
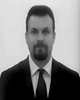
- Open Access
- Authors : Jose Elias Villa Herrera , Cornelio Alvarez Herrera, Antonio Campa Rodriguez , Jose Guadalupe Murillo Ramirez , Humberto Silva Hidalgo, Jose Luis Herrera Aguilar
- Paper ID : IJERTV9IS050365
- Volume & Issue : Volume 09, Issue 05 (May 2020)
- Published (First Online): 18-05-2020
- ISSN (Online) : 2278-0181
- Publisher Name : IJERT
- License:
This work is licensed under a Creative Commons Attribution 4.0 International License
The Interaction of Downflow and Pier Profile by Particle Image Velocimetry
Jose Elias Villa Herreraa, Cornelio Alvarez Herreraa, Jose Guadalupe Murillo Ramirezb, Humberto Silva Hidalgoa,
Jose Luis Herrera Aguilara, Antonio Campa Rodrigueza
a, Universidad Autónoma de Chihuahua, Facultad de IngenierÃa, Nuevo Campus Universitario, Circuito Universitario S/N, C.P, Chihuahua, Chih., México.
b, Centro de Investigación en Materiales Avanzados S. C., Miguel de Cervantes 120, Complejo Industrial Chihuahua. C.P, México.
Abstract:- This manuscript at a controlled laboratory level focuses on interaction, regarding an obstruction structure as a bridge pier, when water flow hits it. Within a scale hydraulic channel, immersed reflective tiny spheres and a laser lighting, it was observed the upstream downflow behavior, before three different pier profiles proposed, and utilizing the known particle image velocimetry technique, from a superior view, it was possible to obtain qualitative data from downstream turbulence degree, respectively. Therefore, in this manner, pier improvements could be considered in order to reduce the theoretical local scour.
Keywords: Downflow, Particle Image Velocimetry, Pier, Profile, Scour.
-
INTRODUCTION
The theoretical local scour, in general terms, occurs due to upstream water flow, when obstructed by pier, divides, going upwards and downwards known as downflow, up to hitting the bottom and subsequently surrounding the pier profile surface [3], causing a turbulent flow, generating a sort of vortex called the horseshoe coming from a strong gradient of pressure and being the main cause of soil erosion [8]. This behavior is similar to a vacuum cleaner suction, accumulating sediments further downstream [6]. Fig.1, shows the theoretical typical behavior of water flow interacting with an obstruction pier.
Fig. 1. Water flow interacting with obstruction pier.
-
LOCAL SCOUR
The scour is a result of erosive action of water streams on the bottom channel, especially in rainfall times when rivers flow rate is higher [10]. Local scour corresponds the sort of failure number one, faces expensive repairing costs [4], terminating service life of a bridge prematurely and even worse, cases where it collapses [1], causing traffic delays, road closures, and unfortunately human casualties [5]. It is important for a bridge over a water open channel to consider countermeasures for downflow and hydrodynamic profiles, in order to protect it from scour that may occur in that particular section, attempting to obtain the best design [7]. Fig. 2, shows evidence of horseshoe vortex scour in bridge pier.
Fig. 2. Evidence of horseshoe vortex scour in bridge pier.
-
PARTICLE IMAGE VELOCIMETRY
The known one as particle image velocimetry (PIV), it is a common utilized optical method to measure the velocity of fields, which is a real time, effective and non-destructive procedure [9]. Fig. 3, shows the PIV equipment and water channel. For subjects such as hydrodynamics and dynamics aspects, it observes the velocity of fields into a movement flow, becoming important for a variety of situations. The essential basic experimentation is measuring the velocity of flow by vectors direction for the best understanding. PIV is utilized in several research fields. Commonly this technique measures the instantaneous velocity distribution in two dimensions, by means of photographic movement determination of immerse water flow tiny spheres in a plane during a pretty short time lapse [2]. PIV applies to measure the distance, s, immersing particles (tiny spheres) into a flow in movement during a time lapse t. Where U means the velocity obtained under the following Eq. (1):
U s
t (1)
Fig. 3. PIV equipment and water channel.
-
METHODS
At controlled laboratory level was utilized the followed equipment: charge coupled device (CCD) camera previously calibrated, 532 nanometer green laser lighting, reflective 9-13µm spheres water immersed and hydraulic transparent plastic channel with 1.5HP electric pump, 0.50 m long, 0.15 m deep and 0.15 m width. Three different 0.02 m thickness pier profiles were proposed, squared, cylindrical and bladed. Afterwards videos were obtained from superior view water flow movement when obstructed by
pier respectively , each video was recorded taking for vector calculation a 24 x 24 pixels window at 700 microseconds, 40 images, then the final result was the average for data, which were processed utilizing a visualization software LaVision Davis8 and programming Octave software as post processing for vectors direction and vortex imaging. Water flow level, was 0.15 m, applying a velocity of 0.117 m/s, generating a flow rate of 1.5835 l/s, hence a Reynolds number obtained was Re = 4374.37, (Re >2000 is considered turbulent flow) under the following Eq. (2), where = water density; V = water velocity; r = hydraulic radius and µ = water dynamic viscosity.
Re Vr
(2)
-
IMAGING RESULTS
Imaging results as following Fig. 4(a), (b) and (c), shows photographs upstream downflow behavior utilizing immersed spheres and green laser lighting for the pier profiles proposed, then Figs. 5, 6 and 7, shows downstream water flow behavior, PIV superior view images for squared, cylindrical and bladed piers respectively, from which ones the third was the better hydrodynamic profile.
Fig. 4(a), (b) and (c). Regarding downflow, similar behavior for squared and cylindrical pier , bladed pier no significant evidence.
Fig. 5. Squared pier downstream water flow behavior.
Fig. 6. Cylindrical pier downstream water flow behavior.
Fig. 7. Bladed pier downstream water flow behavior.
-
CONCLUSIONS
In a qualitative manner, squared pier profile corresponds to the worst of them, due to turbulence and vortexes could be observed immediately behind it. Cylindrical pier profile shows an evident improvement because its roundness, being softer, it is a commonly profile utilized in the real field application for bridges construction. Both previous profiles, appreciably generate the called condition of downflow, therefore attempting to reduce it, the bladed profile is obtained, showing the lowest turbulence and vortexes of three ones, as seen in Figures. The PIV is a useful technique for this research, looking for a profile that provides enhancements for turbulent flow reduction, hence minor scour probability, at least at laboratory level.
-
ACKNOWLEDGMENTS
We want to thank: Eng. Carlos Eduardo Sánchez RamÃrez, Centro de Aeroespacial de la Facultad de IngenierÃa, Universidad Autónoma de Chihuahua, México.
REFERENCES
-
Bao T, Liu Z (2017) Vibrationbased bridge scour detection. A review: structural control and health monitoring 24:e1937, DOI: 10.1002/stc.1937.
-
Cengel Y A, Cimbala J M (2014) Real-time monitoring of bridge scour with magnetic field strength measurement. Fluid mechanics fundamentals and applications. Third edition in SI units chapter 15. Introduction to computational fluids dynamics 127.
-
Dargahi B (1990) Controlling mechanism of local scouring. Journal of Hydraulic Engineering. American Society of Civil Engineers 116(10)1197 1214, DOI:10.1061/(ASCE)0733-9429 (1990)116:10(1197).
-
Fisher M, Atamturktur S, Khan A A (2013) A Novel vibration-based monitoring technique for bridge pier and abutment scour. Structural health monitoring12, 2. SAGE publications11425, DOI:10.1177/1475921713476332.
-
Li H, Barkdoll B D, Kuhnle R, Alonso C (2006)Parallel walls as an abutment scour countermeasure. Journal of Hydraulic Engineering132, 5. American Society of Civil Engineers 51020, DOI:10.1061/(ASCE)0733-9429132:5(510).
-
Melville B W (1992) Local scour at bridge abutments. Journal of Hydraulic Engineering118, 4. 61531, DOI:10.1061/(ASCE)0733-9429118:4(615).
-
Radice A, Davari V (2014) Roughening elements as Abutment scour countermeasures. Journal of Hydraulic Engineering 140(8), 06014014, DOI:10.1061/(asce)hy.1943-7900.0000892.
-
Raudkivi A J (1986) Functional trends of scour at bridge piers. Journal of Hydraulic Engineering112, 1. 113, DOI:10.1061/(ASCE)0733-9429112:1(1).
-
Willert C E, Gharib M (1991) Digital particle image velocimetry. Experiments in fluids 10.181-193, DOI:org/10.1007/BF00190388.
-
Yang H C, Su C C (2015) Realtime river bed scour monitoring and synchronous maximum depth data collected during typhoon Soulik in 2013. Hydrological processes 29, 6. Wiley subscription services inc.105668, DOI:10.1002/hyp.10219.