has a typical hysteresis loop of ferroelectric materials. With increasing of potassium component (x % mol), initially the remanent polarization tends to increase, reaches the maximum value of Pr= 3.19 C/ cm2 at x= 0.48 mol %, and then deacreases. Meanwhile, the coercive field decreased to the lowest value of Ec = 7.26 kV/ cm as the same potassium content, after that raises again.
To determine piezoelectric properties of (KxNa1-x)NbO3 ceramics sitering at 1090 oC, resonant vibration spectra of samples were measured at room temperature. From these resonant spectra, piezoelectric parameters of samples were obtained.
Fig. 6 shows the electromechanical coupling factors of radial vibration mode (kp) and piezoelectric constant (d33) changing as a function of the potassium content. At 0.48 mol % of potassium, the electrical parameters became prominent due to the well-arrangement of microstructure of the ceramics.
Fig. 6. The potassium content dependence of the values kp and d33 of (KxNa1- x)NbO3 ceramics
The largest values for kp of 0.26, d33 of 69 pC/ N, reached at
x=0.48 mol %. And then piezoelectric parameters rapidly decrease when potassium content continue raising. These properties of (KxNa1-x)NbO3 ceramics at x of 0.48 mol % corresponding the microstructures of well-faceted and homogeneously distributed grains.
- CONCLUSION
- INTRODUCTION
- to 0.52 mol %. The highest value of density is 4.26 g/cm3 at potassium content x of 0.48 mol %, coresponding with the uniform in microstructure of ceramic surface. Effects of K/ Na ratios in the vicinity of morphotropic phase boundary on microstructure, ferroelectric and piezoelctric properties are also examined. The (K0.48Na0.52)NbO3 system sintered at 1090 oC shows the highest values of remanent polarization Pr=3,19
The lead-free KNN ceramics with different K/ Na ratios in the vicinity of morphotropic phase boundary were fabricated. All samples has simple perovskite structure. The effect of ratios of K/ Na component on the structure, microstructure and dielectric, ferroelectric properties of (KxNa1-x)NbO3 ceramics was studied. The KNN ceramic with x = 0.48 mol % sintered at 1090 oC shows smallest values of dielectric loss and coercive field. The best dielectric constant, remanent polarization, electromechanical coupling factors, and piezoelectric constant were obtain at potassium component content of 0.48 mol % of KNN ceramic, corresponding to the highest density and homogenous grains size.
REFERENCES
- K. Uchino, Piezoelectric Actuators and Ultrasonic Motors.Kluwer Academic Publishers, Boston, (1997).
- B. Jae, W. R. Cook, and H. Jae (Eds.), Piezoelectric Ceramics, Academic, New York (1971).
- Y. Xu (Eds.), Ferroelectric Materials and Their Applications, Elsevier Science, Amsterdam-London-New York-Tokyo (1991).
- Saito Y, Takao H, Tani T, Nonoyama T, Takatori K, Homma, Nagoya, T, Nakamura M., Lead-free piezoceramics,Nature 432, 84-87 (2004).
- In-Young Kang, In-Tae Seo, Yu-Joung Cha, Jae-Hong Choi, Sahn Nahm,Tae-Hyun Sung, Jong-Hoo Paik, Low temperature sintering of ZnO and MnO2-added (Na0.5K0.5)NbO3 ceramics. Journal of the European Ceramic Society 32, 23812387 (2012).
- EU-Directive 2002/96/EC, Waste Electrical and Electronic Equipment (WEEE), O. J. Eur. Union, 46 [L37] 2438 (2003).
- Jing-Feng Li, Ke Wang, Fang-Yuan Zhu, Li-Qian Cheng, and Fang- Zhou Yao, (K,Na)NbO3-Based Lead-Free Piezoceramics: Fundamental Aspects, Processing Technologies, and Remaining Challenges, J. Am. Ceram. Soc.,96 36773696 (2013).
- Wenxin Ma, Xinghua Fu1, Wenhong Tao, Lei Yang, Guoyuan Cheng and Liping Zhao, KNN-Sb lead-free piezoelectric ceramics
Fig. 5. The remnant polarization (Pr) and coercive field (Ec) of (KxNa
1-x)NbO3
synthesized by hydrothermal method, Materials Science Forum, Vol. 859, pp 3-7 (2016).
ceramics as a function of the potassium (x mol %) content
- V. J. Tennery and K. W. Hang, Thermal and X-Ray Diffraction Studies of NaNbO3KNbO3 System, J. Appl. Phys., 39 [10] 474953 (1968).
- Y. J. Dai, X. W. Zhang, and K. P. Chen, Morphotropic Phase Boundary and Electrical Properties of K1_xNaxNbO3 Lead-Free Ceramics, Appl. Phys. Lett., 94 [4] 042905, 3pp (2009).
- Y. Dai, X. Zhang, G. Zhou, Phys. Lett, 90 (2007).
- F. Rubio-Marcos, J. J. Romero, M. G. Navarro-Rojero, and J. F. Fernandez, Eect of ZnO on the Structure, Microstructure and Electrical Properties of KNN-Modied Piezoceramics, J. Eur. Ceram. Soc., 29, 304552 (2009).
- Dai Y., Zhang X. and Zhu G., Phase transitional behavior in K0.5Na0.5NbO3 -LiTaO3 ceramics, Appl. Phys. Lett., 90, 262903 (2007).
- T.A. Skidmore, T. Stevenson, T.P. Comyn, S.J. Milne Phase Development During Mixed-Oxide Processing of a [Na0.5K0.5NbO3]1-x