
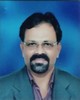
- Open Access
- Authors : W. S. Barde , S. S. Arsad
- Paper ID : IJERTV11IS070067
- Volume & Issue : Volume 11, Issue 07 (July 2022)
- Published (First Online): 25-07-2022
- ISSN (Online) : 2278-0181
- Publisher Name : IJERT
- License:
This work is licensed under a Creative Commons Attribution 4.0 International License
Effect of Concentration Of FeCl3 on Ionic Conductivity of Polypyrrole-Poly (Vinyl Acetate) Composite
W.S.Barde
Department of Physics
Shri Shivaji Science College, Amravati MS, India
S.S.Arsad
Department of Physics
Shri Shivaji Science College, Amravati MS, India
Abstract Electrically conducting polypyrrole (PPy) thin films were obtained by chemical polymerization method. Pyrrole oxidized at different oxidizing strength [0.1 M 1 M] by Ferric chloride [FeCl3]. The transference number study, by dc polarization technique, indicates the ionic conduction in the PPy film. The ionic behavior of the PPy film varies with different oxidizing strength. PPy film synthesized by low oxidizing strength (0.1 M and 0.2 M) shows mixed electrical conductivity with transference number 0.49 and 0.55 respectively, while the films synthesized by higher strength of FeCl3 [ 0.3 M] shows ionic conductivity having transference numbers between 0.8 to
0.99. The temperature dependence of conductivity shows Arrhenius type behavior but two different activation regions are clearly visible. The conducting behavior of the PPy films depends on concentration of oxidizing agent (FeCl3). At lower strength of FeCl3 a small variation in conductivity of the film is observed. The maximum conductivity 7.4 X10-10 S/cm is observed for the PPy film synthesized by 0.5 M of FeCl3, which may be due to more number of charges (counter ions) incorporated into the PPy structure. The tendency of further decrease in conductivity with increase in concentration of FeCl3 may be due to charge association.
Keywords Polypyrrole , ionic conductivity, transference number, activation energy
INTRODUCTION
Electrically conducting polymers such as polyacetylene, polyaniline, polythiophene and polypyrrole have received much attention in the literature of conducting polymers because of their unique properties such as low density and versatility in methods of production, good electrical conductivity and non-metallic temperature dependence of conductivity (Kaiser, 2001a). These polymers have many applications such as in electrochromic devices, corrosion inhibitors, electrochemical actuator, sensors, polymeric batteries, membrane separations, functional electrodes, antistatic coating, and electromagnetic shielding. A second generation conducting polymers has also appeared in transistor, solar cells etc. All these applications are possible because properties of the polymers like electrochemical, optical, chemical, mechanical and electrical can be change by changing monomer and/or dopant concentrations. It has been reported that the properties of conducting polymers depends strongly on processing conditions such as concentration, temperature, stoichiometry, type of monomer, oxidant, dopant and solvent used. Because of the presence of conjugated double bonds and crosslinked covalent bonds polymers are
brittle in nature and infusible which leads to strong inter and intra chain interactions. Attempt has been made to overcome these limitations by designing new monomers and by developing new polymerization mechanism.
Practical application of conducting polymers needed better stability and good processability of the conducting polymers. It has been reported that the properties of the conducting polymers are strongly depends on the processing conditions like concentration and type of oxidant, polymerization temperature, stoichiometry, type of monomer, dopant and solvent. Conducting polymers are britle, infusible and intractable in nature because of the presence of conjugated double bonds and crosslinked covalent bonds. Therefore many attempts have been made to over come such limitations by designing new monomers, by developing new polymerization mechanisms and by blending the polymers.
Composites of the conducting polymers with insulating polymers shows better environmental stability, mechanical strength and procesabilty( Park and Ruckenstein, 1992). In the very beginning of the study of conducting composites, they were made by dispersing conducting particles such as carbon black, graphite fibers and metals in insulating polymer matrices (79, 84). More recently the composites of conducting and insulating polymers have been studied (7, 83, 90, 103). This is because of the versatility of their methods of preparation. Melt mixing, solution casting and fiber spinning are the methods that have been used for production of conducting composites. In these composites the mechanical strength of the host insulating polymer matrix is retained and the conducting polymer imparts the conductivity. Composites have been synthesized mostly by chemical oxidative polymerization method (90, 111,7,82,97) and by electrochemical method of polymerization(103,112,117).
Chemical oxidative polymerization method has advantages of shorter reaction time and large scale production.(Ruckenstein and Park). Also, the number of experimental variables available with electrochemical polymerization is reduced because no electrochemical cell or electrodes are used. Further more it is possible to use chemical deposition to coat other non-conducting material substrate.
The oxidizing agents such as FeCl3 (20,22,42,82,83,88,90,102), CuCl2(20,118), K3Fe(CN)6(58)
and NH4Fe(SO4)2(130) have been used. But FeCl3 is most widely used oxidant in the polymerization of pyrrole. The high oxidation potential of FeCl3 can induce the formation of
highly doped polypyrrole (83,130). But for very high FeCl3 concentration degradation of conjugated bonds is possible because of the formation of covalent carbon-chloride bonds and the aggregated low conductivity material is obtained (107,). In case of polypyrrole the maximum conductivity was reported for oxidant to monomer ratio of 2.33 (101, 102). It has been observed that the electrical properties of the composites have been alterd by changing the strength od oxidizing agent (106,113,107).
In case of insulating substrate sufficient amount of conducting polymer was required to attain the percolation threshold for an insulator-conductor transition (8, 111,97,109,110).
The present paper focuses on the synthesis of PPy films by chemical oxidative polymerization and effect of strength of oxidizing agent on electrical conductivity of polypyrrole with an aim to know the conduction mechanism and structural behavior.
EXPERIMENTAL
The ionic/ electronic transference number (tion/te) was measured using dc polarization technique [15] in which a PPy film is sandwiched between blocking (graphite) and non- blocking (silver) electrodes. A constant dc voltage (1V) is established across the sample and resultant current was monitored as a function of time.
DC conductivity measurement
Electrical conductivity of the sample was measured by two-probe method in the temperature range of 308 383 K .A dc regulated power supply and a pico ammeter with resolution of 1 pA is used for measurement. The composite film was sandwiched between two conducting electrodes and then placed in a muffle furnace. The heating rate of the sample was 2 0 C/min. for the measurement.
RESULTS AND DISCUSSION
Ionic transference number
The current versus time plot for some of the polypyrrrole films are obtained which exhibit typical behavior of ionic charge transport as shown in figs. 1a and 1b.
Sample preparation
PPy is synthesized, at room temperature (303 K) by simple chemical oxidative method. In the first step the solution of ferric chloride (oxidant) and poly (vinyl acetate) in methanol was prepared. Then monomer Pyrrole (E. Merck, Germany) was added to the solutio with stirring. When monomer pyrrole added to the solution of FeCl3 and poly (vinyl acetate), a dark green homogeneous solution was obtained. Conducting polymer composite films were prepared by casting the mixed solution on a plane glass substrate. Evaporation of solvent from the mixed solution results in increasing the oxidation potential, which polymerize the pyrrole rapidly. After complete evaporation of solvent, the thin films were formed which were then washed first with methanol and then with water to remove excess FeCl3. The amount of pyrrole and PVAc used was kept constant for different preparation. In examination of effect of oxidizing strength of FeCl3 on electrical conductivity of PPy-PVAc films, concentration of FeCl3 was changed from 0.1 M to 1
M. for constant pyrrole/PVAc ratio. The films so obtained have the thickness in the range of 60 m 300 m.
DC conductivity measurement
Electrical conductivity of the sample was measured by two-probe method in the temperature range of 308 383 K .A dc regulated power supply and a pico ammeter with resolution of 1 pA is used for measurement. The composite film was sandwiched between two conducting electrodes and then placed in a muffle furnace. The heating rate of the sample was 2 0 C/min. for the measurement.
Ionic transference number
The transference number gives a quantitative information of the extent of ionic and electronics contribution to the total conductivity. The ionic/ electronic transference number can be defined as
tion = Iion/IT te= Ie/IT
Where Iion / Ie are the current contribution due to ions/electrons respectively.
As seen from the fig. 1a or 1b the total current becomes nearly constant at some non-zero value after some time. The final residual current is mainly due to electrons/holes. Hence ionic and electronic transference numbers can be obtained separately from the ratios Iion/IT and Ie/IT respectively. Total ionic transference numbers for all the samples have been calculated from these plots. It was found that for the samples synthesized with 0.3 M to 1 M concentration of FeCl3 transference numbers are lies between the range 0.8 to 0.99 and for the samples synthesized with 0.1M and 0.2 M concentration of FeCl3 transference numbers are 0.49 and 0.55 respectively, which shows mixed type of conduction in films prepared with different strength of FeCl3. The dependence of ionic transference number on FeCl3 concentration is shown in fig.2.
Fig.2 Dependence of ionic transference number on FeCl3 concentration
The ionic transference number (tion) increases with
increase in concentration of FeCl3 and become maximum for
0.5 M of FeCl3. There is a sudden decrease in tion for 0.6 M of FeCl3 and further increases slowly.
The initial increase in tion is due to increase in concentration of ions. Sudden decrease in tion at 0.6 M of FeCl3 may be due to ion association. That is there may be formation of neutral ion pairs. Further slow increase in tion, for concentration of FeCl3 > 0.6 M, may be due to triplet formation of ions
-
Conductivity
The effect of ferric chloride on the properties of the polypyrrole-PVAc films is shown in the table1. The conductivity of the films was found to be increase and then decrease with FeCl3 concentration. The effect of FeCl3 on conductivity of the composite was studied in case of composite films based on polypyrrole and crosslinked poly(methyl acrlate-co-hydroxyethyl acrlate) (106,113,107) and the reported results showed that the conductivity was increased and then decreased with increase in amount of FeCl3. A bell shaped profile of conductivity variation with amount of FeCl3 was reported for polymerization of pyrrole (106). The increase in conductivity of the films indicates the increase in content of polypyrrole in the film. While decrease in the conductivity of the film with further increase in FeCl3 concentration has been attributed to the over oxidation of polypyrrole, which has low bulky density and hence low conductivity. (107,). Same conductivity behavior was observed in the present study of PPy-PVAc films as shown in fig.1
In case of polypyrrole composites grown over PEO-CuCl2 complex blended with polystyrene(PS) (118), phase segregated morphology was observed. The phase segregated morphology indicates the formation of conducting domains and size and shape of the conducting domains depends on the deposition conditions. The effect of phase segregated morphology on the electrical properties of the composites was evidenced in the non-linear current-voltage (I-V) characteristics and the temperature dependence of conductivity. The non-linearity in the I-V characteristics arisen due to the conduction through series of junctions
formed by conducing-insulating-conducting elements in the composite films.
The interpenetrating polymer network composites such as PPy-PMA or PPY-SBA become insoluble after polymerization of pyrrole. This is because of the cross linking of the matrix polymer during polymerization of pyrrole. (111). In the present study it was tested that the PPy-PVAc film is insoluble in methanol and HCl. In general low percolation threshold was reported for inter penetrating network composites.
Fig. 3 shows the conductivity versus temperature plot of polypyrrole thin films. The temperature dependence of conductivity shows Arrhenius behavior but two different activation regions (I and II) are clearly visible. The activation energy is lower in lower temperature region and in higher temperature region activation energy is found to be increased. The crossover from low activation region to high activation region may be due to morphological changes at higher temperatures.
Fig. 3 Variation of lnT vs 1/T for polypyrrole thin films for different concentration of Fecl3
The Arrhenius fitting parameters for polypyrrole films are tabulated in the table 1. As seen from the data it is noted that activation energy for the sample prepared with 0.5 M of FeCl3 solution in region I and II are 0.233 eV and 1.266 eV respectively. A dependence of activation energy with concentration of FeCl3 in region I and II is same (fig. 4). The maximum d. c. electrical conductivity 7.4 X10-10 S/cm at 308 K is observed for the sample prepared with 0.5 M of FeCl3.
Fig. 4 Dependence of activation energy on concentration of Fecl3
The electrical conductivity of the films depends on the initial oxidation potential of the solution. If the oxidation potential of the solution is too low then because of the low rate of polymerization, pyrrole may also evaporates together with solvents during polymerization. On the other hand, if the oxidation potential is too high then rate of polymerization is high. Therefore, phase separation has already progressed before casting the solution. Thus films, for low and high values of concentration of FeCl3, show poor conductivity.
In the present polymerization conditions PVAc may under go cross-link polymerizations via PVA. The alcoholysis reaction that PVAc changed to PVA under excess of methanol is shown. The proton produce during the polymerization of pyrrole may acts as a catalytic function in the alcoholysis reaction and cross-linking of PVAc. Another point that the PVAc modified to cross-linked structure via PVA is that the cast film was soluble in methanol at the initial stage but it becomes insoluble in methanol after few minutes. This indicates that PVAc change to PVA. After complete drying the film remain insoluble even in methanol and other organic solvents and water. Taking in to account the conducting network of polypyrrole and cross-linked structure of PVAc interpenetrating network (IPN) structure is expected in the films. The cross linked structure of the PVAc in the films imparts the better environmental stability to the film.
The dependence of conductivity on concentration of oxidizing agent (FeCl3) is shown in fig.5. Increase in conductivity with FeCl3 concentration up to 0.5 M, as seen from fig.5
Fig. 5 Dependence of conductiviy in polypyrrole films on concentration of Fecl3
during the polymerization of pyrrole was observed and it was reported that the PPy aggregates were connected with each other, which results in formation of the PPy network through out the film.
-
CONCLUSION
-
The PPy films were synthesized by chemical polymerization with different concentration of FeCl3 . It was noted that at 308 K maximum conductivity 7.4 X10-10 S/cm is observed for the PPy film synthesized by 0.5 M of FeCl3. The values of activation energy in both the regions I and II are minimum for PPy film synthesized by 0.5 M of FeCl3 and for lower and higher strength of FeCl3 it increases. The transference number measurement revels that the charge transport in the PPy films is predominantly due to ions.
REFERENCES:
[1] Shirakawa H, Louis E J, Mac Diarmid A. G., Chiang C. K., and Heeger A. J., 1977, J.Chem.Soc.Chem.Commun. 578. [2] Bredas J L and Street G B 1985, Acc. Chem. Res, 18, 309. [3] Bredas J L, Chnace R R and Silbey R, 1982, Phys. Rev., B70, 1132. [4] Akif Kaynak, 1998, Tr. J. Chemistry, 22, 81-85. [5] R. Gangopadhyay and A Das, 2000, J Appl. Phys., 87(5), 2363-2371.
[6] Swapan Chacrabarti, 1999, J.Chem. Phys., 110(18), 9305-9309. [7] Diaz A F, Kanazawa K K, Gardini G P, 1979, J. Chem Soc.Chem.Commun.635. [8] R. Noufi, A. J. frank and A.J. Nozik, 1980,J. Electrochem. Soc., 128,2596. [9] Radomir Cabla, Jiri Skarda and Karin Potje-Kamloth, 2000, Phys.chem.Chem.Phys, 2, 3283-3291. [10] Angeli A, 1916 Gazz. Chim. Ital., 46,229. [11] Mcneill R, Siadak R, Wardlaw J.H.and Weis D.E., 1963 Aust. J. Chem. 16,1056. [12] Dall'Olio A, Dascola Y, Vaaracca V and Bocchi V, 1968 Comptes Rendus, C267, 433. [13] D.C.Trivedi, 1999, Bull. Mater.Sci., 22(3), 447-455. [14] S.K.Dhawan and D.C. Trivedi, 1993, Bull. Mater.Sci., 16(5), 371-380.
[15] Wagner J.B. Jr. and Wagner C., 1957, J. Chem. Phys, 26, 1597. [16] J. Lee, Z. Cai, and C. R. Martin, 1992, Synthetic Metals, 46, 53. [17] Yun Eon Whang, Jun Hee and Seizo Miyata, A Novel Method to prepare Conducting polymer composites. [1] G. Eason, B. Noble, and I.N. Sneddon, On certain integrals of Lipschitz-Hankel type involving products of Bessel functions, Phil. Trans. Roy. Soc. London, vol. A247, pp. 529-551, April 1955. (references) [2] J. Clerk Maxwell, A Treatise on Electricity and Magnetism, 3rd ed., vol. 2. Oxford: Clarendon, 1892, pp.68-73. [3] I.S. Jacobs and C.P. Bean, Fine particles, thin films and exchange anisotropy, in Magnetism, vol. III, G.T. Rado and H. Suhl, Eds. New York: Academic, 1963, pp. 271-350. [4] K. Elissa, Title of paper if known, unpublished. [5] R. Nicole, Title of paper with only first word capitalized, J. Name Stand. Abbrev., in press. [6] Y. Yorozu, M. Hirano, K. Oka, and Y. Tagawa, Electron spectroscopy studies on magneto-optical media and plastic substrate interface, IEEE Transl. J. Magn. Japan, vol. 2, pp. 740-741, August 1987 [Digests 9th Annual Conf. Magnetics Japan, p. 301, 1982]. [7] M. Young, The Technical Writers Handbook. Mill Valley, CA: University Science, 1989.