
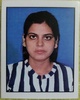
- Open Access
- Authors : Shilpi Saraswat, Prof. Rajesh Johari
- Paper ID : IJERTV12IS080060
- Volume & Issue : Volume 12, Issue 08 (August 2023)
- Published (First Online): 02-09-2023
- ISSN (Online) : 2278-0181
- Publisher Name : IJERT
- License:
This work is licensed under a Creative Commons Attribution 4.0 International License
Review of Advancements in Heat Transfer Enhancement Through Nanofluids
Shilpi Saraswat1
Research scholar, Dept. of Mathematics,
Agra College, Agra
(Dr. Bhimrao Ambedkar University, Agra,UP,INDIA)
Prof. Rajesh Johari2
Professor, Dept of Mathematics,
Agra College, Agra
(Dr. Bhimrao Ambedkar University, Agra, UP, INDIA)
Abstract-This comprehensive review article delves into the realm of heat transfer enhancement utilizing nanofluids, colloidal suspensions containing nanoparticles dispersed within conventional fluids. By synthesizing a wide spectrum of recent research, this review examines the intricate interplay between nanoparticle characteristics, concentration, and base fluid attributes, elucidating their collective impact on heat transfer efficiency. The analysis encompasses diverse mechanisms such as improved thermal conductivity, augmented convective heat transfer, and optimized phase-change behaviors. Challenges and opportunities associated with nanofluid applications are discussed, highlighting their potential to reshape the landscape of efficient heat exchange technologies. As ongoing research strives to refine nanoparticle dispersion, concentration optimization, and practical implementation, this review underscores the transformative potential of nanofluids in advancing sustainable and energy-efficient heat transfer processes.
Keywords- nanofluids, thermal conductivity, heat transfer, nanoparticles
-
INTRODUCTION
Over the past twenty years, extensive research efforts have been directed towards enhancing heat transfer processes within the context of industrial processes. Within any industrial process, heat is typically transferred between two fluid streams, usually from a hotter stream to a cooler stream due to the temperature differential between the fluids. This temperature difference serves as the driving force for heat transfer operations. Particularly within chemical process industries, heat transfer processes are executed through the utilization of heat exchangers.
In the domain of heat exchangers, the transfer of heat occurs through two primary modes: conduction and convection. Adhering to the principles of the first law of thermodynamics, achieving a complete equilibrium between the thermal energy entering and leaving a system is inherently challenging. Consequently, a certain amount of heat energy might be released from the heat transfer equipment to the surroundings, manifesting as heat loss.
This phenomenon significantly impacts both the energy requirements for the operation and the associated costs. As the quantity of lost heat energy increases, so does the overall production cost.
Mitigating heat losses during heat transfer processes is of paramount importance. This necessitates the careful selection of an appropriate heat exchanger type and configuration, with due consideration to the nature of the involved fluids. The selection of the optimal heat exchanger and a suitable working fluid play a pivotal role in ensuring efficient heat transfer operations. Moreover, in the context of heat loss, particular attention should also be paid to the thermal energy dissipated by automobiles and electronic devices.
The dissipation of waste heat energy is directly linked to energy consumption, and a substantial rise in energy consumption significantly amplifies the release of heat energy. This effect is particularly pronounced in power electronics devices and high-powered automotive engines. Maintaining thermal equilibrium in electronic and automotive systems holds immense importance in the global economy. Achieving this equilibrium in electronic devices presents a formidable challenge, leading to extensive research efforts aimed at identifying viable solutions.
Central to this challenge is the issue of heat energy dissipation, which contributes to an increase in the Earth's temperature. This temperature elevation plays a pivotal role in influencing changes in the Earth's climate patterns. Additionally, the escalation of heat energy within electronic devices impairs their operational efficiency, diminishes their dependability, and alters the inherent characteristics of electronic components.
Nanofluids are created through the dispersion of nanoparticles with dimensions at the nanoscale into base fluids such as water, oil, and ethylene glycol. This blending
of nanoparticles into conventional fluids leads to a noteworthy augmentation of both the physical and chemical attributes of the base fluids. Consequently, nanofluids have garnered considerable attention across various sectors, particularly within heat transfer applications. The formulation of nanofluids involves the utilization of diverse materials including metals, metal oxides, carbides, and carbon nanotubes (CNTs).
Traditionally, fluids like water, oil, and ethylene glycol have been favored as primary working mediums in a multitude of heat transfer functions, particularly in cooling processes. However, the intrinsic limitation in thermal conductivity exhibited by these conventional heat transfer fluids can lead to diminished heat transfer rates and subsequently compromise the efficiency of cooling systems. An effective strategy to address this limitation involves introducing nanoparticles with nanoscale dimensions into these conventional fluids. This infusion of nanoparticles tangibly improves the thermal attributes of the fluids and augments their inherent heat transfer capacities.
The process of selecting an appropriate nanoparticle assumes paramount importance from the array of nanoparticles available. This selection hinges on various thermo-physical properties intrinsic to the nanoparticles, encompassing aspects such as density, viscosity, specific heat capacity, thermal expansion coefficient, and thermal conductivity. These factors need to be meticulously evaluated when considering a nanoparticle for applications involving cooling processes.
In this context, the subsequent literature surveys encompass subjects such as the importance of Nanofluids and Hybrid nanofluids in the realm of heat transfer applications, the synthesis procedures for nanoparticles, nanofluids, and hybrid nanofluids, the comprehensive analysis of nanoparticle and nanofluid properties, the utilization of microstructured heat exchangers in heat transfer scenarios. These reviews have been compiled and are presented below.
-
NANOFLUIDS
Nanofluids have emerged as a promising class of heat transfer fluids due to their unique properties and potential for enhancing thermal performance in various applications. Nanofluids are colloidal suspensions containing nanoparticles of sizes typically ranging from 1 to 100 nanometers dispersed within a base fluid, often water or a traditional heat transfer fluid. The concept of nanofluids originated as a means to improve the thermal conductivity of conventional heat transfer fluids by incorporating nanoparticles with high thermal conductivity. This
enhancement in thermal conductivity stems from the increased surface area of nanoparticles and their potential to facilitate heat conduction.
-
THERMAL CONDUCTIVITY OF NANOFLUIDS The thermal conductivity of nanofluids is a crucial parameter that plays a significant role in their heat transfer enhancement capabilities.Several factors contribute to the enhancement of thermal conductivity in nanofluids: nanoparticle material, particle concentration, particle size,
base fluid properties, particle dispersion, temperature and particle interactions. Many researchers experimentally and numerically investigated enhancement of thermal conductivity of nanofluids. Mohammad Hemmat Esfe et al. (2015) experimentally found that with increasing volume fraction of nanoparticles relative thermal conductivity enhances. The increasing temperatures also increase the thermal conductivity of nanofluids, although its effect on the thermal conductivity compared to the effect of volume fraction, was lower. Mohammad Hemmat Esfe et al. (2016) investigated the thermal conductivity of CNTs Al2O3/water hybrid nanofluids. Measured results revealed that the thermal conductivity of nanofluids highly depends on the solid volume fraction, and at high solid volume fractions the temperature effect is notable. Pham Van Trinh et al. (2017) shown that Thermal conductivity enhancement increases as the GrMWCNT/Cu concentration in the nanofluid increases. The enhancement of thermal conductivity at different temperatures was also considered. Reza Naghdi Sedeh et al. (2019) studied the effect of temperatures, the CuO and TiO2 nanoparticles' mass fraction, and basefluid types on thermal conductivity of nanofluid. The results showed that the thermal conductivity changes with the increase in temperature; consequently, with the increase in temperature the thermal conductivity of the nanofluid increases. Wai Kit Woo et al. (2021) found that an increase in thermal conductivity and a decrease in viscosity of the GO nanofluid at high temperature which favour the heat transfer process are associated to the weakening of the intermolecular forces of the nanoparticles. The GO nanoparticles synthesized under the laser frequency of 10 Hz manifest a maximum thermal conductivity enhancement of 82% at a temperature of 50
C while the maximum viscosity increment is recorded as 212% at a temperature of 30 C. Jun Qin et al. (2023) experimentally investigated the effects of different factors, including nanoparticle size and type, volume fraction, and base fluid, on the thermal conductivity enhancement of nanofluids. The experimental results indicated that the thermal conductivity enhancement of nanofluids is proportional to the thermal conductivity of the nanoparticles, with the enhancement being more
pronounced for fluids with lower thermal conductivity. Meanwhile, the thermal conductivity of nanofluids decreases with increasing particle size and increases with increasing volume fraction.
-
HEAT TRANSFER ENHANCEMENT BY USING
NANOFLUIDS
Heat transfer enhancement using nanofluids has garnered substantial attention in recent years as a promising approach to improving the efficiency of various heat exchange processes. M.A.Ahmed et al. (2015) investigated the flow and thermal characteristics of turbulent forced convection flow in triangular-corrugated channel using nanofluids have been numerically investigated over Reynolds number rage of 10005000. Four different types of nanofluids, comprising Al2O3, CuO, SiO2, and ZnO water with nanoparticle diameters ranging from 30 to 70 nm and nanoparticle volume fractions varying from 0 to 4%, were presented. It was observed that the average Nusselt number, pressure drop, heat transfer enhancement, and thermal-hydraulic performance increased as the volume fraction of nanoparticles increased and the diameter of nanoparticles decreased. The utilization of SiO2water nanofluid with nanoparticle diameters of 30 nm and a volume fraction of 4% was found to yield the highest thermal-hydraulic performance across the entire Reynolds range. Hussein Talal Dhaiban (2016) found that the heat transfer coefficient and Nusselt number increased as the Reynolds number and particle concentration were increased. The maximum enhancements in Nusselt number and heat transfer coefficient were 9.5% and 13.5%, respectively, at a Reynolds number of 7100 and a particle volume fraction of 4%. The results of the nanofluids also exhibited good agreement with the available empirical correlation at particle volume fractions of 1%, 2%, and 3%. However, a slight deviation was observed at volume fractions of 4%. T. Hayat, S. Nadeem (2017) examined the three dimensional steady rotating flow of hybrid nanofluid (AgCuO/water) with thermal radiation, heat generation and chemical reaction on a linearly stretching surface. They concluded that hybridity boosts the temperature distribution as well as the heat transfer rate at surface. The thermal boundary of hybrid nanofluid (Ag CuO/water) increased by incrementing the heat generation parameter. Raheem K. Ajeel et al. (2018) were conducted numerical simulations of turbulent forced convection of nanofluids containing silicon dioxide, aluminum oxide, zinc oxide, and copper oxide nanoparticles flowing in semicircular zigzag corrugated channels over a Reynolds number (Re) range of 10,000 Re30,000. The major conclusion drawn from this numerical study was that the utilization of nanofluids in corrugated channels could be
considered as a suitable approach to achieving optimal thermal performance. This finding could guide the design of heat exchangers, potentially leading to more compact configurations. V. Kiseev, O. Sazhin (2019) investigated the heat transfer performance of a loop thermosyphon using Fe2O3/water nanofluid and Ir/water nanofluid as working fluids.the study of nanofluid stability revealed that nanoparticles tended to agglomerate and deposit on the vaporization surface over time, resulting in the formation of a micro/nano relief on this surface. This micro/nano relief enhanced heat and mass transfer during pool boiling, as compared to a technically smooth surface. Muhammad Qasim et al. (2020) found that the thermal performance as well as pressure drop and friction factor of nanofluids is strongly reliant on the volume flow rate and volumetric concentration of nanoparticles. Therefore, heat transfer rate, overall heat transfer coefficient and Nusselt number of nanofluids increases by increasing the volume flow rate and volumetric concentration of nanoparticles. The thermal performance of mini-channel heat sinks for different fin spacing (0.2 mm, 0.5 mm, 1 mm, and 1.5 mm) was investigated by Muhammad Anwar et al. (2020) numerically using CuO-water nanofluids with volumetric concentration of 1.5%. The numerical values computed were than compared with the literature and a close agreement was achieved. They recorded the minimum base temperature of chip to be 36.8 °C for 0.2 mm fin spacing heat sink. A reduction of 9.1% in base temperature was noticed using CuO-water nanofluids for 0.2 mm fin spacing as compared to previously experimental estimated value using water. Muhammad Bilal Hafeez et al. (2021) investigated the heat transfer enhancement in nanofluids using three different nanofluids. The mathematical models were described in the form of partial differential equations (PDEs) along with prescribed boundary conditions. A thermal slip boundary was introduced, and the dynamics of nanofluid flow were discussed using the bvp4c solver in MATLAB through a system of coupled nonlinear ordinary differential equations (ODEs). S. Wiriyasart et al. (2021) demonstrated that a thermal cooling enhancement was employed to dissipate heat from the hot side of the TEM, aiming to decrease the temperature difference between the module's hot and cold sides. This was achieved by utilizing dummy battery packs as cooling loads and employing water, TiO2 nanofluid, and Fe3O4 ferrofluid as coolants, with slight incremental changes in concentration. At a low Reynolds number of 320, thermal enhancements of 4.80% and 13.17% were obtained when Fe3O4 ferrofluid (0.015%v) was used as the coolant compared to TiO2 nanofluid (0.015%v) and deionized water. For Reynolds numbers greater than 535, the enhancements became 11.17% and 12.57%, respectively. Chemseddine Maatki
(2022) examined three-dimensional numerical investigation of the effects of natural double diffusive convection in triangular solar still and comparison of thermal and mass performance between single-stage (case
-
and two-stage seawater feed design (case 2) with the addition of water-CNT nanofluid. The investigation was cnducted by varying the Rayleigh number between 103 and 106 , the buoyancy ratio, the volume fraction of nanoparticles (0 10%), and the length, Lp, of the second seawater feed stage between 0.1 and 0.5. The average heat transfer rate at the condensing surface was higher than at the absorber plate. However, it was observed that the mass transfer was lower at the condensing surface. The two-stage seawater feed design demonstrated an enhancement in heat and mass transfer rates compared to the single-stage configuration. Upon comparing the two- stage feed configuration (case 2) with the single-stage case, a heat transfer rate enhancement of 15% was observed at the hot surface starting from Ra=5 × 10. Furthermore, a mass transfer improvement of approximately 65% was observed at the condensing surface for all studied Rayleigh values. R. Vinoth et al. (2023) experimentally investigated the heat and flow characteristics of curved MCHS using water and hybrid nanofluids. the heat and flow characteristics revealed better results for the curved channel heat sink. It exhibited an experimentally observed increase in heat transfer rate and a drop in pressure by 11.98% and 30.1%, respectively, compared to the straight channel. Brahim Mahfoud(2023) investigated the walls electrical conductivity effect on heat transfer enhancement of nanofluid swirling flow in a cylindrical container in the existence of an axial magnetic field. The addition of nanoparticles (Cu) to pure water upgraded the heat transfer in swirling flows by decreasing the number of fluid layers and the boundary layer thickness.
-
-
CHALLENGES AND OPPORTUNITIES ASSOCIATED WITH NANOFLUID
APPLICATIONS
While nanofluids offer promising advantages, they also present certain challenges and opportunities that need to be carefully considered for successful implementation. challenges with nanofluids are:
-
Ensuring stable dispersion of nanoparticles in the base fluid over extended periods can be challenging
-
At higher nanoparticle concentrations, nanofluids can exhibit increased viscosity, leading to higher pumping power requirements and potential flow issues in heat exchangers and pipelines.
-
Nanofluids may exhibit higher pressure drops compared to base fluids due to increased viscosity
or flow resistance, affecting system efficiency and design.
-
Production and dispersion of nanoparticles can be costly and energy-intensive
Opportunities with nanofluids are:
-
Enhanced Heat Transfer Efficiency
-
Energy Savings
-
Advanced Thermal Energy Storage
-
Materials Science Advancements
-
-
CONCLUSION
In conclusion, this review article has delved into the fascinating realm of heat transfer enhancement through the utilization of nanofluids. The study of nanofluids, which are colloidal suspensions of nanoparticles in conventional base fluids, has shown immense promise in significantly improving heat transfer characteristics in various applications.
Through an extensive analysis of recent research, it is evident that nanofluids offer a multitude of advantages, including increased thermal conductivity, enhanced convective heat transfer coefficients, and improved overall heat transfer rates. These enhancements can be attributed to the unique properties and behaviors of nanoparticles, such as their high surface area-to-volume ratio and Brownian motion effects, which augment heat transfer mechanisms. While the potential of nanofluids is undeniable, it is important to acknowledge the challenges and limitations that still require further investigation. Issues such as long- term stability, potential agglomeration, and environmental impacts must be thoroughly addressed to ensure the practicality and sustainability of nanofluid-based heat transfer enhancement technologies.
In summary, the synthesis of research findings presented in this review article underscores the immense potential of nanofluids as a promising avenue for advancing heat transfer efficiency in diverse applications. As researchers continue to unravel the complexities and possibilities within this field, it is clear that nanofluids hold the promise of revolutionizing heat transfer processes and contributing to more energy-efficient and environmentally conscious engineering solutions.
REFERENCES
[1] Ahmed, M. A., Yusoff, M. Z., Ng, K. C., & Shuaib, N. H. (2015). Numerical investigations on the turbulent forced convection of nanofluids flow in a triangular-corrugated channel. Case Studies in Thermal Engineering, 6, 212-225. [2] Dhaiban, H. T. (2016). Numerical study of heat transfer enhancement in heat exchanger using Al2O3 nanofluids. Journal of Engineering, 22(4), 98-115. [3] Hayat, T., & Nadeem, S. (2017). Heat transfer enhancement with AgCuO/water hybrid nanofluid. Results in physics, 7, 2317-2324. [4] Ajeel, R. K., Salim, W. S., & Hasnan, K. (2018). Numerical investigations of flow and heat transfer enhancement in a semicircle zigzag corrugated channel using nanofluids. International Journal of Heat and Technology, 36(4), 1292-1303. [5] Kiseev, V., & Sazhin, O. (2019). Heat transfer enhancement in a loop thermosyphon using nanoparticles/water nanofluid. International Journal of Heat and Mass Transfer, 132, 557-564. [6] Qasim, M., Sajid Kamran, M., Ammar, M., Ali Jamal, M., & Yasar Javaid, M. (2020). Heat transfer enhancement of an automobile engine radiator using ZnO water base nanofluids. Journal of Thermal Science, 29, 1010-1024. [7] Anwar, M., Tariq, H. A., Shoukat, A. A., Ali, H. M., & Ali, H. (2020). Numerical study for heat transfer enhancement using CuO water nanofluids through mini-channel heat sinks for microprocessor cooling. Thermal Science, 24(5 Part A), 2965-2976. [8] Hafeez, M. B., Amin, R., Nisar, K. S., Jamshed, W., Abdel-Aty, A. H., & Khashan, M. M. (2021). Heat transfer enhancement through nanofluids with applications in automobile radiator. Case Studies in Thermal Engineering, 27, 101192. [9] Wiriyasart, S., Suksusron, P., Hommalee, C., Siricharoenpanich, A.,& Naphon, P. (2021). Heat transfer enhancement of thermoelectric cooling module with nanofluid and ferrofluid as base fluids. Case Studies in Thermal Engineering, 24, 100877.
[10] Maatki, C. (2022). Heat transfer enhancement using CNT-water nanofluids and two stages of seawater supply in the triangular solar still. Case Studies in Thermal Engineering, 30, 101753. [11] Vinoth, R., Sachuthananthan, B., Vadivel, A., Balakrishnan, S., & Raj, A. G. S. (2023). Heat transfer enhancement in oblique finned curved microchannel using hybrid nanofluid. International Journal of Thermal Sciences, 183, 107848. [12] Mahfoud, B. (2023). Effect of Wall Electrical Conductivity on Heat Transfer Enhancement of Swirling Nanofluid-Flow. Journal of Nanofluids, 12(2), 418-428. [13] Esfe, M. H., Yan, W. M., Akbari, M., Karimipour, A., & Hassani,M. (2015). Experimental study on thermal conductivity of DWCNT- ZnO/water-EG nanofluids. International Communications in Heat and Mass Transfer, 68, 248-251.
[14] Hemmat Esfe, M., Saedodin, S., Yan, W. M., Afrand, M., & Sina, N. (2016). Study on thermal conductivity of water-based nanofluids with hybrid suspensions of CNTs/Al 2 O 3 nanoparticles. Journal of Thermal Analysis and Calorimetry, 124, 455-460. [15] Van Trinh, P., Anh, N. N., Thang, B. H., Hong, N. T., Hong, N. M., Khoi, P. H., … & Hong, P. N. (2017). Enhanced thermal conductivity of nanofluid-based ethylene glycol containing Cu nanoparticles decorated on a GrMWCNT hybrid material. RSC advances, 7(1), 318-326. [16] Sedeh, R. N., Abdollahi, A., & Karimipour, A. (2019). Experimental investigation toward obtaining nanoparticles' surficial interaction with basefluid components based on measuring thermal conductivity of nanofluids. International Communications in Heat and Mass Transfer, 103, 72-2. [17] Woo, W. K., Hung, Y. M., & Wang, X. (2021). Anomalously enhanced thermal conductivity of graphite-oxide nanofluids synthesized via liquid-phase pulsed laser ablation. Case Studies in Thermal Engineering, 25, 100993. [18] Qin, J., Tao, Y., Liu, Q., Li, Z., Zhu, Z., & He, N. (2023).Experimental and Theoretical Studies of Different Parameters on the Thermal Conductivity of Nanofluids. Micromachines, 14(5), 964.