
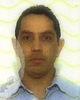
- Open Access
- Authors : Selene I. Nava-Rosales , Raul Cortes-Martinez , Ruth Alfaro-Cuevas-Villanueva
- Paper ID : IJERTV9IS120245
- Volume & Issue : Volume 09, Issue 12 (December 2020)
- Published (First Online): 01-01-2021
- ISSN (Online) : 2278-0181
- Publisher Name : IJERT
- License:
This work is licensed under a Creative Commons Attribution 4.0 International License
Equilibrium, Kinetic and Thermodynamic Studies on Adsorption of Direct Blue 71 from Aqueous Solutions by Calcium Alginate Beads
Selene I. Nava-Rosales1, Raul Cortes-Martinez1,
1Facultad de QuÃmico FarmacobiologÃa, Universidad Michoacana de San Nicolás de Hidalgo,
Morelia, Michoacán, México.
Ruth Alfaro-Cuevas-Villanueva2, 2Instituto de Investigaciones en Ciencias de la Tierra, Universidad Michoacana de San Nicolás de Hidalgo,
Morelia, Michoacán, México.
Abstract This study examined the feasibility for removing Direct Blue 71 (DB71) from aqueous solutions using calcium alginate beads (CABs). The effects of dye concentration, CABs dosage, temperature and pH on adsorption of DB71 by CABs were evaluated by batch type adsorption experiments using CABs and DB71 aqueous solutions. Pseudo second-order, Elovich and Lagergren models were adopted to evaluate experimental data and thereby elucidate the kinetic adsorption mechanism, as well as kinetic parameters of the system. Additionally, this study used the Langmuir, Freundlich, Langmuir-Freundlich (L-F) and Dubinin-Radushkevich (D-R) isotherm models to describe equilibrium adsorption. The adsorption percentage of DB71 increased as dye concentration and CABs dosage increased. Adsorption experiments were performed at three different temperatures (25, 35 and 45°C) and at different pH conditions (pH 3-9). The maximum adsorption capacity (q0) was reached at 35°C and at pH of 6. Based on the fittings of experimental data to Pseudo second-order, Elovich and Lagergren models, it can be established that the adsorption of DB71 onto CABs follows Pseudo-first order kinetics, described by the Lagergren model. The equilibrium adsorption of DB71 is best described by the L-F isotherm at 35°C, suggesting that the mechanism involved on this system is related to chemisorption on heterogeneous surfaces.
KeywordsAlginate; biosorption; dye; Direct Blue 71; removal
-
INTRODUCTION
Environmental pollution is inherent to the advance of modernity and negatively affects public health and ecosystem equilibrium. Meanwhile, water pollution, caused by the discharge of effluents coming from industries that use or produce dyes, represents a growing problem. An estimated quantity of 10000 tons per year of dyes is used worldwide and could be discharged into aquatic waste streams [1]. Direct Blue 71 (DB71) is an azoic dye, a complex organic compound used in textile, plastics, cosmetics, leather, printing, etc., with high worldwide consumption [2, 3]. To minimize the environmental pollution generated by such effluents, they must be treated before discharged into the water bodies. This treatment is given to less than 25% of industrial effluents in Mexico before its release.
The release of direct dyes into the water bodies set toxic conditions for aquatic living beings [4]. The discharge of wastewater with undesirable color onto the hydrosphere affects its translucent capacity, affecting water bodies' photosynthetic activities [5]. Azoic dyes' complex structures provide them resistance to heat, light, and microbial action, so its natural degradation becomes a complicated process [6]. Degradation of these dyes by oxidant agents generates potentially toxic, mutagenic, and carcinogenic amino- aromatic compounds [7, 8]. These compounds can have acute and chronic effects on exposed organisms depending on the exposure time and dye concentration. In humans, dyes can cause allergic dermatitis, skin irritation, cancer, mutation, etc. [1].
Many treatment methods have been developed to remove azoic dyes from aqueous solutions, such as the Fenton process, photocatalytic treatment, biodegradation, coagulation-flocculation, nanofiltration, ozonation, oxidation, reverse osmosis, and many others [9]. Adsorption has proved to be more effective than the other techniques, particularly in terms of initial cost, possible regeneration at low cost, recovery of the sorbate, simplicity of design, operation, and insensitivity to toxic substances [10]. The most effective adsorbent material is activated carbon; however, it has a high recovery cost. Hence there is the need to find a low-cost, commercially available material for the adsorption of dyes.
A wide variety of materials have been investigated as low-cost alternatives to activated carbon for removing dyes, such as zeolites [9], guava seeds carbon [11], carbon nanotubes [12], chitosan-based hydrogels [13], Spirulina and Chlorella algae [14], among others. Alginate is a complex polysaccharide extracted from brown seaweeds and microalgae, representing an abundant biomass source on the planet [15]. Chemically, alginate comprises varying proportions of D-mannuronic acid (M) and L-guluronic acid (G). These compounds are arranged in MM or GG blocks, interspersed with MG blocks [16]. Its unique property of forming water-insoluble calcium alginate (CA) gel through ionotropic gelation with Ca+2 ions has made it possible to encapsulate both macromolecular agents [1719] and low molecular weight agents [2022].
CABs have been used to remove different dyes such as malachite green [23], methylene blue, and methyl orange
[24]. Still, no reports of using such biosorbent removing Direct Blue 71 are mentioned in the literature. CABs have the potential to act as an effective adsorbent for the removal of dyes from wastewaters. Calcium alginate (CA) is a good biosorbent because of its natural origin, biocompatibility, low production and extraction cost, and possible regeneration. These properties allow CA utilization in pharmaceutical, food, and water biotechnology applications. Therefore, the present study's main objective is to analyze the efficiency of adsorption of DB71 in aqueous solutions by CABs under different pH, temperature, CABsdosage, and initial dye concentration conditions.
-
MATERIALS AND METHODS
-
Preparation of Calcium Alginate Beads (CABs)
Firstly, a 2% (w/v) solution of sodium alginate (SA) was prepared as follows: 10 g of SA was mixed with 500 mL of distilled water at 50°C. The suspension was maintained at constant agitation for 3 h until a viscose and clear solution was obtained. Afterward, 1000 mL of 150 mM calcium chloride (CaCl2) solution was prepared. For the formation of CABs, SA solution was dripped in intervals of 1 second approximately into the beaker that contained the CaCl2 solution in constant agitation (60 rpm). The CABs formed were left in the CaCl2 solution for 1 h, then washed three times with distilled water and dried in a heater at 100°C for 5 h. The dehydrated CABs were then used in adsorption experiments.
-
Fourier Transform Infrared Analysis (FTIR)
FTIR spectral data were used to characterize CABs and elucidate whether specific functional groups are responsible for the adsorption of DB71 onto CABs and understand the mechanism of this phenomenon. The analyses of CABs before and after DB71 adsorption were carried out with an FTIR spectrophotometer (Bruker FTIR, model Tensor 27). Spectral scanning was performed in the range of 4000 – 400 cm-1.
-
Adsorption kinetics
DB71 adsorption kinetics was determined by performing batch-type experiments at different contact times. These tests were carried out at 25°C and at a constant agitation speed of 130 rpm. CABs dosage, dye concentration, and dye solution volume were kept constant at 1 g, 50 mg/L, and 10 mL, respectively. CABs and dye solution were maintained in contact for 2 h. Absorbance was measured to the supernatants at 590 nm by a UV-VIS spectrophotometer, at different time intervals; thereby, dye concentration was obtained along contact tie, as well as removal percentage.
-
Effect of adsorbent dose
Batch-type experiments were performed to determine the dosage effect over DB71 removal. These experiments were carried out with different amounts of CABs (g) and
10 mL of DB71 solution at 50 mg/L. The tests were conducted into plastic bottles (25 mL) that contained CABs from 0.1 to 0.9 g. One plastic bottle without CABs was used as the control. The bottles were stirred continuously at a speed of 130 rpm, at 25°C and 1 hour of contact time. Once the contact time was reached, the concentration of dye in the supernatants was determined, as mentioned above.
-
Influence of pH
Batch-type contact tests were performed to determine the influence of pH on DB71 adsorption. Thus, a 50 mg/L DB71 solution was prepared and then placed into plastic flasks, each with 50 mL of the solution. The pH of each flask's solutions was modified from 3 to 9 using HCl 0.1M and NaOH 1M and measured with a pH meter OrionStar serial A11. Then, 10 mL of each dye solution and CABs optimal dosage were put in contact for 1 hour at 25°C and 130 rpm. Supernatants were separated and analyzed by UV- Vis method, as mentioned above.
-
Adsorption isotherms
Adsorption isotherms were performed at 25, 35, and 45°C, in which the initial dye solution concentration varied from 10 to 500 mg/L. 10 mL of each dye solution at pH 6 and CABs optimal dosage were put in contact for 1 h at 130 rpm. Then, supernatants were separated and analyzed by the UV-Vis method, as mentioned above. All sorption experiments were performed by triplicate to determine reproducibility.
-
-
RESULTS AND DISCUSSION
-
FTIR analysis
Figure 1 shows the FITR spectra of calcium alginate beads (CABs) and DB71 adsorbed on CABs. Both signals exhibit peaks at 3445, 1624, 1429, and 1034 (cm-1), attributed to O-H, C=O, C-OH, and OC-OH groups. The wide hydroxyl bands in both spectra can be attributed to the sum of the contributions from many O-H groups present in the polymer [25]. Also, the ratio of weak intensities of the bands corresponding to C=O, C-OH, and OC-OH suggests deprotonated carboxylic groups, that is, anions [26]. The displacement of these broad bands to a higher transmittance percentage in the presence of DB71 is probably caused by chemical interaction between CABs and dye functional groups. Even though the most reactive functional groups of the DB71 molecule are generally the sulfonate groups, these groups are negatively charged, just as the functional groups of the CABs. Thus, the chemical interaction does not involve the sulfonate groups but the positive group of the DB71 that is a primary amine [27]. Figure 1 shows both spectra of CABs before and after DB71 adsorption. All bands show a decrease in intensity, probably due to the generalized degradation of the alginate structure. The bands of functional groups that shifted to a lower frequency were involved in the uptake of DB71 [26].
0.5
0.4
qt (mg/g)
qt (mg/g)
0.3
0.2
0.1
0.0
Experimental data
_______ Elovich, R = 0.99336
– – – – – – – Lagergren, R = 0.99991
__ __ __ PSO, R = 0.99990
Fig. 1. FTIR spectra of CABs and DB71 adsorbed on CABs.
-
Adsorption kinetics
Adsorption kinetics results of DB71 onto CABs as a function of contact time are shown in Figure 2. It is observed that the CABs maximal adsorption capacity is reached in short times, and the qe value corresponds to 0.3472 mg/g, at a contact time of 30 minutes. This behavior is probably due to a process involving physical contact and chemical interactions between specific functional groups of the calcium alginate and the dye.
Pseudo-second-order (PSO) [28], Elovich, and Lagergren kinetics models [29] were adopted to evaluate experimental data and determine the system's kinetic parameters. The best fit was observed with the Lagergren model, shown in Figure 3, with a correlation coefficient of 0.9999. This fact suggests that the system follows pseudo- first-order kinetics. DB71 dye is in excess concerning CABs, so it controls the reaction velocity. Also, it is considered that dye concentration stays constant during contact time. System kinetics parameters obtained by the Statistica software are shown in Table 1. The parameters are: qe represents the adsorption capacity of CABs at equilibrium (mg/g); K1 is the pseudo-first-order kinetic constant (1/min); K2 is the pseudo-second-order rate constant of sorption (mg/g.min); and are Elovich constants related to the initial adsorption rate (mg/g.min) and the desorption rate (mg/g), respectively.
Fig. 2. CABs equilibrium capacity for DB71 removal (mg/g) as a function of contact time (min).
Fig. 3.
0 20 40 60 80 100 120 140
Time (min)
Fig. 4. DB71 adsorption by CABs as a function of time fitted to the Lagergren, pseudo-second-order (PSO) and Elovich models.
TABLE I. KINETIC PARAMETERS OF DB71 ADSSORPTION BY CABS (T=25°C).
Lagergren
Pseudo-second-order
Elovich
K1
qe
R2
K2
qe
R2
R2
0.675
0.344
0.999
3.898
0.6928
0.999
36.03
0.67
0.99
Table 2 shows a comparison of the pseudo-first-order and pseudo-second-order adsorption constants at an initial DB71 concentration of 50 mg g-1and 25°C. Kinetic parameters of 5 different adsorbent materials for BD71 are compared in Table 2. The pseudo-first-order kinetic constant for CABs resulted in being one order of magnitude higher than the first-order kinetic constant for activated carbon (TPAC), rice husk carbon-clinoptilolite composite (RH) are two orders of magnitude higher than the one for wheat shells (WS) [2729]. Also, the pseudo-second-order rate constant of sorption for CABs was three orders of magnitude higher than the one for TPAC, PPC, RH, and four orders of magnitude higher than WS. These facts mean that the adsorption process using CABs is faster than for other materials, which represents an advantage for utilizing the proposed system as it implies a time reduction on dye wastewater treatment.
-
Effect of adsorbent dose
The influence of different dosages of CABs (g/L) is shown in Figure 4. It was observed that the increase of CABs dosage improved the adsorption process. Maximal removal percentage, which also implies the adsorbent's saturation with DB71, was reached at a dosage of 70 g/L. At higher amounts of CABs, removal percentage presented a slight decrement probably caused by the dye agglomeration around the adsorbent material [33].
-
Influence of pH
In this test, the adsorption capacity of DB71 onto CABs at seven different pH conditions from 3 to 9 was evaluated. qe was plotted versus different pH values, as shown in Figure 5. It can be observed that DB71 removal raised as pH increased from 3 to 6. From this pH value, no significant increase in adsorption capacity was observed. This behavior can be attributed to the dye's cationic nature and to
carboxylate groups of alginate, which interact with the positively charged dye via strong interactions, suggesting that an electrostatic mechanism for DB71 removal is involved in the alginate-dye adsorption system. Besides, electrostatic repulsions between carboxylate groups expand the alginate chains making the adsorption sites more accessible to the dye. For pH<4.5, the carboxylate protonation becomes significant, and the adsorption decreases [34].
TABLE II. KINETIC PARAMETERS OF DB71 ADSSORPTION BY CABS (T=25°C).
Material
Lagergren
Pseudo-second-order>
Reference
K1
qe
R2
K2
qe
R2
CABs
0.6750
0.3440
0.999
3.898
0.692
0.999
This work
PAC
0.0540
58.82
0.954
0.00109
68.03
0.997
[30] H
0.0175
45.47
0.965
0.0006
54.38
0.997
[31] S
0.0011
6.16
0.925
0.000138
6.26
0.991
[32] Fig. 5. Effect of CABs dosage in the DB71 adsorption process
Fig. 6. Effect of pH on DB71 adsorption by CABs.
-
Adsorption isotherms
Adsorption capacity (qe) of DB71 onto CABs versus final concentration of dye solutions after 1 hour of contact time, for three different temperatures, were plotted and shown in Figure 6. An increase in the temperature leads to
an increase in dye initial concentration to achieve the same adsorption capacity, reached with lower temperatures in an initial phase of the isotherm. However, the adsorption capacities for the three different temperatures are close to each other in all range of dye solution concentrations.
DB71 uptake slightly decreases with increasing the temperature, after a dye concentration of 70 mg/L. This behavior suggests that an exothermic process controls the adsorption of DB71 onto CABs. Normal dye wastewater temperature variations do not significantly affect the overall decolorization performance [35].
Langmuir, Freundlich, and Langmuir-Freundlich (L-F) isotherm models were chosen to describe equilibrium adsorption. The L-F isotherm best fitted experimental data at 35°C, as shown in Figure 7, with a correlation coefficient of 0.9959, suggesting that the mechanism involved in this system is related to chemisorption on heterogeneous surfaces; this means that the adsorption mechanism implies functional groups, which are responsible for the chemisorption between the dye and calcium alginate chemical structures.
Experimental data
– – – – – – Langmuir, R = 0.97834
_______ Freundlich-Langmuir, R = 0.98929
__ __ __ Freundlich, R = 0.96870
Experimental data
– – – – – – Langmuir, R = 0.97834
_______ Freundlich-Langmuir, R = 0.98929
__ __ __ Freundlich, R = 0.96870
5
5
4
4
3
3
2
2
qe
qe
Fig. 7. DB71 adssorption isotherms on CABs at 25, 35 and 45°C.
0
20
40
60
Ce
80
100
120
0
20
40
60
Ce
80
100
120
1
1
0
0
Fig. 8. Isotherm data fitted to Langmuir-Freundlich (L-F), Freundlich and Langmuir isotherm models at 25°C.
The three isotherm models at 25, 35, and 45°C are indicated in Table 3. For the Langmuir isotherm, which also obtained a high correlation coefficient, q0 represents the maximal theoretical adsorption capacity of the CABs. This parameter (q0) was higher at 35°C than at 25 and 45°C, with a value of 16.27 mg/g. Besides, the parameter b (L/mg) is related to adsorption's energy, whereas K and n in the L-F and Freundlich models indicate the relative capacity and the adsorption intensity, respectively.
-
Thermodynamic parameters
Experimental data were fitted to the Dubinin- Radushkevich (D-R) model to determine if the ion exchange process is determinant in the adsorption process [36]. The D-R isotherm [37] assumes a heterogeneous surface (equation 1):
TABLE III. LANGMUIR-FREUNDLICH (L-F), FREUNDLICH AND
T (ºC)
L-F
Freundlich
Langmuir
K
n
a
KF
n
qo
b
25
0.0086
1.6797
0.0223
0.1006
1.2172
11.3597
0.0060
35
0.0015
1.8631
0.0130
0.0451
1.1151
16.2780
0.0021
45
0.0096
1.3200
0.000029
0.0052
0.6852
11.5465
0.0051
T (ºC)
L-F
Freundlich
Langmuir
K
n
a
KF
n
qo
b
25
0.0086
1.6797
0.0223
0.1006
1.2172
11.3597
0.0060
35
0.0015
1.8631
0.0130
0.0451
1.1151
16.2780
0.0021
45
0.0096
1.3200
0.000029
0.0052
0.6852
11.5465
0.0051
LANGMUIR ISOTHERM PARAMETERS.
qe = Xmexp(-K2) (1)
the FTIR analysis of this adsorbate-adsorbent system at 25
ºC. Consequently, these findings indicate that the DB71 sorption mechanism could be different than merely chemisorption when the temperature rises. Besides, G's negative values reveal that the adsorption of DB71 is thermodynamically feasible and spontaneous [40]. Enthalpy change (H) and entropy change (S) when CABs is the adsorbent for DB71 were also calculated in this article (equation 4) [41]:
Ce = C1(1-Fe) (4)
Where Ce is the concentration of the dye in the solution at equilibrium (mg/L), C1 is the initial concentration of DB71 in the adsorbent at equilibrium (mg/L) and Fe is the fractional conversion of the sorption at equilibrium.
Kc = Fe/(1-Fe) (5)
Equation 5 shows that the equilibrium constant is independent of the quantity of adsorbent and the volume of sorption. The equilibrium constants were calculated at three different temperatures. The thermodynamic parameters were calculated using these values and the vant Hoff equation (equation 6) [42]:
Ln Kc = S/R H/RT (6)
Parameter
25 ºC
35 ºC
45 ºC
Xm (mg/g)
0.00075
0.0372
0.0592
K (mol2/kJ2)
-0.008
-0.017
-0.016
E (kJ/mol)
7.9056
5.4232
5.5901
R2
0.967
0.859
0.882
Parameter
25 ºC
35 ºC
45 ºC
Xm (mg/g)
0.00075
0.0372
0.0592
K (mol2/kJ2)
-0.008
-0.017
-0.016
E (kJ/mol)
7.9056
5.4232
5.5901
R2
0.967
0.859
0.882
TABLE IV. DUBININ-RADUSHKEVICH (D-R) ISOTHERM PARAMETERS FOR DB71 ADSORPTION ON CABS.
Where (the Polanyi potential) = RT ln(1+(1/Ce)) qe is
the amount of DB71 ions adsorbed onto CABs (mol/g); Xm is the adsorption capacity of CABs (mg/g); Ce is the equilibrium concentration of DB71 ions in solution (mol/L); K is a constant related to the adsorption energy (mol2/kJ2); R is the ideal gas constant; and T is the temperature (K). The D-R isotherm can be expressed in linear form (equation 2) [38]:
Ln qe = Ln Xm – K2 (2)
Xm and K were calculated from the slopes and intercepts of the plots of Ln qe vs. 2. The energy of sorption, defined as the change of energy when one mole of the ion is transferred to the surface of the solid, can be calculated using the following expression (equation 3) [39]:
G = -E = (-2k)-1/2 (3)
The parameter E is related to the reaction mechanism. If E is between the values 8 and 16 kJ/mol, ion exchange is the system's main sorption process. If the value is lower than 8 kJ/mol, then the physical sorption is the main sorption mechanism [39]. Table 4 shows four parameters for DB71 adsorption on CABs obtained with the D-R isotherm at three different temperatures.
High correlation coefficients were obtained with the D- R isotherm adjustment; this indicates that the DB71 adsorption process by CABs could also be described with this model. The adsorption energy values (E) are under 8 kJ/mol for two tested temperatures (35 and 45 ºC); this suggests that physical forces dominated the sorption process and that these forces were more important than ion exchange and particle diffusion at these temperatures [38]. However, this fact does not agree with the facts found by
Where Kc is the equilibrium constant, S is the change in the entropy of the process, H is the change in the enthalpy of the process and T is the temperature (K).
The values of H and S were calculated from the slopes and intercepts of the plots of Ln Kc as a function of 1/T for DB71 (graph not shown). For the adsorption of DB71 on CABs the values of H = -27,386.31 J/mol and S = -79.49 J/mol K were obtained. Negative values of H suggest exothermic sorption of the dye onto CABs, and the negative value of S reflects the decreased randomness at the solid/solution interface during the adsorption of dye on CABs. This is a direct consequence of: (i) opening up of alginate structure, (ii) enhancing the mobility and extent of penetration within the alginate, and (iii) overcoming the activation energy barrier and enhancing the rate of intraparticle diffusion [43].
-
-
CONCLUSIONS
Calcium Alginate Beads demonstrated effective removal of the industrial dye, Direct Blue 71, from aqueous solutions since removal percentages up to 80% were obtained and a maximal dye adsorption capacity of 16.27 mg/g was achieved in 43 minutes of contact time. Best adsorption results were obtained with pH alkaline values, which imply that the primary amine present in the DB71 molecule participates in the adsorption process. Besides, the temperature which allowed the best dye uptake was 35°C.
However, the dye uptake difference between the three temperatures is not significant; normal wastewater temperature variations would not significantly affect the overall decolorization performance, so it is unnecessary to change the effluent temperature to use this system. Calcium Alginate is an organic compound, abundant, with a low production cost, and its use does not imply an environmental risk. For this reason, it is demonstrated that the proposed system in this study is a viable option for its use in wastewater treatment contaminated with azoic colorants such as Direct Blue 71.
ACKNOWLEDGMENT
The authors are thankful to S.A. Valencia-Leal for technical support.
REFERENCES
-
R. A. Gopal, M. Song, D. Yang, T. Lkhagvaa, S. Chandrasekaran, and D. Choi, Synthesis of hierarchically structured -Fe2O3PPy nanocomposite as effective adsorbent for cationic dye removal from wastewater, Environ. Pollut., vol. 267, p. 115498, dic. 2020, doi: 10.1016/j.envpol.2020.115498.
-
R. F. Fard et al., Efficiency of multi walled carbon nanotubes for removing Direct Blue 71 from aqueous solutions, Eurasian J. Anal. Chem., vol. 13, n.o 3, Art. n.o 3, 2018.
-
R. Khani, S. Sobhani, M. H. Beyki, and S. Miri, Application of magnetic ionomer for development of very fast and highly efficient uptake of triazo dye Direct Blue 71 form different water samples, Ecotoxicol. Environ. Saf., vol. 150, pp. 54-61, abr. 2018, doi: 10.1016/j.ecoenv.2017.12.018.
-
A. A. Ahmad, B. H. Hameed, and N. Aziz, Adsorption of direct dye on palm ash: Kinetic and equilibrium modeling, J. Hazard. Mater., vol. 141, n.o 1, pp. 70-76, mar. 2007, doi: 10.1016/j.jhazmat.2006.06.094.
-
M. Matto and Q. Husain, Redox-mediated decolorization of Direct Red 23 and Direct Blue 80 catalyzed by bioaffinity-based immobilized tomato (Lycopersicon esculentum) peroxidase, Biotechnol. J., vol. 3, n.o 9-10, pp. 1224-1231, 2008, doi: https://doi.org/10.1002/biot.200800049.
-
C. I. Pearce, J. R. Lloyd, and J. T. Guthrie, The removal of colour from textile wastewater using whole bacterial cells: a review, Dyes Pigments, vol. 58, n.o 3, pp. 179-196, sep. 2003, doi: 10.1016/S0143-7208(03)00064-0.
-
J. Shim, G.-Y. Kim, K.-H. Yeon, S.-H. Cho, J.-J. Woo, and S.-H. Moon, Degradation of azo dye by an electroenzymatic method using horseradish peroxidase immobilized on porous support, Korean J. Chem. Eng., vol. 24, n.o 1, pp. 72-78, ene. 2007, doi: 10.1007/s11814-007-5012-5.
-
E. J. Rodrigues de Almeida, D. E. Christofoletti Mazzeo, L. R. Deroldo Sommaggio, M. A. Marin-Morales, A. Rodrigues de Andrade, and C. R. Corso, Azo dyes degradation and mutagenicity evaluation with a combination of microbiological and oxidative discoloration treatments, Ecotoxicol. Environ. Saf., vol. 183, p. 109484, nov. 2019, doi: 10.1016/j.ecoenv.2019.109484.
-
S. Madan, R. Shaw, S. Tiwari, and S. K. Tiwari, Adsorption dynamics of Congo red dye removal using ZnO functionalized high silica zeolitic particles, Appl. Surf. Sci., vol. 487, pp. 907-917, sep. 2019, doi: 10.1016/j.apsusc.2019.04.273.
-
I. K. Kapdan and F. Kargi, Simultaneous biodegradation and adsorption of textile dyestuff in an activated sludge unit, Process Biochem., vol. 37, n.o 9, pp. 973-981, abr. 2002, doi: 10.1016/S0032- 9592(01)00309-0.
-
M. P. Elizalde-González and V. Hernández-Montoya, Removal of acid orange 7 by guava seed carbon: A four parameter optimization study, J. Hazard. Mater., vol. 168, n.o 1, pp. 515-522, ago. 2009, doi: 10.1016/j.jhazmat.2009.02.064.
-
C.-Y. Kuo, C.-H. Wu, and J.-Y. Wu, Adsorption of direct dyes from aqueous solutions by carbon nanotubes: Determination of equilibrium, kinetics and thermodynamics parameters, J. Colloid Interface Sci., vol. 327, n.o 2, pp. 308-315, nov. 2008, doi: 10.1016/j.jcis.2008.08.038.
-
G. Crini, G. Torri, E. Lichtfouse, G. Z. Kyzas, L. D. Wilson, and N. Morin-Crini, Dye removal by biosorption using cross-linked chitosan-based hydrogels, Environ. Chem. Lett., vol. 17, n.o 4, pp. 1645-1666, dic. 2019, doi: 10.1007/s10311-019-00903-y.
-
Y. A. R. Lebron, V. R. Moreira, and L. V. S. Santos, Studies on dye biosorption enhancement by chemically modified Fucus vesiculosus, Spirulina maxima and Chlorella pyrenoidosa algae, J. Clean. Prod., vol. 240, p. 118197, dic. 2019, doi: 10.1016/j.jclepro.2019.118197.
-
M. L. Pinzón-Bedoya and L. E. V. Villamizar, Modelamiento de la cinética de bioadsorción de Cr (III) usando cáscara de naranja, Dyna, vol. 76, n.o 160, pp. 95-106, 2009.
-
P. Aslani and R. A. Kennedy, Studies on diffusion in alginate gels.
-
Effect of cross-linking with calcium or zinc ions on diffusion of acetaminophen, J. Controlled Release, vol. 42, n.o 1, pp. 75-82, oct. 1996, doi: 10.1016/0168-3659(96)01369-7.
-
-
Controlled release of albumin from chitosanalginate microcapsules – Polk – 1994 – Journal of Pharmaceutica Sciences – Wiley Online Library. https://onlinelibrary.wiley.com/doi/abs/10.1002/jps.2600830213 (accesed dic. 22, 2020).
-
A. Kikuchi, M. Kawabuchi, M. Sugihara, Y. Sakurai, and T. Okano, Pulsed dextran release from calcium-alginate gel beads, J. Controlled Release, vol. 47, n.o 1, pp. 21-29, jul. 1997, doi: 10.1016/S0168-3659(96)01612-4.
-
T. L. Bowersock et al., Oral vaccination of animals with antigens encapsulated in alginate microspheres, Vaccine, vol. 17, n.o 13, pp. 1804-1811, ene. 1999, doi: 10.1016/S0264-410X(98)00437-X.
-
J.-H. Cui, J.-S. Goh, S.-Y. Park, P.-H. Kim, and B.-J. Lee, Preparation and Physical Characterization of Alginate Microparticles Using Air Atomization Method, Drug Dev. Ind. Pharm., vol. 27, n.o 4, pp. 309-319, ene. 2001, doi: 10.1081/DDC- 100103730.
-
M. L. González-Rodrg uez, M. A. Holgado, C. Sánchez-Lafuente, A.
M. Rabasco, and A. Fini, Alginate/chitosan particulate systems for
sodium diclofenac release, Int. J. Pharm., vol. 232, n.o 1, pp. 225- 234, ene. 2002, doi: 10.1016/S0378-5173(01)00915-2.
-
A. Halder, S. Maiti, and B. Sa, Entrapment efficiency and release characteristics of polyethyleneimine-treated or -untreated calcium alginate beads loaded with propranololresin complex, Int. J. Pharm., vol. 302, n.o 1, pp. 84-94, sep. 2005, doi: 10.1016/j.ijpharm.2005.06.020.
-
P. Geetha, M. S. Latha, and M. Koshy, Biosorption of malachite green dye from aqueous solution by calcium alginate nanoparticles: Equilibrium study, J. Mol. Liq., vol. 212, pp. 723-730, dic. 2015, doi: 10.1016/j.molliq.2015.10.035.
-
V. Rocher, J.-M. Siaugue, V. Cabuil, and A. Bee, Removal of organic dyes by magnetic alginate beads, Water Res., vol. 42, n.o 4, pp. 1290-1298, feb. 2008, doi: 10.1016/j.watres.2007.09.024.
-
S. Mandal, S. S. Kumar, B. Krishnamoorthy, and S. K. Basu, Development and evaluation of calcium alginate beads prepared by sequential and simultaneous methods, Braz. J. Pharm. Sci., vol. 46, n.o 4, pp. 785-793, dic. 2010, doi: 10.1590/S1984- 82502010000400021.
-
E. Torres, Y. N. Mata, M. L. Blázquez, J. A. Muñoz, F. González, and A. Ballester, Gold and Silver Uptake and Nanoprecipitation on Calcium Alginate Beads, Langmuir, vol. 21, n.o 17, pp. 7951-7958, ago. 2005, doi: 10.1021/la046852k.
-
W.-T. Cong, S.-Y. Hwang, L.-T. Jin, and J.-K. Choi, Detection of Proteins on Blots Using Direct Blue 71, en The Protein Protocols Handbook, J. M. Walker, Ed. Totowa, NJ: Humana Press, 2009, pp. 729-735.
-
Y. S. Ho and G. McKay, Pseudo-second order model for sorption processes, Process Biochem., vol. 34, n.o 5, pp. 451-465, jul. 1999, doi: 10.1016/S0032-9592(98)00112-5.
-
M. A. López-Leal, R. Cortés-MartÃnez, R. Alfaro-Cuevas- Villanueva, H. E. MartÃnez-Flores, and C. D. J. Cortés-Penagos, Arsenate biosorption by iron-modified pine sawdust in batch systems: kinetics and equilibrium studies, BioResources, vol. 7, n.o 2, Art. n.o 2, feb. 2012.
-
J. R. Baseri, P. N. Palanisamy, and P. Sivakumar, Comparative Studies of the Adsorption of Direct Dye on Activated Carbon and Conducting Polymer Composite, E-Journal of Chemistry, 2012. https://www.hindawi.com/journals/jchem/2012/603196/ (accedido dic. 23, 2020).
-
M. A. Kamaruddin, M. S. Yusoff, H. A. Aziz, and R. Alrozi, Preparation of rice husk carbon-clinoptilolite composite adsorbent for color removal from real textile wastewater: Effects of operating conditions, en 2013 IEEE Business Engineering and Industrial Applications Colloquium (BEIAC), abr. 2013, pp. 494-499, doi: 10.1109/BEIAC.2013.6560176.
-
Y. Bulut, N. Gözübenli, and H. Aydn, Equilibrium and kinetics studies for adsorption of direct blue 71 from aqueous solution by wheat shells, J. Hazard. Mater., vol. 144, n.o 1, pp. 300-306, jun. 2007, doi: 10.1016/j.jhazmat.2006.10.027.
-
A. Leudjo Taka, E. Fosso-Kankeu, K. Pillay, and X. Yangkou Mbianda, Metal nanoparticles decorated phosphorylated carbon nanotube/cyclodextrin nanosponge for trichloroethylene and Congo red dye adsorption from wastewater, J. Environ. Chem. Eng., vol. 8, n.o 3, p. 103602, jun. 2020, doi: 10.1016/j.jece.2019.103602.
-
D. Talbot, S. Abramson, N. Griffete, and A. Bée, pH-sensitive magnetic alginate/-Fe2O3 nanoparticles for adsorption/desorption of a cationic dye from water, J. Water Process Eng., vol. 25, pp. 301-308, oct. 2018, doi: 10.1016/j.jwpe.2018.08.013.
-
E. Fourest and B. Volesky, Contribution of Sulfonate Groups and Alginate to Heavy Metal Biosorption by the Dry Biomass of Sargassum fluitans, Environ. Sci. Technol., vol. 30, n.o 1, pp. 277- 282, dic. 1996, doi: 10.1021/es950315s.
-
M. N. V. Ravi Kumar, A review of chitin and chitosan applications, React. Funct. Polym., vol. 46, n.o 1, pp. 1-27, nov. 2000, doi: 10.1016/S1381-5148(00)00038-9.
-
M. Ruiz, C. Tobalina, H. Demey-Cedeño, J. A. Barron-Zambrano, and A. M. Sastre, Sorption of boron on calcium alginate gel beads, React. Funct. Polym., vol. 73, n.o 4, pp. 653-657, abr. 2013, doi: 10.1016/j.reactfunctpolym.2013.01.014.
-
M. Sarkar, A. Banerjee, and P. P. Pramanick, Kinetics and Mechanism of Fluoride Removal Using Laterite, Ind. Eng. Chem. Res., vol. 45, n.o 17, Art. n.o 17, ago. 2006, doi: 10.1021/ie060016j.
-
B. P. Bering, M. M. Dubinin, and V. V. Serpinsky, On thermodynamics of adsorption in micropores, J. Colloid Interface Sci., vol. 38, n.o 1, Art. n.o 1, ene. 1972, doi: 10.1016/0021- 9797(72)90233-0.
-
M. E. Argun, S. Dursun, C. Ozdemir, and M. Karatas, Heavy metal adsorption by modified oak sawdust: Thermodynamics and kinetics, J. Hazard. Mater., vol. 141, n.o 1, Art. n.o 1, mar. 2007, doi: 10.1016/j.jhazmat.2006.06.095.
-
G. Atun and A. Kilislioglu, Adsorption behavior of cesium on montmorillonite-type clay in the presence of potassium ions, J. Radioanal. Nucl. Chem., vol. 258, n.o 3, Art. n.o 3, dic. 2003, doi: 10.1023/B:JRNC.0000011757.59069.ba.
-
Y. Song, Y. Liu, S. Chen, H. Qin, and H. Xu, Carmine Adsorption from Aqueous Solution by Crosslinked Peanut Husk, Iran. J. Chem. Chem. Eng. IJCCE, vol. 33, n.o 4, pp. 69-77, dic. 2014.
-
N. Khalid, S. Rahman, and S. Ahmad, Potential of Sawdust for the Decontamination of Lead from Aqueous Media, Sep. Sci. Technol., vol. 40, n.o 12, Art. n.o 12, sep. 2005, doi: 10.1080/01496390500267467.