
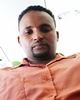
- Open Access
- Authors : Lemma Teklu Kumsa
- Paper ID : IJERTV11IS010050
- Volume & Issue : Volume 11, Issue 01 (January 2022)
- Published (First Online): 26-01-2022
- ISSN (Online) : 2278-0181
- Publisher Name : IJERT
- License:
This work is licensed under a Creative Commons Attribution 4.0 International License
Effect of Deficit Furrow Irrigation Methods on Yield and Water Productivity of Tomato (Solanum Lycopersicum L.) at Bako Tibe District of Western Oromia, Ethiopia
Lemma Teklu Kumsa
Oromia Agricultural Research Institute, Agricultural Engineering Research Process Fitche Agricultural Research Center, P. O. Box 09, Fitche, North Shoa, Ethiopia,
Abstract:- Improving water use efficiency is one of the important strategies for addressing future water scarcity, which is driven particularly by increasing human population. Enhancing agricultural water productivity is a critical response as it is by far the main consumer of global fresh water. A field experiment was conducted at Bako Tibe district of Western Oromia during the off-rain season of 2017/18 and 2018/19 cropping calendar to investigate the effect of deficit irrigation under Alternative furrow irrigation (AFI), Conventional furrow irrigation (CFI) and fixed furrow irrigation (FFI) methods with three water application depth of 100% ETc, 75% ETc and 50% ETc on yield and water productivity (WP) of tomato (Galelia variety) for better agricultural production and environmental sustainability. The experiment was carried out as a two factorial combination of three irrigation systems and three water application levels in randomized complete block design with nine treatments and three replications. Irrigation depth was monitored using a Parshall flume of an opening diameter 3 inch with discharge of 4.239 l/s at a head of 9cm. Results were compared in terms of yield and WP at (P<0.05). There were highly significant differences between the mean results of both irrigation systems and water application levels on yields and WP. The interactions effects between irrigation systems and water application depth were highly significant on WP, while the rest were none significant for both yield and WP. The maximum yield and WP of 27.85ton/ha and 13kg/m3 were obtained under AFI at 100% ETc, while the minimum of 22.01ton/ha and 9.8kg/m3 were obtained from FFI systems 100% ETc, respectively. Thus, AFI with 100% Etc application depth is found the best irrigation system for tomato production and recommended to be used for the communities of the study area and similar agro ecologies.
Key words: Deficit Irrigation, Irrigation systems, Tomato yield and Water productivity
INTRODUCTION
Water is one of the largest renewable natural resources but fresh water is expected to emerge as a key constraint to future agricultural growth. The changing situation comes partly from increasing demands such as population, industry and domestic requirements partly from consequence of climate change (Ashebir et al., 2018). The major concern on future planetary fresh water resources are the effects of climate change on changing sea temperature and levels, drought and flood events, as well as change in water quality, and general ecosystem vulnerabilities (Xu et al.,2015). The unpredictability of climatic events is of key concern to farmers in all countries, particularly in Africa.
According to Awulachew et al., (2010), estimate the irrigation potential of Ethiopia was 5.3 million hectares. In terms of utilization, only about 12% (857,933 ha) has been irrigated by 2015 (FDRE, 2016). So, it is prudent to make efficient use of water and bring more area under irrigation through available resources. This can be achieved by introducing advanced methods of irrigation and improved water management practices (Ashebir et al., 2018).
Deficit irrigation is one way of maximizing water use efficiency for higher yields per unit of irrigation water used in agriculture (Geerts and Raes, 2009; Nagaz et al., 2012). In deficit irrigation application, the crop is exposed to a certain level of water stress either during a particular growth period or throughout the whole growing season, without significant reductions in yields (FAO, 2011). The expectation is that the yield reduction by introducing controlled water stress will be insignificant compared with the benefits gained through diverting the saved water to irrigated additional cropped area (Gijon et al., 2007; Ali et al.,2007).
Tomato (Solanum lycopersicon L.) is one of the most important irrigated vegetable crops and is one of the most demanding in terms of water use (Peet, 2005). The application of various deficit irrigation strategies to this crop may significantly led to save irrigation water (Costa et al., 2007).
In the study area, over application of irrigation water without determining the crop water requirement were identified as the major problem of a crop failure. Under such existing condition, practicing of deficit irrigation and water saving methods of furrow irrigation systems could help to increase agricultural production by expanding irrigable land with the given limited amount of water. Therefore, the objectives of the study were to evaluate the effect of deficit irrigation under furrow irrigation methods on yield and water productivity of tomato for better agricultural production and environmental sustainability.
MATERIALS AND METHODS
Study Area Description
The experiment was conducted at Bako Tibe district of Western Oromia. It is located at about 236 km away from Addis Ababa to Nekemt at East direction from Bako town at an altitude of 1676 masl (Figure 1). The experimental site was characterized by clay loam soil type, dry and wet season with over nine moths wet period. The majority of the rainfall occurs from May to September. The area receives an annual rainfall ranges from 1000mm-2100mm and an average annual temperature of 19oC.
Map of study area
Experimental Design and Procedure
The experiment was implemented in two factorial combinations namely, three irrigation systems and three irrigation water application levels (Table1). The treatments combinations were arranged as completely randomized blocks design with three time replications. The depth of water applied to the field was measured by Parshall Flume of 3inch throat diameter. The effective head of 9cm was calibrated and hence the resulting discharge was 4.239 l/s. The plot size was 4 m × 10m with 1m and 2m space between plots and blocks respectively. Each plot contained four ridges and four furrows. Each bed had 1m width and 10m length. The trapezoidal shape furrow was prepared with an average depth of 40 cm and width of 30 cm and 20cm at the top and bottom, respectively.
Table.1. Treatments used for the experiment
Irrigation systems |
Water application levels |
|||
100% ETc |
75% ETc |
50% ETc |
||
AFI |
AFI100% ETc |
AFI 75% ETc |
AFI 50% ETc |
|
FFI |
FFI100% ETc |
FFI 75% ETc |
FFI 50% ETc |
|
CFI |
CFI100% ETc |
CFI 75% ETc |
CFI 50% ETc |
Where: AFI100% ETc, FFI100% ETc and CFI100% ETc were alternative, fixed and conventional furrow irrigation with full irrigation respectively, AFI 85% ETc, FFI 85% ETc and CFI 85% ETc were 85% of the full irrigation (15% deficit), AFI 70% ETc, FFI 70% ETc and CFI 70% ETc were 70% of full irrigation (30% deficit) and AFI 50% ETc, FFI 50% ETc and CFI 50% ETc were 50% of full irrigation (50% deficit).
Soil Sample Collection and Analysis Methods
Depending on the greatest root depth concentration which is 30cm for transplanted tomatoes, the disturbed and undisturbed composite soil sample before planting were collected at a depth of 0-20 and 20-40 cm. Different soil physical properties such as bulk density, texture, infiltration, field capacity and permanent wilting point were done by core sampler method, pipette method, double ring infiltrometer, pressure plate apparatus by applying a suction of 1/3 and 15 bars to a saturated soil sample, respectively.
Determination of Crop Water and Irrigation Requirement
Crop water requirement of tomato for the growing season was determined from the reference evapotranspiration and crop coefficient using Equation (1). Soil (Table 2) and Meteorological datas (Table 3) were used to determine reference crop evapotranspiration (ETo). Farmers experience was used to determine the numbers of days of each growing stages to estimate reliable Kc for the respective growing stages. A higher value of application efficiency (60%) was adopted, because water was applied more accurately and no runoff. Irrigation scheduling of the crop was computed using FAO CROPWAT program (Allen et al., 1998).
ETc = Kc × ETo (1)
Where: ETc = crop evapotranspiration (mm/day), Kc = crop coefficient (dimensionless), and
ETo = reference crop evapotranspiration (mm/day).
Table 2. Input soil data for CROPWAT model
Depth of sampling (cm) |
FC (%) by vol. |
PWP (%) by vol. |
Bd (g/cm3) |
Sand % |
Silt % |
Clay % |
Textural class |
Infiltration rate (mm/hr) |
0-20 |
32.98 |
24.33 |
1.31 |
37 |
23 |
40 |
Clay |
|
20-40 |
36.33 |
27.3 |
1.35 |
37 |
27 |
36 |
Clay loam |
7.2 |
Average |
34.66 |
25.82 |
1.33 |
37 |
25 |
38 |
Clay loam |
Where: FC, PWP and Bd were field capacity, permanent wilting point and bulk density respectively.
Table.3. Climate data and ETo value of the study area
Months |
Temp. max. (0C) |
Temp. min. (0C) |
Humidity (%) |
Wind speed (km/hr) |
Sun shine (hr) |
Solar radiation (MJ/M2/day) |
Rain fall (m) |
ETo (mm/day) |
January |
30.0 |
11.4 |
53.0 |
2.9 |
8.3 |
523.7 |
11.4 |
3.19 |
February |
31.0 |
12.6 |
49.7 |
3.3 |
8.3 |
537.5 |
16.2 |
3.54 |
March |
31.4 |
14.2 |
50.5 |
3.6 |
7.5 |
529.7 |
47.2 |
3.76 |
April |
31.0 |
14.6 |
53.0 |
3.6 |
7.4 |
516.9 |
63.7 |
3.88 |
May |
28.9 |
14.8 |
61.3 |
3.6 |
6.8 |
506.6 |
144.7 |
3.69 |
June |
26.1 |
14.6 |
69.0 |
3.0 |
5.5 |
469.3 |
223.8 |
3.26 |
July |
24.1 |
14.9 |
74.1 |
2.4 |
3.5 |
402.1 |
251.7 |
2.76 |
August |
24.2 |
14.7 |
74.7 |
2.1 |
3.4 |
419.6 |
233.0 |
2.77 |
September |
25.3 |
14.4 |
72.6 |
2.0 |
4.8 |
464.0 |
145.6 |
3.07 |
October |
27.6 |
13.1 |
64.4 |
2.0 |
7.7 |
517.8 |
70.2 |
3.53 |
November |
28.6 |
11.7 |
59.8 |
2.2 |
8.5 |
530.1 |
26.9 |
3.34 |
December |
29.3 |
10.9 |
55.9 |
2.4 |
8.7 |
533.4 |
12.6 |
3.15 |
Source: Bako Agricultural Research Center station, 1961-2017 G.C.
Crop Agronomy and Management
Improved tomato Galilea variety having a total growing period of 90 days after transplanting was grown on nursery for 21 days and transplanted on experimental plots on 15th January, 2018. The crop variety was selected for its good adaptability, disease resistant and most usable in the study area.
Tomato seedlings were transplanted to the experimental plots based on the recommended space of 60 cm between plants and 100 cm row spacing. Recommended fertilizer of 200 kg/ha DAP at the time of transplanting and 150 kg/ha Urea twice, half at the time of transplanting while, half at 21 days after transplanting were equally and uniformly applied to each treatments. The crop was cultivated and weeded four times during the growing season. It was transplanted on four ridges of each plot and for further analysis; the yield were harvested from the two central ridges to avoid boarder effects.
Depth and discharge measurement
The total amount of water requirement for the crop was diverted to the furrow with calibrated parshall flume having appropriate opening diameter of three inch (3") with a length of 2 m and its appropriate head ranges from 3-33cm. It was calculated as suggested by Michael, (2008):
Q= 0.1771p.5 (2)
Where: Q = discharge from parshall flume, (l/s)
h = effective head of Parshall flume causing flow, (cm)
The time required to deliver the desired depth of water into each furrow was calculated using the equation recommended by Israelsen (1980).
= ××
360×
Where: d= gross depth of water applied, (cm) t= application time, (hr)
l= furrow length in, (m) w= furrow spacing in, (m)
q= flow rate (discharge), (l/s)
Yield assessments (y)
(3)
Yield obtained in ton per ha = y × 104 (4)
Where:
y = yield obtained per square meter
Water Productivity (WP)
Water productivity was determined by dividing grain yield by total applied irrigation water and is expressed as follows (Ali et al., 2007):
WP = GY/Wa (5)
Where:
GY = grain yield (kg/ha)
Wa = total irrigation water applied (m3/ha)
Statistical Analysis
The collected data were analyzed using GenStat18th edition, ANOVA and the mean difference was estimated using the least significance difference (LSD) comparisons.
RESULTS AND DISCUSSIONS
Crop water requirements and irrigation scheduling of Tomato
Crop water requirements were calculated by multiplying the reference evapotranspiration values with the tomato crop coefficient (Allen et al., 1998). The seasonal irrigation water requirement of tomato was found to be 2992m3. This amount of water was needed for 100% ETc with CFI (full irrigation) level treatment, while 75% and 50% of 100% ETc with CFI were 2244 and 1496 m3, respectively. The total irrigation water applied to AFI and FFI systems with 100%, 75% and 50% were 1496, 1122 and 748m3 accordingly. The depth of irrigation water required at each irrigation interval and number of irrigation events were described on Table 4. The result indicates that, the maximum depth of water was applied during mid of March which is he mid development stage of tomato. Sahasrabudhe (1996) suggested that, this is the time when the crop needs high amount of water. Maximization of crop yield and quality can be achieved through meeting crop water requirement during this critical period, given all other factors are met. Probably the high tomato water requirement during this stage of development can be accounted for development of flowers and fruit which is high energy demanding and peak physiological phase for the crop growth (Sahasrabudhe, 1996). By sufficiently supplying water to the plant, during such critical time and ensuring its uptake, it is possible to improve crop water productivity.
Table 4. Irrigation interval and depth of applied water to each treatment
Irrigation systems |
Water application levels |
Irrigation period and depth of applied water (mm) |
|||||||||
15th January |
21th January |
28th January |
04th February |
12th February |
20th February |
01st March |
10th March |
21th March |
31th March |
||
100% ETc |
572 |
656 |
668 |
880 |
1192 |
1396 |
1604 |
1652 |
1716 |
1632 |
|
75% ETc |
429 |
492 |
501 |
660 |
894 |
1047 |
1203 |
1239 |
1287 |
1224 |
|
CFI |
50% ETc |
286 |
328 |
334 |
440 |
596 |
698 |
802 |
826 |
858 |
816 |
100% ETc |
286 |
328 |
334 |
440 |
596 |
698 |
802 |
826 |
858 |
816 |
|
AFI |
75% ETc |
214.5 |
246 |
250.5 |
330 |
440 |
523.5 |
601.5 |
619.5 |
643.5 |
612 |
50% ETc |
143 |
164 |
167 |
220 |
298 |
349 |
401 |
413 |
429 |
408 |
|
100% ETc |
286 |
328 |
334 |
440 |
596 |
698 |
802 |
826 |
858 |
816 |
|
FFI |
75% ETc |
214.5 |
246 |
250.5 |
330 |
440 |
523.5 |
601.5 |
619.5 |
643.5 |
612 |
50% ETc |
143 |
164 |
167 |
220 |
298 |
349 |
401 |
413 |
429 |
408 |
Where: CFI, AFI and FFI are conventional furrow irrigation, alternative furrow irrigation and fixed furrow irrigation, respectively.
Effect of Irrigation Systems and Water Application Levels on Tomato Yield
The analysis of marketable tomato yield showed that, both irrigation systems and water application levels were highly significant at P<0.5 on yields (Table 5). But, the interaction effect between irrigation systems and water application levels, irrigation systems and year, water application levels and year, water application levels and year and irrigation systems were not significant.
Table 5. Analysis of variance of yield (Qt/ha) and water productivity (Kg/m3)
Source of variation |
df |
MS (yield) |
MS (WP) |
Irrigation systems (IS) |
2 |
16211.4** |
47.1992** |
Water application levels (WAL) |
2 |
36580.4** |
67.0226** |
Year |
1 |
100.3ns |
4.9889 |
Irrigation systems * Water application levels (WAL) |
4 |
821.3ns |
6.0413** |
Irrigation systems *year |
2 |
4.8ns |
0.4133 |
Water application levels *year |
2 |
20ns |
0.3813 |
Irrigation systems * Water application levels (WAL) *year |
4 |
6.1ns |
0.9323 |
Where: df- degree of freedom, MS- mean square, WP- water productivity
According to table 6, the yield obtained from the three irrigation systems and water application levels were significantly different from each other. The maximum marketable yield of 278.5 Qt/ha and 299.8Qt/ha were obtained from alternative furrow irrigation (AFI) systems and 100% ETc, while the minimum marketable yield of 220.1Qt/ha and 209.8Qt/ha were observed from fixed furrow irrigation (FFI) systems and 50% ETc water application levels, respectively. The yield obtained in AFI system was better than CFI system under 50% reductions in applied irrigation water. This is probably because of the better application efficiency and physiological response associated with AFI (Zhang et al., 2000) and less evapotranspiration associated with AFI (Stone et al., 1979). The significant yield difference among the water application levels were consistent with the report of continuous water stress during the period of fruit set and fruit development can results significantly reduced fresh fruit yield and blossom-end rot (Sahasrabudhe, 1996).
Table 6. Effect of irrigation systems and water application levels on yield
Irrigation systems |
Yield (Qt/ha) |
Alternative furrow irrigation (AFI) |
278.5a |
Conventional furrow irrigation (CFI) |
261.2b |
Fixed furrow irrigation (FFI) |
220.1c |
Water Application Levels |
|
100% ETc |
299.8a |
75%ETc |
250.2b |
50% ETc |
209.8c |
LSD0.5 |
12.08 |
SE |
4.2 |
CV (%) |
7 |
Effect of Irrigation Systems and Water Application Levels on Water Productivity
As depicted from the analysis of variance (Table 5), irrigation systems and water application levels and their interaction were highly significant at (P<0.5) on water productivity. However, there were no significant differences between the interactions of irrigation systems and year, water application levels and year, water application levels, year and irrigation systems.
The water productivity of the three irrigation systems and water application levels were significantly different from each other (Table 7). The maximum and minimum of 13kg/m3 and 9.8kg/m3 water productivity were obtained from AFI and FFI, respectively. This is because of the higher rate of lateral flow towards the dry soil part in AFI system under low water application levels rather than down ward flows. This is consistent with the significant improvements in water productivity that have been associated with AFI and FFI (Feyen and Zerihun 1999; Kassa, 2001; Woldesanbet, 2005).
The maximum and minimum water productivity of 13.7kg/m3 and 9.9kg/m3 were observed under low and high water application levels of 50% ETc and 100% ETc, respectively. Both irrigation systems and water application levels were better in water productivity under low irrigation water application depth. This is because of the difference in percentage of water actually converted to evapotranspiration out of the total amount applied.
Table 7. Effect of irrigation systems and water application levels on water productivity
Irrigation systems |
Water productivity (kg/m3) |
Alternative furrow irrigation (AFI) |
13a |
Conventional furrow irrigation (CFI) |
12b |
Fixed furrow irrigation (FFI) |
9.8c |
Water Application Levels |
|
100% ETc |
9.9c |
75%ETc |
11.2b |
50% ETc |
13.7a |
LSD0.5 |
0.66 |
SE |
0.23 |
CV (%) |
8.4 |
Interaction effects between irrigation systems and water application levels were significantly different on water productivity (Table 5). According to table 8, water productivity was highly influenced under FFI systems at 100% water application levels. This may be due to, the application of irrigation water to the fixed furrow of the plot throughout the growing season of the crop.
Table 8. Interaction effects of irrigation systems and water application levels on water productivity (Kg/m3)
Irrigation systems |
Water application levels |
Mean |
||
50% ETc |
75% ETc |
100% ETc |
||
AFI |
16.2a |
12.5c |
10.2de |
12.96 |
CFI |
14b |
11.3d |
10.7de |
12.00 |
FFI |
11d |
9.6ef |
8.8f |
9.80 |
Mean |
13.73 |
11.13 |
9.9 |
11.59 |
LSD0.05 |
1.15 |
|||
CV (%) |
8.4 |
Partial Budget Analysis
From the economic analysis results (Table 9), AFI with 100% ETc is better in marginal rate of return and more advantageous to irrigators. Because, for 1birr/ha on average invested cost the farmers may get 1birr/ha plus an extra of 90.87 birr/ha net benefits. In addition to this, the net benefits of 22940 birr/ha is obtained while changing from CFI system with 100% ETc to AFI system with 100% ETc under 50% reduction in volume of applied irrigation water. This means 2992 m3 volume of water is needed to irrigate 1 hectare area in CFI system which is enough to irrigate 2 hectare area of land in AFI system. So, when the area to be irrigated becomes double in AFI system using the saved volume of water, the yield and income obtained also becomes double.
Table.9.Irrigation water & time of irrigation saved and cost- benefit analysis of the interaction effects of irrigation systems and water application levels
Treatments |
Time saved (hr/ha) |
Water saved (m3/ha) |
sum of cost that varies (birr/ha) |
Total yield (ton/ha) |
Adjusted yield (10%) (ton/ha) |
Total revenue (birr/ha) |
Net benefits (birr/ha) |
DA |
MRR= TR*100% MC |
AF with 50% ETc |
183.11'35'' |
748.5 |
31538 |
144.65 |
130.19 |
139150 |
107612 |
D |
– |
FF with 50% ETc |
183.11'35'' |
748.5 |
31540 |
107.35 |
96.62 |
130750 |
99210 |
D |
– |
AF with 75% ETc |
165.19'43'' |
149.7 |
44333.2 |
169.76 |
152.78 |
181725 |
137391.8 |
298.39 |
|
FF with 75%ETc |
165.19'43'' |
149.7 |
44563.1 |
117.35 |
105.62 |
158300 |
113736.9 |
D |
– |
AF with 100% ETc |
57.38'51'' |
1497 |
45896.1 |
188.97 |
170.07 |
280769 |
234872.9 |
9087.47 |
|
FF with 100% ETc |
57.38'51'' |
1497 |
46646 |
160.26 |
144.23 |
206550 |
159904 |
D |
– |
CF with 50% ETc |
126.37'50'' |
1497 |
48569.1 |
135.73 |
122.16 |
141500 |
92930.9 |
D |
– |
CF with 75% ETc |
95.18'22'' |
299.2 |
67366.48 |
151.98 |
136.78 |
177075 |
109708.52 |
89.26 |
|
CF with 100% ETc |
0 |
0 |
71492.1 |
192.5 |
173.25 |
283425 |
211932.9 |
2477.79 |
Where: D, DA, MRR, MR and MC were dominance, dominance analysis, marginal rate of return, change in marginal revenue and change in marginal cost, respectively.
SUMMARY AND CONCLUSSIONS
Tomato needs high amount of irrigation water during the flowering and fruit setting stage and continuous stress significantly reduces fresh fruit yield, especially in FFI system as half of the root stay dry throughout the growth period.
The irrigation water used in AFI system is 50% of CFI system, but the tomato yield obtained was similar. Because in CFI system four furrows irrigated at the same time while in AFI and FFI only two furrows out of four furrows. This may improves working conditions as technology allows irrigator moving on the dry furrows. It is possible to double the irrigated command area by using the saved irrigation water.
Over application and high frequency irrigation was a known constraint in the study area and giving training and advisory service for communities on how to use crop water requirement based irrigation system will be a better solution.
Alternative furrow irrigation system at 100% ETc is the best technology among the tested technologies to be recommended for the communities of the study area, because of its high net benefits, water productivity and yield performance, in addition to time and irrigation water saving.
REFERENCES:
-
Ali, M. H., Hoque, M.R. and Hassan, A. K. 2007. Effect of deficit irrigation on yield, water productivity and economic return of wheat. Agricultural Water Management. Vol. 92; 2007. P151-161.
-
Allen, R.G., Pereira L.S., Raes D. and Smith M. 1998. Crop evapotranspiration: guidelines for computing crop water requirements. Irrigation and Drainage paper No 56. FAO, Rome.
-
Ashebir Haile, Solomon Gezi and Gebeyehu Tegenu. 2018. Integrated effect of mulching and furrow methods on onion yield and water productivity in central highlands of Ethiopia. Proceeding of the 18th International Symposium on Sustainable Water Resource Development. Arba Minch University workshop; 2018, June 08-09. Ethiopia Journal of Water Science and Technology
-
Awulachew S.B., Merrey, D., Van Koopen, B. and Kamara, A. 2010. Roles, Constraints and Opportunities of Small Scale Irrigation and Water Harvesting in Ethiopian Agricultural Development: Assessment of Existing Situation. ILRI workshop; 2010 March 14-16; Addis Ababa, Ethiopia: International Water Management Institute(IWMI).
-
Costa, J. M., Ortuno, M. F. and M. M. Chaves. 2007. Deficit irrigation as a strategy to save water: physiology and potential application to horticulture.
J. Integr. Plant Biol. 49: 14211434.
-
FAO (Food and Agricultural Organization). 2011. The state of world's land and water resources for food and agriculture. Managing systems at risk. FAO, Rome, Italy.
-
FDRE (Federal Democratic Republic of Ethiopia). 2016. Growth and transformation plan II (GTPII). Volume 1, Addis Ababa.
-
Feyen J. and D. Zerihun. 1999. Assessment of the performance of border and furrow irrigation systems and the relationship between performance indicators and system variables. Agricultural Water Management 40: 353-362.
-
Geerts, S. and Raes, D. 2009. Deficiti irrigation as an on-farm strategy to maximize crop water productivity in dry areas. Agricultural Water Management. 96, p12-22.
-
Gijon, M.C., Guerrero , J., Coureiro, J.F., and Moriana, A. 2007. Deficit irrigation without reducing yield or nut splitting in pistachio. Agricultural Water Management. 68: 1-17.
-
Israelsen, O.W., and Hansen, V.E. 1980. Irrigation Principles and Practices. Jonsen Wiley and Sons, Inc. New York, London.
-
Kassa Teddla. 2001. Performance evaluation of surface irrigation methods. Alemaya University.94 p.
-
Michael, A.M. 2008. Irrigation Theory and Practices. 2nd edn. Jupiter Offset Printers, Dehli-32.
-
Nagaz, K., Masmoud, M.M. and Mechlia, N. 2012. Effects of deficit drip-irrigation scheduling regimes with saline water on pepper yield, water productivity and soil salinity under arid conditions of Tunisia. Journal of Agriculture and Environment for International Development-JAEID 106(2) 85-103.
-
Peet, M. M. 2005. Irrigation and fertilization. In: Heuvelink E, ed. Tomatoes, Crop Production Science in Horticulture. CABI Publishing, UK. pp. 171- 198.
-
Xu, J.,Li, C., Liu, H., Zhou, P., Tao, Z., Wang, P., Meng, Q. and Zhao, M. 2015. The effect of plastic film mulching on maize growth and water use in dry and rainy years in Northeast China. PLoS ONE 10(5).