
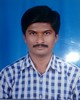
- Open Access
- Authors : P. Sureshkumar , Dijo Joseph , Krishnaprasad .V , Mohammad Aneesh Pacheri, Yadhukanan
- Paper ID : IJERTV11IS060044
- Volume & Issue : Volume 11, Issue 06 (June 2022)
- Published (First Online): 09-06-2022
- ISSN (Online) : 2278-0181
- Publisher Name : IJERT
- License:
This work is licensed under a Creative Commons Attribution 4.0 International License
Investigation and Safety Measures of Fire Accidents in Electric Vehicles
P.Sureshkumar
HoD Automobile Engineering
JCT College of Engineering and Technology Coimbatore.
Krishnaprasad .V
UG Student Automobile Engineering JCT College of Engineering and Technology
Coimbatore.
Dijo Joseph
UG Student Automobile Engineering JCT College of Engineering and Technology
Coimbatore.
Yadhukanan
Mohammad Aneesh Pacheri
UG Student Automobile Engineering JCT College of Engineering and Technology
Coimbatore.
UG Student Automobile Engineering JCT College of Engineering and Technology
Coimbatore.
Abstract: Electric vehicles (EVs) have revolutionized the automobile industry worldwide in the previous decade, owing to the rapid advancement of Li-ion battery technology. The fire danger and hazard associated with this sort of high-energy battery, on the other hand, has become a serious safety problem for electric vehicles. This article concentrates on the most recent EV fire-safety concerns, such as thermal runaway and fire in Li-ion batteries. Extreme misuse circumstances, such as those caused by incorrect operation or traffic accidents, might result in thermal runaway or fire. The discharge of hazardous gas, fire, jet flames, and explosive may occur as a result of the battery's failure. This article examines battery fires in battery electric vehicles, hybrid electric vehicles, and electric buses. Furthermore, key battery fire properties gained via testing are analyzed in various EV fire situations. Because the burning battery pack within is inaccessible to outside applied suppressants and can re-ignite without appropriate cooling, suppressing EV fires becomes more difficult once the onboard battery is involved in a fire. As a result, a large amount of suppression agent is required to cool the battery, extinguish the fire, and prevent it from re-igniting. This review aims to assist researchers and industries working in the fields of batteries, electric vehicles, and fire safety engineering by addressing these concerns, encouraging active research collaborations and attracting future research and innovation on increasing the global safety of future EVs. Only then will society come to terms with EVs in the same way that it has with cars.
Keywords: Li-ion battery, Electric vehicle, Fire incidents, Fire tests, Heat release rate, Fire suppression
-
INTRODUCTION
The electric vehicle (EV) is propelled by an electric motor and runs on electricity. The word "electric vehicle" normally refers to automobiles, although it may also apply to rail vehicles, surface and underwater watercraft, and aerospace applications. This word is used in this study to refer to road EVs that are powered entirely or partially by a Li-ion battery (LIB). Battery electric cars (BEVs) run entirely on electricity, although plug-in hybrid electric vehicles (PHEVs) and hybrid electric vehicles (HEVs) may run on gasoline as well.
The electric vehicle (EV) was created in the 1800s as a result of a succession of developments in battery and electric motor technology [1]. Due to fuel shortages and natural calamities in the 1900s, EVs were in high demand for a brief while [2]. After the 1930s, however, interest in EVs waned as oil and gasoline became more affordable and widely available, allowing gasoline-powered cars to go faster and further [3]. Currently, billions of internal combustion engine vehicles (ICEVs) are in use, accounting for around 87 percent of all cars on the road. Petroleum accounts for approximately 33% of our global energy [4]. Limited natural energy resources, a growing global population, and global warming, on the other hand, intensify people's perceptions of energy susceptibility and the need for more environmentally friendly transportation options.
EVs returned to the world arena in the twenty-first century, accompanied by the fast growth of the LIB during the 1990s. EVs are now not only a symbol of environmentally friendly transportation, but they also offer exceptional driving performance [1,2]. EVs, on the other hand, are still in their infancy compared to ICEVs, which have been in continuous usage and development for the past century. This is especially true when it comes to their apparent fire safety. This safety risk is preventing the EV from becoming the dominant mode of transportation [5,6].
The battery is not only the source of electricity for the EV, but also the primary source of fuel for the EV fire, just like gasoline or diesel is the primary source of fuel for ICEV flames. In [1720], the processes of battery thermal runaway, as well as battery fire phenomena, dangers, and hazards, are discussed. The safety properties of battery material and chemistry were highlighted in these evaluations, which also detailed contemporary scientific understandings of battery fire phenomena.
However, the overall fire risk and dangers of electric vehicles are yet unknown. Large-scale EV rechargeable batteries and full-scale EVs need expensive fire testing, which are rarely disclosed. EV ownership is steadily expanding as the electric vehicle market expands. Despite unresolved fire-safety difficulties, the energy density of LIBs continues to rise [21]. As a result, the likelihood of an EV fire will rise. This study examines these fire dangers, as well as incidents involving electric vehicles (EVs) powered by batteries, particularly the LIB. The limited large-scale fire testing of EV, as well as the accompanying fire-protection techniques, are also thoroughly discussed.
Figure 1. Typical EV fire accidents in recent years
-
The Growing Demand for Electric Vehicles
In many nations and locations throughout the world, the electric vehicle market has performed admirably. China dominated the market in 2018, with a 48 percent share [27], followed by the European Union with a 26 percent share. Although people are more resistive to new technologies ("social" hurdles) [28], EVs are currently less mature and dependable than gasoline-powered cars in terms of lifetime, range, and charging station availability ("technical" barriers). Government subsidies, on the other hand, have proven to be a powerful motivator in the electric vehicle industry. In reality, in every major market, government policy has a significant impact on EV sales and trajectory. Furthermore, the EV industry is being boosted by ongoing battery cost reductions, as well as improved battery performance and increased manufacturing volumes.
Figure 2. Sales percentage of EV in the global vehicle market
The European Union is leading the way in boosting the electric vehicle industry, thanks to the encouragement of beneficial government policies. In 2017, EVs accounted for 34.7 percent of new car sales in Norway, rising to 52 percent when hybrid cars are included [30]. (1) BEVs are exempt from vehicle registration tax; (2) BEVs are exempt from value-added tax; and (3) BEVs pay the lowest rate of the vehicle licencing charge given by Norway's government. Finally, BEVs can use bus lanes and are not subject to tolls [31]. In terms of hybrid and electric passenger car registrations on the European continent, France is second only to Norway [32]. According to an Electric Car Index developed by OSV Ltd, France has surpassed Norway as the global leader in electric vehicles. The government plans to invest 400 million on EV research and development. In 2017, 11,987 electric car charging stations were established, with electric vehicles accounting for 1.5 percent of the French personal vehicle market. s part of its long-term zero-emission mobility strategy, Germany has set the aim of being the leading market and provider for electric mobility by 2020 [33]. Several strategies for EV research and development have been undertaken by the German government (R&D). For example, $240 million will be spent on batteries for electric automobiles, prioritising domestic manufacture and ensuring that German professionals are trained in the subject.
In the United States, the number of electric vehicles on the road increased from 9,750 in 2011 to 762,000 in 2017 [29]. This improvement is attributed in part to state regulations and lower battery prices [34]. Since 2010, the federal government of the United States has offered a tax credit for PEVs purchased. The tax credit for each car varies from $2,500 to $7,500, depending on the battery capacity and gross vehicle weight classification. Other priority measures, such as high occupancy vehicle (HOV) lane exemptions and accelerated licence plate purchases, have been given in addition to cash incentives. Extending governmental incentives for electric vehicles until 2020 allows for further industry development.
Since 2009, the Chinese government has provided considerable subsidies for the purchase of electric vehicles [36]. These subsidies, which are part of China's Electric Vehicle Subsidy Scheme, were revised in 2013 to scale with an EV's electric driving range rather than battery capacity in order to encourage EV quality and safety. China's subsidies scheme aims to increase the appeal of electric vehicles while also creating national champions and e-mobility ecosystems for the future decades. By 2040, China is expected to have 200 million electric vehicles on the road, accounting for 60% of total passenger car sales [29].
Because of its limited natural resources, Japan has a lengthy history with electric vehicles. The Nissan Tama, the world's first electric vehicle, debuted on the road in 1947 . Japan has made significant investments in battery development and was one of the first countries to offer plug-in hybrid electric vehicles to the worldwide market . As a result, the Nissan LEAF and Toyota Prius, two of the most popular EVs on the market today, are Japanese. Aside from the nations listed above, several more throughout the world offer significant tax breaks for EV purchase and ownership. Singapore, the Netherlands, and Ireland are three examples [29].
-
EV Batteries and their Fire Risk
One of the dangers that surrounds cars is fire. They've starting to become increasingly noticeable in EVs as the quantity of EVs grows. The majority of BEV and PHEV fires, especially those involving self-ignition, begin in the battery power system (Figure 1). The battery capacity can be compared to the gasoline capacity in an ICEV's fuel tank in terms of propulsion. As a result, EV fires are linked to the fire risk and danger associated with the battery cell and power system, as well as the battery pack's size and capacity. The bigger the number of batteries and the quantity of
energy they can hold, the greater the risk of an EV catching fire [18].
Figure 3. EV battery cells: (a) the pouch cell (b) the prismatic cell;(c) the cylindrical cell(d) approximate battery cell size of popular EVs (e) the 60 kWh battery pack is fully assembled by LG Chem in Korea, which employs 288 prismatic pouch cell(f) Teslas battery module, which consists of hundreds of cylindrical cells (g) Nissan LEAF battery pack .
Electric vehicles may use a significant number of batteries. This is important since an electric vehicle's power consumption is hundreds of times more and quicker than that of a typical smartphone. Electric vehicle batteries must have a lot of power (up to 100 kW) and a lot of energy capacity (up to tens of kWh). Simultaneously, they overcome considerable obstacles relating to space and weight constraints while being cost-effective . EV batteries are typically made up of cells, modules, and a pack . The fundamental unit of a LIB is the battery cell, which is linked in series or parallel to form a battery module. The cells are held together by a frame that protects them from external shocks, heat, and vibration. The battery pack is the component that connects the modules to the infrastructure of the pack. Structures, wiring, cooling loops, and power electronics are all part of this infrastructure. Several modules are also equipped with power, charging/discharging, and temperature management systems. The Battery Management System is the common name for this (BMS). The EV can store a lot of energy thanks to this compact construction. However, this makes controlling the temperature inside the pack difficult
. The driving performance of an electric vehicle is determined by the qualities of individual battery cells. In electric vehicles, traditional battery technologies like lead-acid, nickel-cadmium (NiCd), and nickel-metal hydride (NiMH) have all been employed. Despite having a lesser fire danger than LIBs, they have a lot lower energy density and capacity, as well as a much slower charging and discharging rate. As a result, they are inappropriate for current electric vehicles. The LIB has been widely utilised in many types of electrical devices, including EVs, since Dr. Goodenough created it in 1980 and Sony commercialised it in 1991. The LIB now leads the EV industry and is projected to continue to do so in the next decades [21,29]. LIBs are popular because of their high energy density and small size.
As shown in Figure 3, there are three types of cells based on their design and manufacturing process: cylindrical cells, prismatic cells, and pouch cells. All three types of cells are utilised in actual automobiles, and Table 1 shows the fundamental characteristics of three different battery cells. LIB cells used in EVs typically have capacities ranging from 3 to 300 Ah, depending on the kind and manufacturer. Table 2 shows that the usual energy density of a vehicle is greater than 100 Wh/kg. The chemistry and architecture of the LIB cell are responsible for this energy. Tesla, for example, employs NCA (nickel, cobalt, and aluminium oxide) in their cylindrical 18650 cell, which has a specific energy of 3.4 Ah per cell and 248 Wh/kg.
The Tesla Model S has the biggest energy capacity among passenger EVs, at over 100 kWh. On a single charge, its energy capacity may provide a driving range of around 380 kilometres . However, the risk of an EV fire grows as the quantity and capacity of batteries (or fuel) increases, as does the potential fuel load [18].
Table 1. Basic parameters of three typical battery cells.
Manufacturer
Configuration
Geometry [mm]
Voltage [V]
Capacity [Ah]
Specific Power [W/kg]
Weight [g]
Panasonic
Cylindrical
18.5×65.3
3.6
3.2
120
48.5
Hitachi
Prismatic
148×91×26.5
3.6
28
2300
720
Kokam
Pouch
462×327×15.8
3.6
240
360
4780
Table2. Battery pack information for selected BEV with a specific model and range test [26]
Brand
Capacity [kWh]
Cell density [Wh/kg]
Cell type
Range [km]
Nissan Leaf S (2017) [55]
40
229
Pouch
243 (EPA)
Renault Zoe 40 (2017) [56]
41
228
Pouch
400 (NEDC)
BMW i3(2016) [57]
42.2
230
Prismatic
246 (EPA)
Tesla Model S (2017) [58]
90
~250
Cylindrical 18650
509 (NEDC)
The fire danger and LIB has become a serious safety problem since the Li-ion battery became the primary power source for EVs a decade ago. This is due to the rising size of deployment and battery pack energy density. Lithium (as a chemical element) is associated with concerns about safety [18,29,30]. When a Li-ion battery is hit from the outside and subjected to harsh working circumstances, it can shatter, ejecting sparks, flammable gases, and poisonous fumes that can be ignited further, resulting in steady combustion, jet flames, or a gas explosion [21,31,32].
Even while a standard battery system has a minimal chance of self-ignition [33,34], it is susceptible to external thermal, mechanical, and electrical effects that can occur under extreme operating circumstances or events. Electrical hits and harsh working circumstances, on the other hand, are uncommon for most portable electronic devices, such as the laptop and smartphone, but they are regarded typical. The operation condition for an EV battery, on the other hand, is more harsh due to the frequent ascent and descent under complicated road and traffic circumstances. Furthermore, the battery capacity (or fuel load) of an electric vehicle is hundreds of times that of a portable electronic item, posing a larger fire risk in the event of thermal runaway and igniting. The safety features built into the EV and battery pack architecture, on the other hand, are more sophisticated, minimising the likelihood of (spontaneous) failure. As a result, assessing the battery fire danger in EVs based on the perception of battery fire risk in portable electronic devices is inaccurate.
Thermal Impact: Users want to be able to drive their electric car in the same conditions as a conventional internal- combustion vehicle, including extreme cold and heat. EVs are predicted to be usable in places like California, where summer temperatures may reach 45°C, or in Norway and Canada, where daily winter temperatures are below -5°C and occasionally below -15°C. The battery, like people, functions best at ambient temperature (20-30°C). Extremely hot or cold conditions are detrimental to the battery's functioning and reduce its longevity (Figure 4). Some undesirable chemical reactions can occur in high-temperature situations, resulting in overheated batteries [18,30]. It is possible to cause a thermal runaway[18,33] with a low thermal dispassion ability, which can eventually lead to an EV fire. The internal resistance of the battery increases as the temperature drops. This resistance can accelerate the formation of metallic dendrites [1] as well as generate extra heating effects within the battery, increasing the likelihood of a battery fire [18].
Figure 4. LIBs should operate within a limited temperature and charge range to warrant optimal life and safety [5].
Reprinted with permission.
Mechanical Impact: Without the protection of an EV framework and/or a battery module and pack container, most commercial LIB cells are relatively brittle. An EV, like any other conventional vehicle, may be involved in a traffic collision over its lifespan. Nonetheless, thanks to contemporary LIB and EV design, the vast majority of accidents will not destroy the battery [26,27]. The goal of integrating LIB packs into highly reinforced portions of the vehicle (see Figure 5) is to eliminate the chance of being pierced during an accident. However, at high speeds, as some EVs may attain in a short amount of time [32], even the best degree of protection is unable to reliably avoid fire (see Table 1).
Figure 5. (a) LIBs are typically located inside the safe-zone [66], and (b) battery layout for a Nissan Leaf [68].
Electrical Abuse: The goal of quick charging and discharging, as well as high driving performance, has a detrimental impact on EV fire risk . LIBs are designed to accept and store a specific quantity of energy over a specific period of time. Excessing these limitations, which can occur as a result of charging too rapidly or overcharging, can cause performance degradation or early failure. Joule heating and interior chemical reactions are common side effects of electrical misuse. The former produces heat, while the latter may eventually result in an internal short circuit. As indicated in Table 1, several EV fires are caused by improper operating circumstances and internal problems, such as a short circuit in the high voltage circuit, overcharging, and an overheated environment. Most sorts of electrical abuse, on the other hand, are impossible for many EVs provided their BMS is properly constructed and operates . A considerable part of the situations where "self- ignition" or "spontaneous ignition" occurrences have happened, aside from battery cell failure, are believed to be due to poor manufacturing and design practices, as well as insufficient electronic control systems, BMS, and power transmission control systems.
Depending on SOC, a battery fire can release 5-10 times more energy of the stored electrical energy (or kinetic energy), as shown in Figure 6(b).
Figure 6. (a) Stored energy and mass of common commercial LIBs of different scales are listed in Table 3, (b) the comparison of flame heat from battery fire, (c) fire heat release of burning vehicle fuel vs. range.
It's worth mentioning that a gasoline car with a normal fuel tank capacity of 45-65 L (i.e., 35-50 kg gasoline) might have a longer range (e.g., over 800 km), although the amount of gasoline consumed decreases with driving distance. In contrast, the overall mass of EV fuel (LIBs) does not decrease when the EV is driven, and the potential heat emission from burning LIB does not fluctuate considerably in the SOC range of 20 to 100% (see Figure 6a-b) . Furthermore, the heat release rate (HRR) of fire is a stronger measure of fire intensity and hazard than total heat release.
Table 3 The energy capacity, PHRR, and mass for batteries of different scales and applications.
Battery & Fire
Energy, Eb [Wh]
PHRR [kW]
Mass [g]
Configuration
Single Battery Cell
11
20.9 [81]
95 [81]
pouch
10
9.1 [83]
cylinder
10
–
45 [84]
Cylinder 18650
8
1.9 [85]
cylinder
10
5.6 [86]
44.3 [86]
Cylinder 18650
5
8.3 [86]
40.2 [86]
Cylinder 18650
Portable Battery Module
112
54.8 [87]
1,228 [87]
pouch
124
–
639 [87]
–
107
–
734.8 [87]
–
92
28.5 [87]
–
pouch
124
57 [87]
–
pouch
185
49.4 [88]
1,675 [88]
prismatic
26
21[89]
–
pouch
259
145 [89]
–
pouch
800
70 [85]
–
cylinder
288
39 [85]
–
cylinder
72
14.3 [85]
–
cylinder
32
6.6 [85]
–
cylinder
16
3 [85]
–
cylinder
962
442.6 [84]
4,560 [84]
cylinder
185
37.8 [90]
900 [90]
prismatic
EV Battery
16,000
300 [65]
–
prismatic
12,000
1,515 [91]
–
–
18,000
1,651[91]
–
–
40,000
–
174,672 [55]
–
41,000
–
179,825 [56]
–
42,200
–
183,478 [57]
–
90,000
–
360,000 [57]
–
24,200
–
318,000 [92]
–
64,000
–
457,200 [93]
–
EV Fire (battery
+ plastics)
24,000
6,300 [94]
–
–
16,500
4,200 [94]
–
–
23,500
4,700 [95]
–
–
battery power station fire
500,000
2,555 [96]
–
–
1,000,000
5,070 [96]
–
–
2,000,000
10,150 [96]
–
–
3,000,000
15,220 [96]
–
–
4,000,000
20,290 [96]
–
–
5,000,000
25,370 [96]
–
–
Figure 7. PHHR of burning Li-ion batteries of different scales
The PHRR for EVs will be attained in full-scale EV fire testing after the LIB is also engaged in the fire (i.e., igniting)
, comparable to other fire occurrences. Table 4 summarizes key fire characteristics linked to burning ICEVs and EVs. The amount of fuel in the fuel tank, the capacity of the battery pack, and the amount of polymer material on-board can all affect the outcome. In general, evidence shows that electric cars, which typically have battery packs of 20-40 kWh for BEVs and 1-20 kWh for PHEVs [5], will offer a fire risk similar to that of conventional vehicles. Section 3.1 delves more into the regular full-scale EV and ICEV fire testing, as well as the evolution of HRR throughout time. However, it remains to be seen if this holds true for high-performance EVs and heavy EVs, which may have battery capacities of up to 100 kWh and 660 kWh, respectively, as compared to their ICEV counterparts.
Smoke and Toxicity: There is a significant risk of thermal runaway when the battery temperature surpasses roughly 150°C. When thermal runaway occurs, either the cell or the cell's safety valve will rupture, releasing hazardous gas. More battery cells will fail as thermal runaway spreads, resulting in more smoke and poisonous fumes. Hydrogen fluoride (HF), hydrogen cyanide (HCN), carbon monoxide (CO), and other poisonous gases are examples . When these gases are inhaled, they can cause dizziness, headaches, coma, loss of consciousness, and even death . Inside the LIB cell, fluorine can create phosphorous oxyfluoride (POF3), which is potentially more hazardous than HF. The following equations provide the reaction formulae for the generation of HF and POF3:
LiPF6 LiF +PF5 (4-1)
PF5 + H2O =POF3 + 2HF (4-2) LiPF6 + H2O LiF + POF3 + 2HF (4-3)
The chemistry and size of the LIB play a role in possible gas emissions, which range between EV manufactures and kinds. For LiFePO4 (LFP) cells, Sturk et al. discovered that gas emissions were sluggish and in small quantities . Although the total amount of HF released was identical, the concentration of HF in the released species was greater for LFP than for NMC/LMO cells that emitted a bigger gas volume in a shorter period. This suggests that the emission behaviour of an electric bus, which uses LFP more frequently than passenger cars, would differ from that of a burning passenger EV.
There is a scarcity of data on the quantity of harmful fumes generated in both ICEV and EV fires. As indicated in Table 5, the experiments undertaken by Lecocq et al. Provide some insight into this topic. The overall quantity of HF emitted by EVs is nearly twice that measured for the ICEVs under consideration . When the LIB begins to burn, which did not occur until 30 minutes after the fire in another portion of the car, the detected levels of HF grow. This contribution could be seen sooner if the fire started in the LIB. The danger of this emission, on the other hand, is highly dependent on the event situation. Outside, HF will most likely rise and rapidly disperse, but in enclosed places, if gases are not ventilated, this might be a problem. However, further large-scale tests are required to confirm this. It is also advised that harmful vapours and fumes from an EV fire be removed using a water spray jet. The quantities of acid gases such as HF, which are generated in ICEV, EV, and LIB fires, can be lowered by spraying water on them to wash them out [1] . Although the efficacy of water spray has yet to be determined, it is said that this strategy is currently in use for combating the effects of chemical fires, and so might give firefighters with a handy tool to help them deal with EV flames in the field.
Table 4. List of toxic gas emissions from full-scale EV fire tests .
Vehicle
Weight
Battery or fuel capacity
Total CO (kg)
Total HF (kg)
Unknown BEV
1122
16.5 kWh
10.4
1.5
Unknown ICEV
1128
Full tank of Diesel
12.0
0.6
Unknown BEV
1501
23.5 kWh
11.7
1.5
Unknown ICEV
1404
Full tank of Diesel
15.7
0.8
In comparison to fire tests on single battery cells and battery packs (e.g. [18,29,31,13]), there is still a scarcity of data on how an EV will behave in a fire. Full-scale fire experiments are expensive and sometimes constrained by trade secrets. Nonetheless, reliable predictions for their fire behaviour might be derived based on scientific understanding of small-scale LIB testing and the gathered database of EV fire events.
-
-
Lessons from past EV fire accidents involving battery
EV fires have received a lot of attention and have sparked a lot of debate in the media. Although the overall number of EV fires is still significantly lower than that of ICEV fires [5], this might be due to the fact that EVs have a far smaller worldwide market share than ICEVs. According to statistics, arson is responsible for over half of all ICE vehicle fires, and many more are started by other fires [1]. In Haarlem, Netherlands, in 2010, more than 26 automobiles were damaged in an underground parking garage with no sprinkler system. At least 1,400 automobiles were burned in a multi-story car park in Liverpool, UK, in a huge chain reaction fire in 2018, which may have been prevented.
Electric vehicle fires are predicted to follow the same pattern as ICEV fires. Unfortunately, there are currently no precise statistics on the distribution of various causes of EV accidents, such as arson, fires caused by other fires, traffic accidents, and self-ignition. It's worth noting that while there are many self-ignited fire mishaps involving ICEVs, the bulk of them involve older cars. The majority of self-ignited fire mishaps in both ICEV and EV vehicles begin in their power systems, namely the engine and battery, respectively. Because the majority of EVs in the world are still relatively young, and the number of EVs is still fast increasing, a statistical comparison of fire risk between ICEV and EV is not conceivable.
When an electric vehicle (EV) fire occurs, the battery is frequently cited as the major cause of the EV fire. However, there are a variety of additional circumstances that can contribute to this, including charging system failure, cable overload
, igniting of other combustible materials, and arson . The fires that do occur in electric vehicles are very new and typically complicated. They can, however, be classified into the following groups:
-
While parked, the EV catches fire (often referred to as spontaneous or self-ignition). Extreme weather conditions, such as low/high temperatures or excessive humidity, may be to blame. It's possible that it's linked to'spontaneous' internal cell breakdown. These failures are frequently tied to LIB misuse that occurred beyond of its safety window at some time throughout its existence.
-
An EV's LIB has been damaged due to a traffic collision or other forms of mistreatment. The battery pack is so badly damaged that the LIB ignites during or shortly after the collision. With the growing number of electric vehicles on the road, the likelihood of an EV getting involved in such an accident will undoubtedly increase.The LIB of an EV has been subjected to thermal abuse and reignites after the initial fire had been dealt with.
-
The LIB and EV are ignited by external factors which may include, arson, wildland fires or a burning structure in the vicinity of the vehicle.
Figure 8. Examples of temperature measurement plan for battery cells [136].
The breakdown of a battery can result in a variety of events, including venting, fire, or even explosion. The European Council for Automotive Research and Development (EUCAR) has identified these various dangers (see Table 5). The battery's reactions to abusive circumstances can be categorised according to the level of danger severity. An explosion is the most serious type of incident. Aside from misuse testing, several standards take into account the evaluation of chemical risks. The flammability and emissions of poisonous gases or smoke created during the thermal runaway process are the subject of the chemical hazard test. SAE J2464:2009, SAE J2929:2013, and UL 2580:2013 are some of the standards that give thorough information on quantifying and assessing the toxicity and flammability of Li-ion battery emissions. The composition of the observed emissions should be below specific concentrations.
Table 5. Hazard severity level and descriptions
Hazard level
Description
Classification criteria and effect
0
No effect
0
1
Passive protection activated
No damage or hazard; reversible loss of function. Replacement or re-setting of the protection device is sufficient to restore normal functionality.
2
Defect/Damage
No hazard but damage to RESS (rechargeable energy storage system); irreversible loss of function. Replacement or repair.
3
Minor leakage/venting
Evidence of cell leakage or venting with RESS weight loss <50% of electrolyte weight.
4
Major leakage/venting
Evidence of cell leakage or venting with RESS weight loss >50% of electrolyte weight.
5
Rupture
Loss of mechanical integrity of the RESS container, resulting in the release of contents. The kinetic energy of released material is not sufficient to cause physical damage external to the RESS,
6
Fire or flame
Ignition and sustained combustion of flammable gas or liquid (approximately more than one second), excluding sparks.
7
Explosion
Very fast release of energy sufficient to cause pressure waves and/or projectiles that may cause considerable structural and/or bodily damage, depending on the size of the RESS. The kinetic energy of flying debris from the RESS may be sufficient to cause damage as well.
Figure 9. NFPA emergency field guide for (a) FIAT 500e BEV, and (b) BMWi3 hybrid EV .
Every EV manufacturer is required to provide consumers with unique model-specific accident instructions, including fire emergency response. The National Fire Protection Association (NFPA) provides an emergency field guide for disabling various batteries for various vehicle brands and models (Figure 9). The safety information and procedures should be familiar to the vehicle operators. Manual service disconnects might stop the high-voltage battery without cutting or touching components directly, but bad conduct could lead to significant harm or death. The following are the recommended emergency protocols in the event of an EV fire. Customers should pull over, turn off the car, exit the vehicle, and move away from it before calling emergency services if they see sparks, smoke, or flames emanating from the vehicle. In-service information on the type of vehicle involved, such as BEV or HEV. People should be warned not to inhale smoke, vapours, or gas emitted by the burning car, since this might be dangerous. Keeping a safe distance upwind, and uphill if feasible, from the car fire is the best way to avoid this. Finally, while waiting for emergency responders, consumers should keep off the road and out of the way of any approaching vehicles.
The most recent NFPA Electric Vehicle Emergency Field Guide includes more thorough instructions for dealing with vehicle fire accidents including mainstream hybrid and electric cars from various manufacturers. There are two sections to the instruction. The initial processes section contains the methods for identifying, immobilising, and disabling the vehicle. The plan for fire suppression is the second phase. Hybrid and electric vehicle fires should be extinguished using proper vehicle firefighting tactics as suggested by the NFPA and in compliance with department SOPs (standard operating procedures).
The suggested EV firefighting method is stated as follows by combining different codes of practise from various nations.
-
Locate the vehicle. Fire rescue centres in various European nations can request information based on a vehicle's licence plates. This can assist firemen in:
-
-
determining the right rescue datasheet;
-
determining the firefighting plan depending on the scenario;
-
protecting persons first;
-
controlling or extinguishing the fire, and if the automobile is charging, switching off the charging infrastructure if feasible.
-
After the fire has been extinguished, the vehicle should not be moved; (6) The final stage is on-site cleaning. Certain disposal methods are also advised following a fire disaster, such as parking the EV outside after the event because of the re- ignition ability of the battery.
More public parking lots are beginning to install EV charging stations in order to attract EV users and demonstrate their commitment to sustainability as the number of electric cars (EVs) climbs year after year. There are a variety of concerns regarding this, and several cities have prohibited charging in parking garages. Parking lot structures represent a high fire risk due to the volume and density of autos they may store (Figure 10). Their low ceilings help fire spread by reflecting heat down to the fuel load, while poor ventilation causes deadly gas accumulation. Parking lots are designed to handle a large number of cars burning at the same time. As long as the fire does not extend beyond 3-4 autos, there will be no structural collapse. Fire suppression systems can assist to reduce the risk of a fire by keeping it from spreading. Traditional sprinklers and other fire suppression equipment can assist to reduce the threat of a fire. However, the chemical harm that burning automobiles, particularly electric vehicles, pose to structures should be properly assessed.
Figure 10. (a) Park Garage with GRID bot charging stations and (b) hundreds of new EVs parked in a public area.
CONCLUSION
This study examines recent battery fires in electric vehicles (EVs), as well as related fire safety concerns and mitigation techniques. Because of high demands in driving performance and charging speed, unavoidable traffic accidents, and the rising scale and energy density of battery packs, the fire danger and hazard of Li-ion battery (LIB) is particularly important in EV. In order to offer a qualitative knowledge of the danger and hazard of EV fire, many typical fire accidents in battery EVs, hybrid EVs, and electric buses are studied. As the market share of electric vehicles grows over the next few decades, a rise in EV fire incidents is projected. Because of this, there have only been a few full-scale EV fire experiments thus far.
Because of the risk of battery re-ignition and the difficulties of cooling the battery pack within, EV fires are more difficult to put out. Water is still the most effective way to put out an electric vehicle fire, and a lot of it is needed to extinguish and cool the battery. When sprayed directly to the battery pack, however, fewer suppressors is required. Furthermore, nothing is known about the fire risk presented by discarded EVs and battery packs. In the future, better-designed fire-protection systems should be required for buildings and parking areas with a higher number of EVs and charging stations. The goal of this review is to assist researchers and businesses working with batteries, electric vehicles, and fire safety.
REFERENCES
y 2014.
[1] Matulka R. The History of the Electric Car. Department of Energhttps://www.energy.gov/articles/history-electric-car.
[2] Anderson CD, Anderson J. Electric and Hybrid Cars. second. McFarland & Company; 2010. [3] Grauers A, Sarasini S, Karlström M, Industriteknik C. Why electromobility and what is it? In: Sandén B, editor. Systems Perspectives on Electromobility, Chalmers University of Technology, Göteborg; 2013. [4] BP. Statistical Review of World Energy 2018 2018:153. [5] Bisschop R, Willstrand O, Amon F, Rosengren M. Fire Safety of Lithium-Ion Batteries in Road Vehicles. BorÃ¥s: 2019. [6] Bisschop R, Willstrand O, Rosengren M. Handling Lithium-Ion Batteries in Electric Vehicles – Preventing and Recovering from Hazardous Events. 1st International Symposium on Lithium Battery Fire Safety, Hefei, China: 2019. [7] National Transportation Safety Board. Preliminary Report: Crash and Post-crash Fire of Electric-powered Passenger Vehicle 2018. [8] CGTN. Tesla car catches fire in China, investigation underway 2019.https://news.cgtn.com/news/3d3d514d7a416a4d34457a6333566d54/index.html.
[9] Bangkok Post. Porsche catches fire while charging 2018. https://www.bangkokpost.com/thailand/general/1429518/porsche- catches-fire-while-charging. [10] Loveday S. BMW i3 REx Burns After Catching Fire While Parked In Spain. INSIDEEVs 2018. [11] Zhou X. Frequent Fire Accidents on Electric Vehicle. Operators 2018;10:656. [12] National Transportation Safety Board. Preliminary Report: Highway HWY18FH013. National Transportation Safety Board 2018. [13] Revill J. Tesla crash may have triggered battery fire: Swiss firefighters 2018. [14] National transportation safety board. Preliminary Report – Battery Fire in Electric-powered Passenger Car. National Transportation Safety Board 2018. https://www.ntsb.gov/investigations/accidentreports/pages/hwy18fh014-preliminary.aspx. [15] Deick M Van. Facebook 2018. https://www.facebook.com/Marco.vandeick/posts/344761026325031. [16] Gutman M, S. Yuon. Firefighters work 16 hours to put out fires in Tesla Model S. ABC News 2018. https://abcnews.go.com/Technology/tesla- opens-investigation-car-burst-flames-times/story?id=59930420. [17] Wang Q, Ping P, Zhao X, Chu G, Sun J, Chen C. Thermal runaway caused fire and explosion of lithium ion battery. Journal of Power Sources 2012;208:21024. doi:10.1016/j.jpowsour.2012.02.038. [18] Wang Q, Mao B, Stoliarov SI, Sun J. A review of lithium ion battery failure mechanisms and fire prevention strategies. Progress in Energy and Combustion Science 2019;73:95131. doi:10.1016/j.pecs.2019.03.002. [19] Feng X, Ouyang M, Liu X, Lu L, Xia Y, He X. Thermal runaway mechanism of lithium ion battery for electric vehicles: A review. Energy Storage Materials 2018;10:24667. doi:10.1016/j.ensm.2017.05.013. [20] Ouyang D, Chen M, Huang Q, Weng J, Wang Z, Wang J. A Review on the thermal hazards of the lithium-ion battery and the corresponding countermeasures. Applied Sciences 2019;9:2483. doi:10.3390/app9122483. [21] Evarts EC. Lithium batteries: To the limits of lithium. Nature 2015;526:S935. doi:10.1038/526S93a. [22] Moon G. Renault-Samsungs Electric Vehicle Catches Fire Due to Ignition from Bonnet. ETRC·KGTLAB 2016. http://www.ipnomics.net/?p=14858. [23] Pecht M. Safety. CALCE Battery Research Group 2015. https://web.calce.umd.edu/batteries/safety.html. [24] Home of EV. What should we do during the EV fire? SOHU 2018.https://www.sohu.com/a/233521985_526255.
[25] Hertzke P, Müller N, Schenk S, Wu T. The global electric-vehicle market is amped upand on the rise. McKinsey Center for Future Mobility 2018. [26] Offer GJ. Automated vehicles and electrification of transport. Energy & Environmental Science 2015;8:26 30. doi:10.1039/C4EE02229G. [27] Frost & Sullivan. Global Electric Vehicle Market Outlook, 2018. 2018. [28] Egbue O, Long S. Barriers to widespread adoption of electric vehicles: An analysis of consumer attitudes and perceptions. Energy Policy 2012;48:71729. doi:10.1016/j.enpol.2012.06.009. [29] International Energy Agency. Global EV Outlook 2018: Towards cross-modal electrification. IEA Publications 2018. doi:10.1787/9789264302365-en. [30] The Economist Intelligence Unit. France ranked top for EV adoption in 2017. The Economist 2018. [31] Bjerkan KY, Nørbech TE, Nordtømme ME. Incentives for promoting Battery Electric Vehicle (BEV) adoption in Norway.Transportation Research Part D 2016;43:16980. doi:10.1016/j.trd.2015.12.002.
[32] U.S. Commercial Service. The Electric Vehicle Market – France 2017. https://www.export.gov/article?id=E- Mobility-in-France. [33] Germany Trade & Invest. Electromobility in Germany: Vision 2020 and Beyond 2015. [34] Lu J. Comparing U.S. and Chinese Electric Vehicle Policies. Environmental and Energy Study Institute 2018. https://www.eesi.org/articles/view/comparing-u.s.-and-chinese-electric-vehicle-policies.