
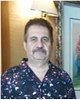
- Open Access
- Authors : Riyadh R. Yassin, Samsudin Haji Taib
- Paper ID : IJERTV9IS020277
- Volume & Issue : Volume 09, Issue 02 (February 2020)
- Published (First Online): 07-03-2020
- ISSN (Online) : 2278-0181
- Publisher Name : IJERT
- License:
This work is licensed under a Creative Commons Attribution 4.0 International License
Geo- Environmental Research Study by Application of Integrated Techniques to Evaluating, Determining and Monitoring the Geo-Hazard of Karst Features and its Deposits in Selected Sites Situated Over Carbonate Karst Environment in Kuala Lumpur and Selangor, Peninsular Malaysia
Riyadh R. Yassin1, Samsudin Haji Taib2
1,2 Department of Geology, Faculty of Science, University of Malaya,
50603 Kuala Lumpur, Malaysia
Abstract:- This geo – Environmental research study focuses on due to irregular distribution of pinnacles and gutters in these evaluating, determining and monitoring the potential geo- chosen karst environments region. Under data analysis could hazard of various classes of carbonate karst features and its affecting the accuracy of integrity and reliability of the deposits, extend in the surface and subsurface of selected ex- technique.
mining sites that situated over covered carbonate karst An ERT with 05 m electrode spacing will provide a environment in Kuala Lumpur and Selangor, West Malaysia, sufficient resolution that will allow pinpointing the exact via the integration of geological, geophysical, structural, locations of the in-filled Buried sinkholes, cavities, channels satellite imaging and environmental techniques. and other geophysical anomalies. The interpretation of the Several sites selected for this study, located in Kajang, resistivity data indicated that the reactivated sinkholes have Bangi and Sentul not mentioned in this study due to badly- caused due to present of large cavities, and most of the soil in behaved in the instrument reading and keep on the other sites these sinkholes appears to have flowed into the subsurface via as will mentioned. The first selected site is situate next to a solution-widened system of joints or fractures. Many Jalan Universiti, Tasik University Malaya, Kuala Lumpur, sinkholes and these cavities have detected underneath the while the second selected site is located at Parlimen Street, surface in the ER Tomography sections in the chosen sites, also in Kuala Lumpur. The third selected site is located in proving the fact that all of these cavities are pre-existing Batu Caves, Selangor, and finally, the fourth selected site is features that rapidly filled with clay, and the only reason they
located in Petaling Jaya, Selangor. have recently detected were due to collapses or subsidence.
The subsurface karst feature is one of the main Samples from 20 borings holes in the designated sites characteristics in the development process of karst areas, have collected using a hand auger having a maximum length especially when these features contain sediments such as clay, of 12 m for analysis and auger machine until depth between sand and mineralized materials. This study concentrates in 20-22m. The borings was conducting in an unconsolidated the development of karst features in these selected sites. material over a karstified limestone environment. The soil Several subsurface karst features were identifying in these borings have used to define certain karst features, small sites during the utilization of a high-resolution geophysical cavities and a few secondary processes spatial deposits. The technique. depths of the boring holes were approximately 02m, 05m, 10m
The Electrical Resistivity Tomography (ERT) was and 11m.
employed in order to realize the research objectives, image the Cassiterite minerals (Tin-bearing alluvium) found subsurface karst features across several sites as a main stage, accumulated in rich deposits of clastic sediments, silty sand understand the origin of these karstic features, recognize their with a small amount of clay and covered by a layer of sand, deposits, and finally, to estimate its depth. The tomography and gravelly sand. The Tin is dark in color, with a grain size data from different profiles has interpreting with using of the of less than 300 µm. It also contains an abundance of elements extracted boring samples by auger in order to improve the that are characteristic of specific granites and other possible results of (ERT). The resistivity data from the multi-electrode passageway finder elements such as, Bi, B, Cu, Pb, and Zn. measurements collected from several profiles have plotted in These mineral deposits were present in soil pipes and the the tomography sections. fractures at shallow depths. These deposits have probably The resistivity maps imaged many features in the trapped by the buried irregular surface of a carbonate karst
anomaly of different shapes. The interpretation of the environment.
resistivity data has used to generate the geological model of The clay-filled fractures and caves, or small cavities, the selected sites, which consists of karstified limestone unit of have represented by zones of high-conductivity and low bedrock from the geophysical survey, with varying depths resistivity in the Wenner ERT sections. Furthermore, the soil relative to the ground surface. The geological variation was pipes are associated with zones containing low-medium
resistivity in the Wenner ERT sections. All of these karst features are above the water table, so the low resistivites may be due to the infilling by clay, soil, high conductivity minerals deposits, or pockets of perched meteoric water within the buried sinkholes or pipes. In addition, radon gas detected within this water in some places gives reading of (01-03) Ohm- m.
The structural study at Selangor and Kuala Lumpur via the utilization of (Google terrain satellite image) and (Radar sat image map) shows several sets of lineaments representing (fractures and Faults), originating from many directions, which are NW-SE, N-S, NE-SW, WNW-ESE and ENE-WSW, while very few were found in the E-W direction.
Furthermore, this study discovers that most of drainage patterns that have created along each fold outcropping within the study area have dictated through the direction of the fractures and faults. Due to Orogeny movement during earlier periods, which affected the study area and lead to deep erosion processes took place in these orientations which reflecting a strong linkage between the orientation of the lineaments and drainage patterns. Consequently, this research proved an effective relationship between the karst in Kuala Lumpur limestone and the visible surface of drainage patterns. The isolated areas of high resistivity adjacent to the surface might be cause by concentrations of sandy gravels,
residual chert, iron oxide nodules or limestone fragments.
Moreover, various amounts of overlying sand have detected in many locations on the surface at the selected sites, which are mostly characterized by high resistivity interpreted as dry and friable sand due to ex-mining excavating operations, extracting from the pits of tin mine adjacent to those sites. In addition, some subsidence area over the buried sinkholes in some sites in-filled with rock fragments and friable sand had done through engineering remediation techniques.
This current study of the field model demonstrates that the Electrical Resistivity Imaging technique is a useful geophysical tool that can be used to image bedrocks and characterize the bedrocks surface and its features in the subsurface karst terrain for the purpose of automated data collection and imaging inversion processing.
This study clarified that the marbleized limestone bed rock in site 1 (Tasik university Malaya) and site 4 (Petaling Jaya) is deep, only rock head pinnacles what observed in ER Tomography sections in both sites. Besides, this study clarified that the marbleized limestone bed rock in site 2 (Jalan Parlimen) and ste 3 (Batu cave) is shallow and contain numerous combined cave systems and cavities what observed in ER Tomography sections of both sites.
The E. R. Tomography technique is being limited to the depth of 28.6 m. This limitation severely limits our attempt to understand the activities of the underground combined cave systems it extensions. Moreover, the existence of cavities that discovers in these sites.
In accordance to geo-technical table for engineering classification of karst ground conditions, to classify the karst in semi wet and wet tropical region that presented by (Yassin,
- R. & Haji, Taib, S. ,2012). The karst level in site#1 (Tasik University Malaya) observed in the ERT sections between profile # 1 and profile# 4 is an mature karst type KaIII. Due to presence of deep Gutter or channel, extend between pinnacles from profile#1 to profile#4. Furthermore, mature buried sinkholes observed in the sections of profiles #2 & #3. The karsts level in site #2, Jalan Parlimen, observed in the ERT sections between profile # 1 and profile #2 is a complex karst KaIV due to presence mature buried sinkholes, cavitiesand relief pinnacles. Moreover, observed in the ERT sections between profile # 3 and profile #5 deep Gutter and caves.
Furthermore, the karsts level in site#3( Batu Cave) observed in the ERT sections between profiles #1 and profile
#6 is a complex karst type KaIV. Due to the presence of underground channel fill with sediments, buried sinkholes extend between pinnacles from. Finally, the karst level in site#4 (Petaling Jaya) observed in the ERT sections between profile #1 and profile #4 is an mature karst type KaIII. Due to the presence of many pinnacles and various type of buried sinkholes. The karst level found in the study area between, type mature karst type KaIII and complex karst type KaIV.
The Electrical Resistivity Imaging technique is also able to clarify the subsurface geological information for geological and engineering construction projects in order to assist decision-making on any requirement for soil improvement in these karstic regions. In addition, this study had shown an impression of surface and subsurface remediation process that can use with the integrating techniques in site characterization. The implementation of these processes and its strategies which resulting in monitoring, operation, maintenance, and the costs that can affect the effectiveness, timing and cost of remedial solutions.
Furthermore, in the construction of the housing structure requirement to development a technique for remove of radon gas from out- door and in-door air streaming and other contaminants that affected the breathing and present in the air within the buildings and housing complex in these karst regions.
Key Words: Geo – Environmental, Integrated techniques, Evaluate, Geo-hazard, Karst features, Kuala Lumpur and Selangor- Peninsular Malaysia
- INTRODUCTIONAccording to the established definition, karst is generally a terrain underlain by limestone, dolomite, dolostone and marbleized limestone, where the topography is primarily formed by the dissolving rocks. A karst terrain is often recognizable by a wide range of closed surface depressions, well-developed subterranean drainage, sinkholes, sinking streams, springs, caves and noticeable interactions between the circulation of surface and groundwater. Gutters are features that are separating by upward-protruding limestone, known as pinnacles. Carbonate rocks contain various amounts of insoluble materials.
However, when the soluble constituents had dissolved and removed by groundwater, the insoluble materials had separated and discarded. Insoluble materials consist of sedimentary gravel, sand, silt, and clay-deposited carbonates. Chert and flint are also present in these deposits. Iron oxides had precipitated from water, which weathers iron minerals in the original sediments. These insoluble residues accumulate at the sides of the pinnacles and the bottoms of the gutters. These accumulations may lead to the filling of the gutters, which will in turn blanket the rock surface.
Allochthones sediments such as volcanic ash, alluvial sediment, or marine sediment might also mantle the bedrock. Regardless of their origin, the sediments shield the limestone bedrock, preventing any solution- related features to form in the underlying bedrock.
Irregularly distributed gutters and pinnacles significantly affect land development. The pinnacles contribute to differential subsidence of the ground surface when combined with other factors such as the gutters, will form a potential area for sinkhole development (Benson et al., 1998).
On the other hand, defining the depth-to-bedrock by applying the boring data is time-consuming, expensive and inaccurate, because the data is just insufficient. Residual soils had produced because of mechanical and chemical breakdown of rocks from the ground surface downward, and the boundary between the underlying rocks and overburden soil. The type of soil is distinguished by sharp contrasts in imaging (Sowers, 1996), as the overburden soil is fundamentally different from the underlying carbonate rock. This contrast provides the basis for the geophysical technique that describes the bedrock surface in covered karst environments.
Several sites selected for this study, The first selected site is situate next to Jalan Universiti, Tasek University Malaya, Kuala Lumpur, while the second selected site is located at Parliament Street, also in Kuala Lumpur. The third selected site is located in Batu Caves, Selangor, and finally, the fourth selected site is located in Petaling Jaya, Selangor. The satellite images viewing the location of study-selected sites in Kuala Lumpur and Selangor, Peninsular Malaysia represents in figure 1.
Forty-one steel electrode arrays have employed in these surveys. The space between the electrodes was 05 m, while the lengths of the electrical survey lines were 200m. Both measurements depend on the size of the area and the placement of the wire arrays. The space between the two lines ranged from 25m at one site to 30m at another.
The resistivity data were acquired in order to image and characterize the shallow subsurface adjacent to the invisible and unidentified karst features at these sites.
An ERT with 05 m electrode spacing provided the sufficient resolution to pinpoint the locations of filled sinkholes and other geophysical anomalies, and identified an area of ongoing subsurface erosion that might collapse in the near future. This method has normally applied to near surface investigations as non-destructive technique.
The resolution and validity of the inverted model mainly depends on the geometry of electrode arrays that used, and the signal-to-noise Ratio. Several authors have studied the comparison between various electrode configurations for near surface studies respective to speed of field completing, signal/noise ratio, lateral positioning of the target, penetration and accuracy in depth determination. Most of the engineers misunderstanding that how the sinkholes created, even some of the geologists, its need them to understand that this processes is continues, may be started before 10th of years and still active till now a day.
Figure 1: Google Terrain, Google Normal and Satellite image map viewing the location of study-selected sites in Kuala Lumpur and Selangor, Peninsular
Malaysia
The sinkhole created in un- consolidated materials in cover layers of limestone, dolomitic or marbleized bedrocks, with thickness more than 15.0m. Then they discharge or emptying there capacity through cavities and channels in the limestone bedrocks. This research show that many sinkholes created over the limestone bedrocks in Kuala Lumpur and Selangor in the un-consolidated material even when the marbleized limestone rock deep. Besides, this study display that the thickness of upper layers is the mostly important in the processes of sinkholes creation.
These study also comprising the problems faced the civil engineers and geo-engineers when constructing in carbonate karst environments, due to settlement of footing, the length of conventional piles, the bored piles, sinkholes problems and the effect of earthquakes and heavy rains in creating the sinkholes, voids, caves and cavities.
- OBJECTIVE OF THE STUDY(ERT) Geo-technical investigation technique has integrated with other techniques and engineering subsurface remediation techniques at the fundamental sites in order to realize the following objectives:
- Site characterization through applying of integrated techniques.
- Imaging the subsurface to detect evidence for near surface karst features such as (sinkholes, cave, cavities, channels and voids).
- Pinpoint if any mineral-air-water in filled karstic features are present at the subsurface.
- Estimate the depth, shape, variety, and understand the origin of these surface karstic features.
- To detect, determine sinkholes and overburden thickness in these covered carbonate karst terrains; and to define probable eventually collapse area in the near future to set the planning solution for it.
- Estimate the depth of the original bedrock (marbleized limestone), and reproduce the geological model representing the studied regions.
- Determine the type of sediments and the subsurface layering, including its depth and thickness.
- Determining the presence of subsurface features from various natures in the overburden layers, that overcomes the doubts and difficulties in driving of conventional, jack -in and bored piling.
- Recognize the effect of the structural elements influencing the development of subsurface karst in the study area.
- Identify and classify the level of karst ground conditions due to presences of the karst featuresin the subsurface that might compromise the integrity of any proposed future work in these sites.
- Assess the construction sites and evaluate the subsurface karst formation level and its extent, and identify the problematic zones of a developed limestone area that might result in a potentially dangerous collapse or ground failures, which might superimpose these features in near future.
- The best surface and subsurface geotechnical remediation technique can use with the integrated techniques in site characterization and hazard mitigation.
- LOCATION OF THE STUDY AREAThe selected sites are approximately the crux of Peninsular Malaysia. 150 years ago, Cassiterite (SnO2) had discovered in the region on the alluvium overlying bedrock (Yeap; 1985; 1987). Generally, speaking, the study area is located in the broad valley of Klang River, consisting of a flat alluvial plain and bounded by hills; predominantly made up of granitic rock to its west and east.
The primary study region is located in Kuala Lumpur, situated at the West Coast of Peninsular Malaysia. It is the capital and largest city of Malaysia, and its location coordinates are 3°8 0 N and10°1420 E. This state includes two sites, namely:
The first selected study site, located at Jalan University, Tasik University Malaya, its coordinate latitude N 3° 07′ 05.63″ – N 3° 07′ 16.73″, and longitude, E 101°
39′ 29.91″ – E 101° 39′ 38.29″ , as shown in Fig. 2.
The second selected study site, located at Jalan Parlimen; is a major road in Kuala Lumpur; its coordinate approximately latitude N 3°09′ 01.74″ – N 3°09′ 05.68″, and longitude E 101°41’16.87″ – E 101°41’25.59″, as shown in Fig. 3.
Figure 2: Satellite and photograph Images viewing the location of the selected study site no.1 in Jalan Universiti, Tasik University Malaya, Kuala Lumpur
Figure3: Satellite and photograph Images viewing the location of the selected study site no.2 in Jalan Parlimen -Kuala Lumpur
The second studied region is located at a state called Selangor. Selangor is on the west coast of Peninsular Malaysia, bordered by Perak to the north, Pahang to the east, Negri Sembilan to the south, and the Straits of Malacca to the west. It surrounds the federal territories of Kuala Lumpur and Putrajaya. Its capital is Shah Alam, and its coordinates are 3° 20’0″ N, 101° 30’0″ E.
Selangor includes two study sites (site #3 & site #4), namely:
The third selected study site is located at a place called Batu Caves, consisting of a limestone hill, which consist of a series of caves and cave temples located in the Gombak district; its coordinate 3° 14’4.13″ N- 3° 15 2.88 N and 101° 41 2.55 E -101° 41 8.18 E. The
study area is situating to the north west of the limestone hills, approximately at latitude N 3°15′ 2.88″ N 3°14’49.92″, longitude E101°40’55.2″ E101°41′ 8.16, as
shown in Fig. 4.
Figure 4: Satellite and photograph Images viewing the location of selected study site no.3 in Batu cave- Selangor
The fourth selected study site is Asia Jaya, located in Petaling Jaya. The site is adjacent to a major federal highway linking Kuala Lumpur and Klang, Selangor. Its exact location is northwest of the limestone hills,
approximately at latitude N 3°06′ 18″ N 3°06′ 28.10″, and longitude E101°38′ 27.36″ E101°38′ 42″, as shown in Fig. 5.
Figure 5: Satellite and photograph Images viewing the location of site no.4 in Petaling Jaya Selangor
- GEOLOGY OF THE STUDY AREA
-
- General GeologyThe rock-hard geology of the area consists of sedimentary rocks, ranging in age from Middle-Upper Silurian to Mesozoic or younger. At the bottom of this sequence are the oldest strata; the Hawthornden Formation, and the Kuala Lumpur Limestone Formation, while above it, is both from the Middle-Upper Silurian (Gobbett, 1964). This Lower Paleozoic formation experienced its first phase of folding during the Devonian age, followed by an extensive period of weathering and erosion. During the Permo-Carboniferous period, the Kenny Hill Formation had deposited upon the eroded surface of the Kuala Lumpur Limestone Formation to form an angular unconformity.
The second phase of folding followed soon after, perhaps during the Jurassic Age, which results in further deformation of the Lower Paleozoic rocks. It can see that in the Lower Paleozoic sequence, the bedding dips are commonly steep and overturned; differing from the gentler dips of the Kenny Hill Formation, which overlays them. Granites, estimated to be either younger or broadly contemporaneous with the second phase of folding, then intruded the geological sequence. The last period of deformation is NE-SW and NW-SE trending faulting, which has affected all the formations and the granitic outcrop (Stauffer, 1968). The faulting has inadvertently displaced the Kuala Lumpur Limestone Formation.
- Limestone of Kuala Lumpur and SelangorGenerally, the surface of limestone in the Peninsular Malaysia comes in different features and shapes, depending upon the differential rate of chemical erosion, or as a result of rainfall and direction of streams flows. Karst of tropical areas usually possesses extraordinary vertical hills with hemispheroidal shape. Its development span from a cone to tower karst, documented as an individual landscape for many years, such as karst hills (Jennings, 1985).
Limestone hills are characterizing by its steep-sides with sub-vertical to suspended cliffs. The base of limestone hills also frequently exhibit deep horizontal scratches or undercuts, which is due to its dissolution by streams, groundwater and swamp water.
Limestone rocks are extending to the subsurface along the western belt of Peninsular Malaysia to include the Langkawi Islands, South of the northern state of Perlis and Kedah, Kinta Valley of Perak, Kuala Lumpur, and Selangor.
Acording to scientists, Peninsular Malaysias limestone may have originated from various geological periods between the Silurian/ Ordovician times, which are almost 505 to 410 million years ago, and the Triassic period; which are 245 to 208 million years ago. Photographs viewing several of Limestone hills in Kuala Lumpur and Selangor in figure 6.
Figure6: Photographs viewing several of Limestone hills in Kuala Lumpur and Selangor
Many of the limestone formations in Malaysia have actually metamorphosed into marbles; a metamorphic rock consisting mainly of fine to coarse-grained recrystallized calcite and dolomite. Due to recrystallization, the crystals contain interlocking or sutured boundaries forming a mosaic, hence increasing the density and strength of the rock. For example, the marble shows the same limestone of wide occurrence in Peninsular Malaysia, with the major occurrences in the Klang Valley (Kuala Lumpur). In these areas, the limestone occurs as cliffs, as well as widespread bedrock formations.
The Kuala Lumpur area shows that the Limestone Formation dominates the majority of the area. The limestone has estimate to be about 236.827 km2 of Kuala Lumpur, or approximately 40% is underlining with distinctive limestone rocks, with a thickness of about 1850 m overlying graphitic schist, known as Hawthornden Schist. At the top of the sequence is Kenny Hills formation, which occupies the heartland of Kuala Lumpur, including areas at KLCC and Bukit Bintang.
According to (Gobbet & Hutchison, 1973), Kuala Lumpurs Limestone is Upper Silurian marble, finely crystalline grey to cream, thickly bedded, variably dolomitic rock. Banded marble, sac choroidal dolomite, and pure calcitic limestone also formed and are present. It dolomitize as a result of chemical thermal metamorphism and replacement of minerals, on top of recrystallization as a result of thermal solutions of Silica (SiO2), forming marbles consisting of fine and coarse crystals. The chert,
clay minerals, iron ore and peat or organic materials are the most common pollution in the Kuala Lumpur limestone.
Geologically, the outer belt contact of limestone/granite more thermally metamorphosed into marble, compared to its inner counterpart, via granite intrusion. Originally, the flat limestone plateau has deeply dissected by dissolution (Chng, 1984). Regional geology map of Malaysia viewing the bedrock geology of Kuala Lumpur and Selangor present in figure -7.
The surface of Kuala Lumpurs limestone has cropped out at Batu Caves, as it forms the only natural exposure at the north of Kuala Lumpur area, rising to a height of about 213 m above its surrounding areas. Otherwise, the exposures of limestone have only found in open cast hydraulic tin mines. During the process of mining, the overlying alluvium, which measured from 2.5 to 14 m thick, had removed.
The limestone underlies a low-lying plain of Kuala Lumpur, narrowing to the south, where its in turn in overlain by Kenny Hills formation, and further south near Serdang by Kajangs formation. It is composed of memorized limestone, with little amounts of interblended schist and phylite (metamorphic rocks) infrequently changed at the south near Sungai. It is crystalline in nature and grey in color; although white pure limestone is not uncommon, and contains some impurities, such as clay. This dolomitic limestone varies from one place to another, with a general increase of MgCO3 towards the south, based on the distance from granitic intrusions.
Figure7: Regional geology map viewing the bedrock geology of Kuala Lumpur and Selangor
In Klang Valley, i.e. Kuala Lumpur and parts of Selangor, the Lower Palaeozoic sediments are the almost entirely surrounded by Mesozoic tin-bearing granites. The limestone and the dolomite, which form the upper part of the succession, underlie an extensive alluvial flat, on which the local tin mining activities were concentrated after the pit was flooded or filled in. The Kuala Lumpur limestone had estimated to be 6000 feet thick, and contain fossils from the Middle or Upper Silurian age (Gobbett, 1963).
- Karst in Kuala LumpurFractures, joints and bedding plain are natural passageways for waste groundwater and acidic rain. The weathering and chemical dissolution in fractured limestone has found to be more aggressive than massive limestone (Bannister, Arbor, 1980); (Ericson, Migon, Olvmo, 2004); (Waltham; Bell; Culshaw, 2005). These fracture-patterns in limestone could be the reason for underground streams and multi-elevation cavities formation (Sowers, 1975); (Waltham, A. C. & Fookes, P. G., 2003).
Karst features had developed from a self-accelerating process of water-flow along well-defined pathways such as bedding planes, joints and faults. As the water percolates downward due to gravity, it dissolves and enlarges the pathways. Enlargement of a pathway allows more water flow, which increases the dissolution rate. As the enlarged pathway transmits more water, it pillages drainage from the surrounding rock mass. Over time, this process results in a very jagged appearance, sometimes dissecting vertically and deeply into the rocky terrains. It continues until it reaches the water table, below which all pore space had occupied by water.
Since the rocks had saturated with water, the water flow, and by association, the dissolution rate, invariably slows. The water table fluctuates as a result of seasonal change, and creates a zone of preferential dissolution along the zone of fluctuation. Over time, this process creates solution channels. Limestone usually forms at shallow
depths of a few meters, to less than 25m, except in areas covered by the thick Kenny Hill formation, where it might be as deep as 200m, such as those at the Petronas Twin Towers (Azam et. al. 1996). It is worth noting that cliff-like drops in the limestones profile were spotted at the sites of Petronas Twin Towers (Azam et. al. 1996), Pan Pacific Hotel (Mitchell, 1986), and Bistari Condominium (Hewitt &Gue ,1996).
The differences in the bedrock levels vary from 70m to more than 100m within these sites, which makes the presence of cavities in limestone highly unlikely. They form a part of the solution channel system, and almost all of the dimensional cavities encountered by boreholes is greater than 3m, analogous to (Tings, 1986) observations.
Cavities have described as partially filled, slimy and possess low N values (Standard Penetration Tests (SPT)). Large forms of solution, especially in dense nonporous limestone is thick-bedded and well jointed (Gobbett, 1965). Soft porous and poorly jointed limestone does not provide a favorable environment, due to the fact that; the groundwater flow is not concentrated into particular channels.
The highly irregular topography of limestone rock head in the Kuala Lumpur region was first discovered in opencast tin mines around 150 years ago; deep borehole records from construction sites in the city confirm its widespread occurrence (Tan, 1986a;1986b;1987). The rock head karst identified to have developed during the Quaternary period, although it is possible that a considerable amount of dissolution had occurred prior to the deposition of the Permo-Carboniferous Kenny Hill Formation; this paleo landscape has buried by alluvium to form Kuala Lumpurs current landscape of Kuala Lumpur (Chan & Hong, 1985).
(Paton, 1964) believed that the presence of mature karstic features in this area is the result of the climate; Kuala Lumpur receives approximately 2400mm of rain per year, at a temperature of around 26.5°C, which imparts much CO2 to the groundwater of these humid areas. The effects of dissolution processes due to the effects of several directions of the structural geology features that represented in (fractures & joints) urbanized over limestone rock in Kuala Lumpur and Selangor present in figure 8.
The construction of high-rise buildings on a flat alluvial plain that conceals the highly irregular topography
of limestone bedock has always been a challenge for engineers practicing in Kuala Lumpur (Ibrahim & Fang 1985); (Tan &Komoo, 1990). A large number of geotechnical problems have occurred during the construction of the previous major engineering projects on the limestone formation, some example of cases include the construction of the Petronas Twin Tower (Tan, 1996);(Pollalis, 2002), the Berjaya Times Square Complex (Gue& Tan, 2001), the Pan Pacific Hotel (Mitchell, 1985) and the Sri-Mara Complex (Tan, 1985);(Ting &Toh, 1985).
Figure 8: Effects of dissolution processes due to several directions of the structural geology features represent in fractures and joints urbanized over limestone rock in Kuala Lumpur and Selangor
- General GeologyThe rock-hard geology of the area consists of sedimentary rocks, ranging in age from Middle-Upper Silurian to Mesozoic or younger. At the bottom of this sequence are the oldest strata; the Hawthornden Formation, and the Kuala Lumpur Limestone Formation, while above it, is both from the Middle-Upper Silurian (Gobbett, 1964). This Lower Paleozoic formation experienced its first phase of folding during the Devonian age, followed by an extensive period of weathering and erosion. During the Permo-Carboniferous period, the Kenny Hill Formation had deposited upon the eroded surface of the Kuala Lumpur Limestone Formation to form an angular unconformity.
-
- THE POTENTIAL TIN MINERALS AND OTHERS ENVIRONMENTAL CONSIDERATIONSLimestone is a soluble rock, even in slightly acidic water, such as carbonic acid formed from the dissolution of carbon dioxide in water. The end result of the solution of limestone are a host of karst features, such as cavities, caves, solution slots or holes, pinnacle bedrock, stalactites/stalagmites, basal scrapes, overhang and outcropping in limestone cliffs. In addition, residual soils left over in the dissolution process of limestone. This residual red soil is name (terra rossa), which covers the limestone bedrock. These are accumulations of the insoluble residues or impurities of the limestone, comprising silica, organic matter, clays, and iron oxides.
Cassiterite minerals (Tin-bearing alluvium) are accumulating in rich deposits of clastic sediments, sand, silty with some clay and cover with a layer of sand and sand with gravel. The tin is dark in colour, with a grain size of less than 300 µm. It also contain an abundance of elements that are characteristic of specific granites and other possible passageway finder elements such as; As , Bi, B, Cu, Pb, and Zn. These mineral deposits are present in soil pipes and fractures at shallow depths. These deposits
had probably trapped by the buried irregular surface of carbonate karst environment. Previous study found that Cassiterite minerals in Kuala Lumpur and Selangor accumulates in alluvial channels as placer deposits, due to the fact that it is harder, heavier, and more chemically resistant than granites containing the resistant weathered grains.
Cassiterite is usually black, purple, or otherwise dark in color. Also, Tin ore deposits from carbonate rocks found in the Kinta region have a high tin content exceeding 1 percent, a complex mineral composition, and admixtures of F, W, Cu, Be, Sb, Pb, Zn, and other elements. Tin has found in some limestone areas; having washed down from the granite ranges, while some occurring in areas relatively separate from limestone. These tin-bearing granites generally date to the Mesozoic era. The tin-bearing alluvium possibly trapped by the buried karst and accumulated in rich deposits, with notable examples in Selangor.
The Tin is considering a rare commodity, mostly found in small amount from the earths crust. Consequently, in overall volume, the quantities of tin- mined are much smaller than other metals like copper, lead,
and zinc. The environmental impact is generally dependent on the sulfide mineral content of tin ore. The principal ore mineral; Cassiterite; is moderate to highly resistant to physical and or chemical weathering, and tend to be concentrated in alluvial deposits. The mobility of tin in natural environment is low (Rose, et.al., 1979). Cassiterite, the principal tin ore mineral, is highly resistant to both chemical and physical weathering, and can be transported long distances from source areas for it to be concentrated in streams, beaches, or marine placers.
- STRUCTURAL STUDY AND THE RELATION WITH KARST ENVIRONMENT
- Karst Environment of Kuala Lumpur and SelangorMost of Kuala Lumpur and Selangor regions have found to be lying over limestone bedrocks from the Silurian period, where karst development had been extensive, classified as Extreme Karst of Class kV by (Waltham and Fookes 2003). These karst features occur in these regions at two scales, described below:
-
-
- Buried karst, with the small-scale of features, in different elevations from ground surface.
- Buried karst, with the large-scale of features below the limestone bedrock, including caves that have formed by the dissolution processes, many of these features are partially or completely in filled with mineral or sediments, while others have created due to roof-collapse and sinkholes.
-
These features, at both scales, impeded the design and construction of engineering structures. Currently, there are no established guidelines for predicting the occurrence of the larger scale features karst in Kuala Lumpur.
The progress in this study toward the determination of relations between the structural geology represented in fracture lines and joints urbanized over limestone rocks and the drainage patterns and river pathways. Weathering and erosion process are expect to be more destructive within fractures scratching the rocks within the rocks of the formations exposed in the study area, compared to its
larger counterpart (Ericson, 2004);( Johansson, M., Migon & Olvmo, M., 2004); (Waltham, T., 2005); (Bell, F., G. & Culshaw, M., G. , 2005). In limestone, the proposed zones of fractures lines that are subject to dissolution is outward in appearance of a major cavities and underground rivers (Sowers, 1976), (Waltham &Fookes, 2003), which implies a relation between structural geology and the groundwater in the study area.
Fracture-pattern analysis and steps were subsequent in the directions of structural elements of the defined marbleized limestone via field observations in Kuala Lumpur and Selangor. Moreover, by utilizing the geological map of the region, in order to obtain the probable direction of the lineaments (faults and fractures) orientation can be determined. Furthermore, radar sat- image has employing to identify the orientation of lineaments (fractures and faults) in both Kuala Lumpur and Selangor. The orientation of lineaments (fractures and faults) that affected the studied regions in Kuala Lumpur and Selangor present in Google terrain satellite map in Figure 9.
The drainage networks of the region were premeditated to acquire the directions and occurrence of river-pathways. The directions of structural elements, faults, fracture-lines and joints were compared with river directions, and from this comparison, it is apparent that rivers and structural elements have certain directions. In addition, the same directions of karst development mean that the expected directions of probable karst are advantageous. Therefore, there is relationship between the karst in Kuala Lumpur limestone and the visible surface of drainage patterns. In this study, discover that most of drainage patterns that creation along each fold outcropped within the study area have based on the direction of the fractures and faults. Due to orogeny movements, that affects the study area in the earlier period and its led to deep-erosion processes in these orientations. These fractures and faults now reflect strong relationship between the orientation of the lineaments and the drainage patterns.
Figure 9: Google terrain, satellite image map showing the orientation of lineaments (fractures and faults) that affected the studied regions in Kuala
Lumpur and Selangor
The structural study on Selangor and Kuala Lumpur employed Radar sat image shown in figure10, showing the orientation of lineaments (fractures and faults) in Kuala Lumpur and Selangor. The image showed evidence of six main sets of fractures and lineaments, originated from many directions, which are NW-SE, N-S, NE-SW, WNW-ESE and ENE-WSW, with a few found in the E-W direction. The fracture-lines directions are displayed using a rose diagram to produce percentages in
several specified direction. The fracture lines have grouped i many classes of equal size, N0010- 0100, N0110- 0200, N0210- 0300.
Figure 11, which present the fracture orientation as determine from above Kuala Lumpur Limestone Formations that takes with direction of north magnetic poles, in (A) at year 1965, and in (B) at year 2012. The differences in reading could be due to (the shift of north magnetic pole with long period about 50 years).
Figure10: Radar sat image showing the orientation of lineaments (fractures and faults) in Kuala Lumpur and Selangor
The limestone of Kuala Lumpurs Formation contains many folds, shown in the satellite map. In addition, the axes of those folds are also clearly can shows on the geological map of Selangor and Kuala Lumpur. The fracture orientations were calculated from the orientation of fold axes and bedding planes, based on assumption that
each of the readings can be used to determine the probable direction of shear and tensile joints of these folds, (Price, 1966). The accumulation of fracture-lines directions was measured from the Grid starting from the direction of North Magnetic pole in the North 00 3° East.
.
Figure 11: Fractures orientations as determine over and above Kuala Lumpur Limestone Formation,
- in year 1965, (B) in year 2012.
-
- Erosion Sequence through the EventsAnalysis of the drainage patterns was conducted on every formation outcropping over the area shown on Kuala Lumpur and Selangor by employing topographicatlas satellite map of Google (2012), and the geological map of Selangor and Kuala Lumpur (1965), reflecting a strong linkage between their direction and the underlying
geological formation. The geological map of Selangor and Kuala Lumpur (1965), shown in Fig. 12, displayed the location of the oldest rocks on the northwest, representing the Hawthornden Formation. Meanwhile, the youngest, on the right to the southeast, represent granites.
The oldest rocks will have undergone extensive deformation, while the youngest the least. The stream
directions in the Hawthornden Formation are more uniformly distribute than that in Kenny Hill Formation to the left shown in the map. The granites contain drainage patterns that are largely independent of the history of their countrys rock. Thus, the stream patterns appear to reflect geological structure. Those same structures might expect in Kuala Lumpur Limestone, which are located between the Hawthornden and Kenny Hill formations. These have based on the estimation of the larger scale karst directions, restricted on the pattern of streams, and their relationship to fractures. The research demonstrated the relationship between karst in Kuala Lumpurs Limestone, and the pattern of visible surface drainage. Figure- 12; shows the distribution of the geological formation in the study area (Kuala Lumpur and Selangor states).
Through the investigation of the drainage features pattern of the whole of the Klang basin on the old geological map and aerial photograph shows that these orientations are visible outside and inside this basin but the control exerted by the orientations of structure is very clear. The drainage pattern is closely adjusting to the structure. Most of the streams follow one of the three dominant orientations. The 30° direction is the most important, followed by the 105° direction, then the 140° direction, is relatively less noticeable. The 30° and 140° directions tend to occur in the second and third order sections in the main stream channels, while the 105° direction affects largely the first order sections. Such the pattern of the drainage networks of the whole of the upper Klang.
The main branch streams come together into the structural basin of the Kuala Lumpur lowlands. The drainage on the sedimentary rocks has a marked frame pattern with north south between Kuala Lumpur and Petaling Jaya and between Sungei Way and Puchong. At right angles to this direction are the east-west sections such as those between Petaling Jaya and Sungei Way and west of Puchong. Some evidence from the original frame pattern still survives in the upper part of the Klang basin. The path of Sungei Gombak has a marked north south in the sections between Kampong Lalang and Batu Caves. Moreover, the path of Sungei Klang takes an east west in the sections
from the confluence of the Sungei Batang Kelang and Sungei Songloi to the confluence with the Sungei Sleh.
Both of Sungai Gombak and Sungai Klang have fissuring the quartz ridge of Klang Gates. The Sungei Gombak is it does not follow the line of weakness of the Gombak Fault but crosses the ridge to the west of it. The Sungei Klang crosses the granite again in its lower course, west of Puchong. Geologically, the observation come consideration that most likely the streams have started flowing as soon as the land has rose above the sea in the Mesozoic era. At that time, the granites intruded lay beneath the sedimentary cover. The drainage net developed on this cover was let down on to the underlying granitic rocks. The original stream pattern was most probably started on a cover of the Kenny hill formation and would have been let down on to the Kuala Lumpur limestone bedrocks and then aboard the schists and eventually reaching the granite.
Drainage pattern on the sedimentary cover would have been a frame network, and once the streams had removed the cover; their courses would have started to become adjusting to the structure of the schistose rocks. A new pattern would be choppy and slowly appear. The analysis of the stream net on the schists to the south of the Klang ridge suggests that are the main directions took by the drainage on this rock is the orientations of 30° and 140°.
It is possible to assume that all the structural orientations reflect conditions on the granite only. The 30° and 140° orientations reflected structure within the granite, while the 105° direction corresponding to more recent movements along the shear plane within the complex fault zone. It has believed that recent fault-movements would be expected to have interrupted and advanced alteration that took place when the drainage net was let down on to the granites (Morgan, R.P., 1976). It may be the reason that under tropical climate conditions alterations the structural influences would be rapid and that will follow minor or slightly orogenic earth movements. The 105° orientation is suitably strong within the Kuala Lumpur fault zone to have caused some basins to develop asymmetrically and it is not common on the schists.
Figure 12: Old geological Map (1993) showing the distribution of the geological formation in the Study regions (Kuala Lumpur and Selangor states)
The long period of weathering in the region which leading, the rocks to undergo in a tropical climate and all size reduced but the most of resistant minerals subdivisions smaller than sand grain size. The further reduction of this material within the rivers have effected more rapidly through solution than by mechanical abrasion, Douglas, (1968).
The size of the river’s load possesses is (little abrasive power) with which to erode and make additional changes to its course. Even in mountainous terrain, where the river capacity is coarser due to layer type, the rivers cannot expect to make rapid changes to new conditions. It is probable that some orientations are common to both of the schists and the granites. The 30° direction is commonly following by lineaments in the granites of Selangor (Tjia,
H.D. & Bignell, I.D., 1976).
The three stages of stream patterns found within the upper Klang basin consequently display evidence of a frame pattern developed on the original sedimentary cover. Its adjusted to the orientations in the schists on which certain basins developed asymmetrically. Sometimes the same orientations found in the schistose rocks that located adjusting to shear planes in the granitic rocks within the Kuala Lumpur fault zone but with further asymmetrical development concentrated. In the lower Klang basin, the stream pattern continue adjusted to the structures of the sedimentary rocks. While in the west of Puchong what has happened the stream come across the overburden of granite.
- Karst Environment of Kuala Lumpur and SelangorMost of Kuala Lumpur and Selangor regions have found to be lying over limestone bedrocks from the Silurian period, where karst development had been extensive, classified as Extreme Karst of Class kV by (Waltham and Fookes 2003). These karst features occur in these regions at two scales, described below:
- FIELD SURVEY TECHNIQUE
-
- Instrumentations and Measurement ProcedureA Multi-Electrode resistivity survey had conducted at selected sites in covered karst terrain, located in Kuala Lumpur and Selangor, Peninsular Malaysia. The survey had carried out via the SAS1000 system, manufactured by ABEM Inc. of Swedish. Fig. 13 shows theSAS1000 system carrying out the survey.
The Two Dimensional electrical resistivity imaging/tomography surveys have conducted, employing a large number of electrodes in the Wenner configuration. The length of each profile is 200 m, with an electrode spacing of 5 m each. Normally, a constant spacing between adjacent electrodes is used. These electrodes had connected to a multi-core cable, configured in a Wenner array (Griffiths and Barker, 1993). The multi-core cable had attached to an electronic switching unit, and is use to automatically select the applicable four electrodes for each measurement.
The field systems have an in-built microprocessor system so that the sequence of measurements to take, the type of array to use, and other survey parameters such as the current to use has entered into a text file, readable by a computer program in a field-systems computer. Different resistivity meters use different formats for the control-file, which requires consultation with the systems manual.
Figure- 13: shows the SAS1000 system, manufactured by ABEM Inc. of Swedish
After reading the control file, the computer program automatically selects the appropriate electrodes for each measurement. This could be a significant advantage for surveys in very rugged terrains. Fig. 6 overviews the typical setup for a 2-D survey, with a number of electrodes
along a straight line, attached to a multi-core cable. The typical setup for a 2-D survey with a number of electrodes along straight line attached to a multi-core cable present in figure 14.
Figure 14: shows the typical setup for a 2-D survey with a number of electrodes along straight line attached to a multi-core cable in different sites in Kuala
Lumpur and Selangor
In the typical survey, most of the fieldwork consists of laying out the cable and electrodes. After that, the measurements are automatically took and store in the computer of the device. To obtain a good 2-D image of the subsurface, the coverage of the measurements must be perfect. In order to obtain the best results, the measurements in a field survey should be carried out in a systematic manner so that, all of the possible measurements are made and recorded. This will affect the quality of the interpretation model obtained from the inversion of the apparent resistivity measurements (Dahlin & Loke, 1998). As the electrode spacing increases, the number of the measurements decreases. The number of measurements that can obtained for each electrodes spacing for a given number of electrodes along the survey line depends on the type of array being use. The Wenner array provides the smallest number of possible measurements compared to the other common arrays that used in the 2-D surveys.
The Electrical Resistivity methods had generally used to explore buried sinkholes and forecast layered collapses. The geological sections of the sinkhole can approximately modeled to examine the effectiveness of the resistivity imaging, which is consider as a two-dimensional exploration approach. If a suitable array distance(s), equal to the diameter and the depth of sinkhole are select, the stratigraphy and the structure of the layers that include
sinkholes can be successfully mapped, which simplifies future sinkhole development assessments and monitoring.
- Geophysical Survey TechniqueA high-resolution geophysical technique has used to realize the case objectives. Electrical Resistivity Tomography (ERT), employed in the Wenner configuration, was functional in order to image the subsurface across the study area as a primary stage, which determines the subsurface karst features and recognize their shape and depth estimation. The Wenner configuration array was chosen based on previous work that showed good resolution of Epi-karst features (sinkholes, cavity and caves with the applying of this technique e.g. (Roth et al., 1999); (Labuda and Baxter, 2001), (Yahia, N. A.,Yassin,
R. R., Abdel Al-Qadir, S. O., 1992).
The Geophysical survey techniques represented in four of Two-Dimensional (2-D) electrical resistivity profiles displayed at site no.1 and oriented in (NE480SW), Fig. 15 reviews the location of resistivity profiles # (1- 4), in selected study site no.1. Additionally, the geophysical survey technique represents five of Two-Dimensional (2-
D) Electrical Resistivity profiles, displayed at site no.2, oriented in (NE85°SW). Fig. 16 reviews the location of resistivity profiles #( 1- 5), in selected study site no.2.
Figure15: Satellite image 2012 display the location of resistivity profiles # (1- 4) in selected study site no.1
Tasik University Malaya, Kuala Lumpur, Peninsular Malaysia.
The location of resistivity profiles # (1- 5) in site#2, Padang Merbuk, Jalan Parlimen, Kuala Lumpur, Peninsular Malaysia display Satellite image 2012 , figure 16.
Figure 16: Satellite image 2012 display the location of resistivity profiles # (1- 5) in site#1, Padang Merbuk, Jalan Parlimen, Kuala Lumpur, Peninsular
Malaysia
Furthermore, the Geophysical survey technique represents six of Two-Dimensional (2-D) Electrical Resistivity profiles displayed at site no.3, oriented in (NW45°SE).Fig. 17 reviews the location of resistivity profiles # (1- 6), in the selected study site no.3.
Figure 17: Satellite image 2012 display the location of resistivity profiles # (1- 6) in selected study site no.3, Batu cave, Selangor, Malaysia peninsular
Additionally, the geophysical survey technique represented in the fourth of Two-Dimensional (2-D) electrical resistivity profiles is display in selected study site no.4, oriented in (NE45°SW).Fig. 18 reviews the location of resistivity profiles no. (1- 4), in the selected study site no.4.
Figure 18: Satellite image 2012 display the location of resistivity profiles #( 1- 4) in selected study site no.4, Asia Jaya, Petaling Jaya, Selangor, Peninsular
Malaysia.
- Data CollectionThe Electrical Resistivity data were collected along the ERT survey performed with many Two-Dimensional (2-D) electrical resistivity profiles obtained over unknown and suspected filled Epi-karst features such as sinkholes and cavities across four sites, which were Task University Malaya, Jalan Parlimen, Kuala Lumpur and Batu Caves, Petaling Jaya, Selangor. ER resistivity tomography cross- sections imaging have conducted on the Epi-karst features, and then followed by borings with Auger to verify the accuracy of the resistivity models that contain a modicum of evidence. About 190 data points have collected for each (41-electrodes) in a single profile, and on average, about 760 data have collected for a total 4th profiles at site no.1. Besides, on average, about 950 data has collected for a total of fifth profiles at site no.2. While, about 1150 data were collecting for a total of6th profiles in site no.3. Finally, about 760 data have collected for a total of4th profiles, at site no.4.On average, about 2100 data were collected form a total of four profiles at four sites.
- Data ProcessingPost-field survey, the resistivity measurements are usually concentrated on the apparent resistivity values. Nowadays, almost all commercial multi-electrode systems come hooked up to a computer. The raw apparent resistivity Wenner data had inverted and interpreted using the rapid two-dimensional (2D) resistivity inversion least squares method, Loke, (1998). The program used RES2DINV (ver. 3.4) to acquire a 2D true earth resistivities inversion soution, which is then contoured. These 2-D images may still contain a quantity of distortions and artifacts from the modeling process. The values near the base of the section, for instance, are often incorporated with effects or off-line information projected onto the section, due to lateral current spreading at wide electrode spacing. The instrument SAS are connected to a laptop computer by a special cable for data transfer, which were then processed to generate 2-D resistivity models of the subsurface. It assumes that the subsurface is uniformly layer; hence resulting in lateral smoothing i.e. mixing will occur in non-layered strata. This step involved in converting the apparent resistivity values into a resistivity model section can use for geological interpretation. The data has already saved in the RES2DINV format. This inversion software is developing by (Loke and Barker 1996), and the conversion program is bundled with many commercial systems. The inferior data points, caused by different problems such as poor ground contact for some of the electrodes, will contain significantly higher errors and show more complicate patterns compared to their non- problematic counterparts.
As a rule of thumb, data points with errors of 100 percent and above can usually remove. In this survey, the Root Mean Square (RMS) errors statistics quantify the distribution of the percentage difference between the logarithms of the measured resistivity values, and those calculated from the true resistivity model (calculated apparent resistivity values). Those with a small RMS value
indicates a close match defined by the convergence limit, the default value of which is with the average of 5% change in the RMS error between iterations. The average RMS error is 7.25%, which is the change in the RMS error between iterations. These error values fluctuate between a minimum of 4.3% and a maximum of 18.3%.
The interpretation of bedrock surface elevation via boring data from 20 soil borings (Figure 6) has used to define the limestone/clay boundaries. The imperfection in the soil boring data recorded may be from the top of the weathered limestone zone, rather than the top of unweathered bedrock. Residual limestone rocks or cherty boulders in the overburden may have impeded the development of some borings.
- Instrumentations and Measurement ProcedureA Multi-Electrode resistivity survey had conducted at selected sites in covered karst terrain, located in Kuala Lumpur and Selangor, Peninsular Malaysia. The survey had carried out via the SAS1000 system, manufactured by ABEM Inc. of Swedish. Fig. 13 shows theSAS1000 system carrying out the survey.
-
- ANALYSIS AND INTERPRETATIONS OF RESISTIVITY PROFILES DATA
- INTRODUCTIONAccording to the established definition, karst is generally a terrain underlain by limestone, dolomite, dolostone and marbleized limestone, where the topography is primarily formed by the dissolving rocks. A karst terrain is often recognizable by a wide range of closed surface depressions, well-developed subterranean drainage, sinkholes, sinking streams, springs, caves and noticeable interactions between the circulation of surface and groundwater. Gutters are features that are separating by upward-protruding limestone, known as pinnacles. Carbonate rocks contain various amounts of insoluble materials.
The ERT technique applied in this geo-electrical survey to imaging the shallow subsurface from a depth of less than 2.0 m, to a maximum depth of less than 29.0 m. This technique has used in the karstified carbonate regions as a function to investigated karst features such as sinkholes, cavities, depressions and channel pipes. The survey found that this tool is suitable for differentiating surficial soil, clay, sand; weathered marbleized limestone bedrocks, intact marbleized limestone bedrocks and water and air-filled cavities.
In addition, it has applied due to its requiring less effort and more time effective. Its based on the application of electric current into analyzed bedrock and measuring the intensity of electric resistivity to its conduit. Basically, its gives information of electric resistivity properties through the analyzed material towards electrical current passage, (Telford, W.M. et al. ,1990).
Mostly, when the overburden soil is clay-dominate. The electrical conductivity of clayey soil and carbonate rock has an electrolytic origin, whereas most earths materials are not electrical conductors.
According to Archies law, (La Moreaux et. al., 1984), electricity has conducted through interstitial water by ionic transport. Generally, Carbonate rock has a significantly higher resistivity than clayey soil due to its much smaller primary porosity and fewer interconnected pore spaces. The typical resistivity value is more than 1000 ohm-m (Telford W.M. et al., 1990). Clayey materials tend to hold more moisture and have a higher concentration of ion to conduct electricity, therefore having resistivity values of less than 100 ohm-m (Telford, W.M. et al., 1990).
Several reports and researches on application of geophysical techniques in many karst terrains in several countries around the world have examined. These reports and researches have done by (Anderson, N.L. et al., 2007), (Louis, I. F. et al., 2002). Moreover, other research had
completed by (Yassin, R. R. , 2002) and (Zhou, et. al.
,2000). Finally, earlier research had completed by (Yahia, et al., 1992). These reports enabled the determination of the electrical variables associated with the nature of sediments. Conclusions have made based on the variations in electrical resistivity values related with the nature of sediments.
The geological classification permits the successful imaging of the bedrock and subsurface karstic features, because soil, sand, clay, carbonate rock and air-filled cavities can normally be differentiated and mapped.
Clay
Are usually distinguished by low apparent resistivitys and variables, which are dependent on moisture, mineral content, purity, and unit shape/size, usually from( 05) ohm- m to less than ( 60) Ohm-m. In this case, the clay has divided into many types with different colors, which have use in this resistivity section as:
-
-
- Highly moisturized soft clay is usually distinguish by insufficient low apparent resistivity with water- filled porosity or very high mineralized. In addition, has typically given Maroon color in this study.
- Soft clay with pond water content has usually distinguished by extremely low resistivity and has very high conductivity or highly mineralized. Moreover, has typically given dark Red color in this study.
- Moderate moisturized soft clay is usually distinguished by Very low apparent resistivity and has very high conductivity or moderate mineralized. In addition, has typically given Orange color in this study.
- Clay with low-moisturized are usually distinguished by low apparent resistivity or with low mineralized content. Moreover, has typically given yellow color in this study.Sand
Is usually characterize by medium apparent resistivity and variables, depending on the moisture content, purity and unit size, usually from (70) Ohm-m, to less than (160) Ohm-m. The sand is also dividing into many types; its colorings scheme described below:
- Sand, distinguished by medium apparent resistivity, is typically dark green color in this study.
- Sandy clay, distinguished by its low medium apparent resistivity, has typically given light green color in this study.Weathered limestone rock
- Comparatively weathered limestone rock had typified by high apparent resistivites, typicallymore than (200) Ohm-m, to less than (400) Ohm- m, has typically given blue color in this study.
Intact limestone rock
- Is distinguished by higher apparent resistivity, naturally from more than (400) Ohm-m to more than (3000) Ohm-m, and varies depending on layer thickness, its impurities and moisture content. It has given a Navy color in this study.Intact pure marbleized limestone or dolostone rocks
- Is distinguished by higher apparent resistivity, naturally from more than (4000) Ohm-m to more than (8000) Ohm-m, and varies depending on layer thickness, its impurities and moisture content. It has given a dark Blue color in this study.Air-filled cavities or voids
- Are generally characterized by very high apparent resistivity, usually more than (4000) Ohm-m to less than (6000) Ohm-m, but varies depending on the conductivity of the nearby strata and size/shape of void or cavity. Classically, it takes a black color in this study.Transitional zone
- Mostly high-apparent resistivity (160) Ohm-m(200) Ohm -m, consists mostly of rock fragments and sand and or marly limestone. Classically, it takes a Khaki color in this study.
Radon
- Radon gas hasmostly found in karst area with unground features such as buried sinkholes, that containing water or underground caves and channels. Mostly has extremely very low – apparent resistivity from (1) Ohm-m (3) Ohm m.
-
Hence, electrical resistivity values were resolute for each rock unit. The results have tabulated in Table 1. This table was suitable for investigation karst features and its deposits within karst terrains. Also in the same time was suitable for detecting any mineral deposits within the sediments in the area but it need experience for that.
The geological classifications have utilized in this geo-electrical survey that describe above it permits the successful imaging to mapping and differentiated bedrocks and subsurface karstic features. Because there is crossing point between the value of resistivity data of intact limestone and the value of resistivity data to define karst features in fill with air such as cave, cavity and sinkholes. The table above can use for twice time, one with resistivity from 3000 – 6000 -m to define the cave, cavity and sinkhole with air-infill, table 1.
Table 1: Describes the range of resistivity values with the expected geological unit deposits to define the cave, cavity and sinkhole with air-infill.
In addition, to define rock fragments of limestone with friable and coarse grains sand which containing high porosity with air. In the second time with resistivity from 3000- 6000-m as intact limestone, table 2. To define the weathered, intact limestone and intact marbleized limestone without mention to cavity and sinkhole with air-infill.
Table 2: Describes the range of resistivity values with the expected geological unit deposits to define the weathered, intact
limestone and intact marbleized limestone
In the following the analysis and interpretation of the data collected from resistivity profiles. The acquired data was interpretated by applying the geological classification originating from the variations in electrical resistivity values, which describes the range of resistivity values with the expected geological units deposit.
These classifications is the key interpretations of the geoelectrical data in order to understand the resistivity profiles and the function of sinkholes and other subsurface karstic features that investigated at these sites. In addition, this classifications has successfully used before for exploration of mineral deposits in karst area, (Yassin, R.R., 2002). The interpretation of resistivity data identifies the points illustrated in the objective of the study.
The smoothing that observed in the subsurface limestone bedrock is the result of employing the RES2DINVver.3.54. Software, which assumes that the subsurface is uniform, layered; and consequently lateral smoothing will form in a non-layered stratum. Some of the boreholes has completed by auger to the depth of 10 m at some point, facilitating in the interpretation of covered sediments in these sites.
The high contrast in resistivity values between the carbonate rocks and the clayey soil is favorites to use of the geo-electrical resistivity method to delineate the boundary
between bedrocks and overburden. The Wenner arrays provide the highest resolution, and are most sensitive to vertical resistivity boundaries found at pinnacles and gutter interfaces. However, the data collected from the Wenner array are sensitive to near-surface resistivity variations, Griffiths & Barker, (1993), and therefore can produce noisy data. Computerized instrumentation permits automatic selection of four electrodes from a multiple-electrode array to use for each measurement, which significantly expedites the data collection process. The measuring of apparent resistivity is volume-averaged values that had affected by all the geologic layers, through which the induced electric current flows. An inversion program converts the array of apparent resistivity data into a model of the geology that would yield the observed distribution of the apparent resistivity values. The product of the data inversion process is a 2-D image. The extensive description of the classification described above is the key for the interpretations of the geological survey that had carried out to understand the resistivity profiles and the function of sinkholes and other investigated subsurface karstic features. The interpretation of the resistivity data in the absence of borehole control in the area was relatively complex. Some of boreholes has completed by auger reaching the depth of
~10m. At some point, it facilitates the interpretation of covered sediments in these sites.
- 1 ANALYSIS AND INTERPRETATION OF RESISTIVITY PROFILES DATA IN SITE #1 (TASIK UNIVERSITYMALAYA)
Figure (19 – A): The Inverse model of electrical resistivity section for profile#1
Figure (19 – B): The Inverse model of electrical resistivity section for profile#2
Figure (19 – C): The Inverse model of electrical resistivity section for profile#3
Figure (19 – D): The Inverse model of electrical resistivity section for profile#4
Figure 19(A-D): The inverse model of electrical resistivity section from profiles#1 to profiles#4, viewing the interpreted location of shallow karst features (sinkholes and cavities) in site #1 (Tasik university Malaya)
Through the interpretation of E. R. Tomography section in profile #1, figure (19-A), of this site, observed zone of medium resistivity, a shape in the form of channel or stream valley between two rock head, in- filled with silty sand and sand, extended between electrode 18- 31 and reaching a depth of >28 m. In the top -middle, clay deposits has shown between electrodes no. 22-29. In the left flank of this profile, uniform rock head of marbleized limestone observed laying near the surface beneath electrodes no. 4- 19, from depth 6.36m down to depth >28m. Rock head Pinnacle have clearly observed to be located beneath electrode 31 -32 at a depth of ~10m.
The interpretation of E. R. Tomography section in profile #2, (figure 19-B), of this site showed that the same channel or stream valley in- filled mostly with clay and some sand, extended between electrodes E20-E31.
Zone of very low resistivity had detected between electrodes 20-30, representing buried sinkhole. The depth has reached to more than 28m.Very low resistivity were detected between electrodes 23- 30; representing lens of clay contains of highly moisturized clay and water. Another lens very deep, appear between electrodes E14-E18, at a depth of 24.0m.
Small sink or pits of clay -infill appear in the surface between electrodes no.E10-E11, E12-E14 & E17- E18. In addition, sand had detected on the surface between electrodes E13-E18.inclined marbleized limestone bedrocks have observed beneath electrodes no.E26-E39, at a depth of 24.0m, beneath electrode no.E35, and at a depth of 6.38m, beneath electrode E35. Floater of limestone appears between electrodesE13-E16 at a depth between
8.0-14.0m. Small pits observed nearly between electrodes E2-E3, in-fill with sand and rock fragments by remediation technique.
The interpretation of E. R. Tomography section in profile no. #3 figure (19-C), of this site showed that the same channel or stream valley between two rock head, in- filled mostly with clay and some sand, extended between electrodes no.E10-E34. Very low resistivity appears between electrodes no. E24-E30, representing buried sinkhole contains of highly moisturizing (clay and water and some of radon gas). Cover soil subsidence observed on the surface, one small between electrodes no.E2-E3 and the other medium between electrodes no.E13-E18, in-fill with sand and rock fragments by remediation technique.
In the left flank of this profile, uniform rock head of marbleized limestone observed lying near the surface beneath electrode no.E4-E12, from the depths between 4.0m and 6.0m, down to depth of >28m. Limestone bedrock was observed beneath electrodes no.E33-E39, from the depth between 9.0 and 6.0m down to depth of >28m. Pinnacle rock head observed beneath electrodes no.E9-E10, at the depth of 4.0m, beneath electrodes no.E33-E34, at the depth of 8.0m and the other between the electrodes no.E38-E39 at the depth of 4.0m.
The interpretation of E. R. Tomography section in profile #4, figure (19-D), of this site showed that the channel or stream valley decay to the right flank, sand in- fill was detected on the surface between electrodes no.E31- E37 to depth of >28m. Small sink or pits of clay in-fill appear in the surface between electrodes no.E3-E5, E29- E31 and E37-E39.
Uniform rock head of marbleized limestone observed laying near the surface beneath electrodes no.E5- E24, from depth between 8.0m and 6.0m down to depth of >28m. Although, rock head Pinnacle has observed beneath electrodes no.E6-E17, at the depth of 3.0m.
-
- ANALYSIS AND INTERPRETATION OF RESISTIVITY PROFILES DATA IN SITE #2 (JALAN PARLIMEN)Figure (20 – A) : The Inverse model of electrical resistivity section for profile #1
Figure (20 – B): The Inverse model of electrical resistivity section for profile #2
Figure (20 – C): The Inverse model of electrical resistivity section for profile #3
Figure (20 – D): The Inverse model of electrical resistivity section for profile #4
Figure (20 – E): The Inverse model of electrical resistivity section for profile #5
Figure 20(A – E): E. R. Tomography sections from profiles#1 to profiles#5, viewing the interpreted location of subsurface karst features (sinkholes and cavities) in in site#2, Jalan Parlimen, Kuala Lumpur
Kuala Lumpur region known as a region with near surface over hanging layers of intact marbleized bedrocks and underground combined caves systems and this will show in this study area.
Through the interpretation of E. R. Tomography section in profile #1, figure (20-A), near surface over hanging layers of intact marbleized bed rocks shown extended along this profile. The depth of this bed has varied from the surface 1.5m down to a depth between 8.0m and 12.4m. Furthermore, those beds effected by strong dissolutions process that end in forming subsurface dissolution sinkhole extending between electrodes no.E22- E30, and appears from the depth of 1.50m down to reach a depth of 26m, which may indicate an enlarged fracture.
This sinkhole in-fill with low resistivites values due to presence of unconsolidated materials of silty sand, clay and clay soil.
The layers of intact marbleized limestone bedrocks observed between electrodes no.E19- E23 continuing down to the depth of >28m. Cave or cavity in-fill with sand are observed beneath the weathered marbleized limestone between electrodes no.E8-E27 at a depth of ~6.5m down to the depth of ~24.0m. Due to applying of ERT technique, this method limited by its maximum depth about 30 m, and then the description of this cave or cavity escapes in this work.
Through the interpretation of E. R. Tomography section in profile #2, figure (20-B), near surface over hanging layers of intact marbleized bedrocks shown
extended along this profile. The depth of this bed has varied from the surface 1.5m-5.0m down to the depth between 8.0m 25.0m. Furthermore, these beds effect by strong dissolution process, which end in forming subsurface dissolution sinkhole extending between electrodes E20- E31, and appears from depth 4.50m down to reach a depth of >26m, which may indicate an enlarged fracture.
This sinkhole is in-fill with unconsolidated materials of; sand, silty sand, clay and silty clay. The low resistivities values are due to presence of moisturized clay and radon gas observed in the core of this Sinkhole. Its look like the meteoric waters passes from the surface through the conduit beneath electrode no.E33 to the sinkhole.
The cover layers consisting of unconsolidated material of; soil, clay, silty sand and sand from the top surface until the depth of 6.38m, in the left flank of this profile. The right flank showed thick deposits of clay in trough extended between electrodes no. E33-E39, from the surface down to the depth of 9.0m
Uniform rock head of marbleized limestone observed lying near the surface beneath electrode electrodes E4 – E20 continuing down to reach a depth between 24.0 to more than 28.0m in left flank. In the right, flank the weathered marbleized limestone between electrodes E20-E32 with depth of ~5.5m. Besides, rock head pinnacles detected between electrodes no. E30 – E33. Due to applying of this technique its limited by its maximum depth; 30 m, so the description of this cave or cavity escapes in this work.
Through the interpretation of E. R. Tomography section in profile #3, figure (20-C), a huge gutter appearing extended beneath electrodes no.E3-E33, infill with sand. The cover layers consist of unconsolidated material of, clay soil, silty from the surface until depth of 6.38 m -10.0m in
the left flank of this profile. The clay layer shows the presence of some meteoric water after rainy day.
The disappearing of near surface intact marbleized limestone bedrocks in left flank, and extended beneath electrodes no. E34-E40, in the right flank from the depth 1.50m near the surface then inclined in electrode no.E34 to a depth of ~26.5m, until the electrode no.E27. Floating of weathered limestone observed beneath electrodes no.E11- E14, at a depth of ~12.5m
The interpretation of E. R. Tomography section in profile #4, figure (20-D), shown that the disappearing of the huge gutter, and extension of limestone body extended beneath electrodes no.E8-E39. The cover layers consist of unconsolidated material of, clay soil, silty from the surface until a depth of 6.38 m -12.4m beneath electrodes E3-E33.
Cave or cavity has observed in the weathered marbleized limestone bedrock, sand in-filled, extended between electrodes no.E17-E24 at depth of ~24.5m. However, due to the maximum allowable depth of this method only being 28.7 m, the description of this cave or cavity lack in this study.
The interpretation of E. R. Tomography section in profile #5, figure (20-E), displayed uneven cover layers consist unconsolidated material of clay soil, silty from the surface till a depth of 5.50 m -7.5m beneath electrodes no.E3-E39.
The appearing of near surface layers of weathered and intact marbleized limestone bedrocks extended along the profile beneath electrodes no.E5-E39. Dual caves or cavities have observed in the weathered marbleized limestone bedrock, sand in-filled, extended between electrodes no.E15-E17 and electrodes no.E22-E26 at depth of ~22.5m. However, due to the maximum allowable depth of this method only being 28.7 m, the description of this cave or cavity lack in this study and if those dual cavities connected to the one appear in the profile #4, figure (20-D).
- ANALYSIS AND INTERPRETATION OF RESISTIVITY PROFILES DATA IN SITE #3(BATU CAVES)
- ANALYSIS AND INTERPRETATION OF RESISTIVITY PROFILES DATA IN SITE #2 (JALAN PARLIMEN)Figure (20 – A) : The Inverse model of electrical resistivity section for profile #1
Figure (21 – A): The Inverse model of electrical resistivity section for profile #1
Figure (21 – B): The Inverse model of electrical resistivity section for profile #2
Figure (21 – C): The Inverse model of electrical resistivity section for profile #3
Figure (21 – D) : The Inverse model of electrical resistivity section for profile #4
Figure (21 – E): The Inverse model of electrical resistivity section for profile #5
Figure (21 – F): The Inverse model of electrical resistivity section for profile #6
Figure 21(A-F): The Inverse model of electrical resistivity section from profiles#1 to profiles#6, viewing the interpreted location of shallow karst features
(sinkholes and cavities) in site#3, Batu cave, Selangor
Batu cave known as an area with underground combined caves systems and this will show in this study. The study area locates immediately behind dark cave. Through the interpretation of E. R. Tomography section in profile #1, figure (21-A), near surface over hanging layers of intact marbleized bed rocks shown extended along this profile and profile #2, #3,#5& #6. The depth of this bed varied between 17m and 12.5m. Furthermore, these beds covered with thin layers of unconsolidated material of soil clay and sand until the depth of 1.25 m. In profiles #1, 5&6 this bedrocks affected by high dissolution process of stream sinks.
Meteoric water running down from the limestone
hills that located narrowly to the study area and pass downward through fractures winded by erosions to process the water channel pipe that observed in E. R. Tomography section in profile #1, in between electrodes no.E5-E6. In profile #2, in between electrodes no.E7-E8& E31-E32. In profile#3, in between electrodes no.E9-E10&E32-E33. Besides, in the profile #5, observed in between electrodes no.E6-E8.
A sinkhole appearing in underground surface in profile#3, figure (21-C), extended between electrodes no.E17- E26, in-filled with sediments and meteoric water that running downward through the water channel pipe. In addition, another buried sinkhole is clearly had spotted near
the surface in profile#4, in-filled with soil clay and other material moisturized with meteoric water. Furthermore, radon detected with electrical resistivity between1-3 shown with pinky color in all underground water of this karst area, figure (21-D).
Rock cliff of marbleized limestone appears in the middle of profile #4 between electrodes no.E7- E20. In profile #5, in between electrodes no.E9-E30. In addition, in profile #6, in between electrodes no.E5-E20. Furthermore, high resistivites of marbleized limestone representative of pinnacles mostly observed in ERT section in profile #1 extended between electrodes E14 – E17. In profile #2,
extended between electrodes no.E15 – E17. In profile#3, extended in between electrodes no.E15 – E17 & E25 – E27. Uneven Trough or Gutter in-fill with sediments of sand and clay until depth >28 observed in the Tomography section of profile #6 between electrodes E20 E35.
This E. R. Tomography technique has used being limited to a depth of 30 m. This limitation severely limits our attempt to understand the activities of the underground combined caves systems and cavities that discovered in this profile. The marbleized limestone rocks very near to the surface and gives high resistivites, and after heavy raining day only could take the reading.
- 4 ANALYSIS AND INTERPRETATION OF RESISTIVITY PROFILES DATA IN SITE #4 (PETALING JAYA)Figure (22 – A): The Inverse model of electrical resistivity section for profile #1
Figure (22- B): The Inverse model of electrical resistivity section for profile #2
Figure (22 – C): The Inverse model of electrical resistivity section for profile#3
Figure (22 – D): The Inverse model of electrical resistivity section for profile #4
Figure 22(A-D): Inverse model of electrical resistivity section from profiles#1 to profiles#4, viewing the interpreted location of shallow karst features
(sinkholes and cavities) in site #4 Petaling Jaya, Selangor
Through the interpretation of E. R. Tomography section in profile #1, figure (22-A), many karst features were observed in the tomography sections. This profile mostly contains high quantity of clay extended from electrodes E8-E33 on the surface on the right flank with depth of 6.33in the left flank to reach a depth of 19.8m.
An trough contain subsidence sinkhole observable beneath electrodes E13-E22, reaching a depth of 24m, containing deposits with low resistivity values and some suspensions meteoric waters. Pocket of high resistivity value represent mixture of friable sand and rock fragments found over this sinkhole and are observe via site inspection, represent surface engineering remediation to fill subsidence area. Besides, limestone pinnacles were observe beneath electrodes 10-14 at depth of 6.33m, and beneath electrodes 24-29 at a depth of 15.0m.
In profile #2, figure (22-B), several lenses were observed in the tomography section along this profile, with varying shape and depth from the surface, between electrodes mo.E21-E25, E27-E29 and E30-E33. These lenses are in-filled with clay soil with low resistivity values with a quantity of suspension meteoric water. Channel observed in left flank, including longitudinal conductive body of clay extended between electrodes no.E6-E18, ended with lens or older subsidence sinkholes.
Meanwhile, many pockets of high resistivity value filling pits represent mixture of friable sand and rock fragments re found over these lenses and are observe via site inspection. However, represent surface engineering remediation to fill subsidence area. Besides, in this profile in many places represent surface engineering remediation. Finally, limestone pinnacles observed beneath electrode no.E16-E17, with a depth of 12.4m, and beneath electrode no.E28-E29, with a depth of 17.0m.
In profile #3, figure (22-C), a longitudinal conductive body of soil clay represent a channel extended between electrodes E5-E32, including lenses in-filled with clay deposits containing meteoric water throughout between electrodes E13-E14, E17-E21, E22-E32, and E34- E39.
The lens has observed in this profile between electrodes no.E22-E32 clearly represents old subsidence sinkholes with a depth of more than 24m. Materials with High resistivity values filling pits represent mixture of friable sand and rock fragments found over these lenses and are observe via site inspection. However, this leading to the conclusion that the surface engineering remediation was used this material to refill the subsidence area over the sinkholes. Finally, limestone pinnacles were observed beneath electrodes no.E15-E16, with a depth of >19.8m, and the other spotted adjacent to the surface beneath electrode no.E32-E33, with a depth of 5.0m.
In profile #4, figure (22-D), sinkholes were spotted in the middle of this profile between electrodes E19-E26, with a depth of 3m-5m from the surface, down to depth of
more than 28.0m. A longitudinal conductive body of soil clay represents a channel extended between electrodes no.E3-E20. Finally, limestone pinnacles were observed beneath electrodes no.E15-E16, with a depth of >19.8m.
- THE DEPTH OF MARBLEIZED LIMESTONE BEDROCK IN THE STUDY SITESLarge numbers of geotechnical problems have occurred during the construction of the previous major engineering projects on the Carbonate Karst terrains. These problems are creating the complexity by increased expansion of the karsts morphology. Often, only engineers who are familiar with soluble rock understand these anomalies and problems that are associated with it.
The tables below present an outline or portrayal of some selected points regarding the marbleized limestone bedrock depth of the four (4) sites in Kuala Lumpur and Selangor that will be favorable for engineers for them to recognize the depth of limestone in these selected construction sites under studies. These are, however, incomplete and can only provide common suggestions of projected ground conditions, despite the possibility of ending up with enormous discrepancies regarding the depth of the local features.
The survey shows that the depth of marbleized limestone bedrocks in these sites was uneven or asymmetrical and possesses numerous pinnacles and gutters. Table 10-A, B, C, D and E described the approximate depths of weathered, intact marbleized limestone bedrock and pinnacles in the selected construction sites through the interpretation of E. R. Tomography section.
In construction site #1(Tasik University Malaya), the limestone bedrock was deep, the depth of weathered limestone bedrock was deep 22.0 m. For intact limestone bedrock, the depth varied between 22.0 m and >28.0m. However, what we recognize and the depth of pinnacles varied between 3.0m- 9 6.0 m.
In construction site #2(Jalan Parlimen), the effect of dissolution process clearly observed in the images of profile section. In this site, the depth of weathered limestone bedrock mottled between 1.25 m and 12.4m. The depth of an intact imestone bedrock varied between 4.0m and >14.0m, while the depth of its pinnacles varied between 7.0 m and 28.0m.
In construction site #3(Batu Cave), the effect of dissolution process clearly observed in the images of profile section the depth of weathered limestone bedrock mottled between 1.25m and 15.0m. The depth of intact limestone bedrock varied between 2.0 m and 24.0 >28.0m, and the depth of pinnacles varied between 5.0 m and 12.0 m.
In construction site #4, the depth of weathered limestone bedrock mottled between > 12.4 m. The depth of
intact limestone bedrock varied between >22.0m. The depth of intact limestone bedrock varied between 8.0 m and>28.0m, and the depth of pinnacles varied between 9.0 m and 28.0 m.
This study clarified that the marbleized limestone bed rock in site 1 (Tasek university Malaya) and site 4 (Petaling Jaya) is deep only what observed in ER Tomography sections of both sites. Besides, this study clarified that the marbleized limestone bed rock in site 2 (Jalan Parlimen)
and site 3 (Batu cave) is shallow and contain numerous combined caves system and cavities what observed in ER Tomography sections of both sites.
This E. R. Tomography technique has limited to the depth of 28.6 m. This limitation severely limits our attempt to understand the activities of the underground combined caves systems and cavities that discover in these sites.
Table 10.A: Described the approximate depths of weathered, intact marbleized limestone bedrock and pinnacles in site #1 (Tasik University Malaya) through the interpretation of E. R. Tomography section.
Table 10.B: Described the approximate depths of weathered, intact marbleized limestone bedrock and pinnacles in site #2 (Jalan Parlimen) through the
interpretation of E. R. Tomography section.
Table 10.C: Described the approximate depths of weathered, intact marbleized limestone bedrock and pinnacles in site #3 (Batu Cave) through the interpretation of E. R. Tomography section.
Table 10.D: Described the approximate depths of weathered, intact marbleized limestone bedrock and pinnacles in site #4 (Petaling Jaya) through the interpretation of E. R. Tomography section.
- SUPPORTING E.R.TOMOGRAPHY RESULT BY OCCUPYING APPROPRIATE DRILLING DATAThe Electrical resistivity data were acquired from these designated sites, and 20 borings holes were opened using the (Hand-auger method), which are rotated by hand, cut into the soil with blades and pass the cutting material up a into bucket auger. The dug material removed and the auguring continued to reach the required depth. An auger that was 12 m long is used prepared locally for this project on the other hand it is a cheap and simple process. The auger drilling by hand is slow and limited to a depth of about 10-12 m maximum.
The borings is completed in unconsolidated material of soil, clay, sandy or silty clay, and sand layers deposits not coarser than (coarse sand) over karstified limestone environment. The depth of boring holes reached approximately about 2m, 5m, 10m, and 12m. The depth to bedrock has defined as the depth that the auger refused in the borings. This borings have designed to be able to spatial define some karst features, small cavities and a few secondary processes deposits. Furthermore, the samples
from these 20 borings have analyzed. The length of the auger blade varies from 0.30-0.50m.The auger is rotated until it is full of soil, then it is withdrawn to remove the soil and the soil type present at various depths is noted. Repeated with drawl of auger for soil removal makes boring difficult below 8-10m depth. The soil samples collected in this manner are disturbed samples and can used for classification test. Impossible in very non-stiff clay or coarse friable sand to auger boring may because the hole tends to collapse when the auger has removed.
Investigation by Bore hole sampling using of (mechanical auger ) at Kuala Lumpur and Selangor till depth more than 20-22m to provide appreciated informations and assemble as much data as possible about the underlying strata of areas, then to compare the Boring data with that of geophysical surveys.
The ground conditions in this study area are mostly consist of two major soil formations; first the Kenny Hill sedimentary rock and second the sand over formation and the Kuala Lumpur limestone formation which is highly weathered karstic.
One of the borings stumbled upon the borings encountered clay in fill cave over the ERT profile. The boring also came across clay-filled fractures, caves and pipes at depths of 1.6 and 3.8 m, as well as a small clay- filled void at about 6.3 m deep.
Pipes are representing as high-conductivity (low resistivity) zones in the ERT images. Soil pipes are
associated with low resistivity zones on the Wenner ERT sections. All of these pipes are above the water table, so the low resistivites may be due to infilling by clay that are highly mineralized, or pockets of suspended water within the pipes. Borings in different sites in Kuala Lumpur and Selangor by employing different types of Augur in studied the sallow subsurface karst features deposits present in figure 23.
Figure 23: borings in different sites in Kuala Lumpur and Selangor by employing different types of Augur in studied the sallow subsurface karst features
deposits.
A typical soil profile from an ex-mining site has shown that the soil is erratic, consists of very soft clayey to very loose sandy materials. Concrete blocks are coming across in some boreholes. The thickness of the dump has 10m up to 14m in some boreholes. The test of boring carried out from Bore hole sampling using of (mechanical auger ) at Kuala Lumpur and Selangor the site showed the dump material highly varied, consisting of concrete blocks, wood, steel bars, metal scraps in conjunction with the ground.
Unconfined compressive strengths (UCS) of the Kuala Lumpur limestone from a few locations have abbreviated in as including a low values due to failures along fissures (fractures and cavities). The limestone of Kuala Lumpur is of upper Silurian marble, finely crystalline, variably dolomitic rock. Banded marble, dolomite, and pure calcitic limestone as describe by (Gobbet & Hutchison, 1973). The limestone overhang is assume, in boreholes cavities with vertical dimensions of up to 03m have come across, due to the material above and underneath the overhang is of the same nature.
Through the drilling of bore holes, in site no.1, profile #1, borehole no.1,drilling point E20, Table 3, the thickness of the soil layer abut 1.0m from the surface, after that silty sand till depth 6.0, then layer of sand with some rock fragments extended over depth >20.0m.
Table 3: Viewing the description of bore holes in site #1 (Tasik University Malaya)
In profile #2, borehole no.2,drilling point E25, the thickness of the soil layer from the surface till the depth
1.25 m, after that layer of stiff clay till depth 3.0m, then layer of non- stiff moisturized clay extended till the depth of >12.4m. then layer of stiff clay till the depth of 17.0 m, after that layer of silty clay till depth 19.0m, then layer of silty sand till depth 20.0m.then layer of sand extended over the depth of >20.0m.
In profile #3, borehole no.3,drilling point E25, thin crust of the soil layer from the surface till the depth 1.25 m, after that layer of non-stiff moisturized clay extended over the depth of >12.0m.
In profile #4, borehole no.4,drilling point E15, the thickness of the clayey soil layer from the surface till the depth of 5.0 m, after that layer of silty sand till depth 8.0m, then layer of compact sand extended over the depth of
>12.0m.
Through the drilling of bore holes, in site no.2, profile #1, borehole no.5,drilling point E20, Table 4, the thickness of the soil layer abut 0.50m from the surface, after that layer of intact marbleized limestone bed rock depth extended over depth >12.0m.
Table 4: Viewing te description of boreholes in site #2 (Jalan Parlimen)
In profile #2, borehole no.6, drilling point E10, the thickness of the soil layer from the surface till the depth
1.50 m, after that layer of silty sand till depth 2.50m, then layer of compact sand till depth 6.00m , then rock fragments and sand till depth 6.00m, after layer of intact marbleized limestone bed rock depth extended over depth
>6.0m.
In profile #3, borehole no.7, drilling point E20, layer of clayey soil from the surface till the depth 5.50 m, after that layer of silty clay till depth 6.50m, then layer of silty sand till depth 9.0m , then layer of compact sand extended over depth >12.0m extended over the depth of
>12.0m.
In profile #4, borehole no.8, drilling point E25, and soil layer consisting of stiff clay, extended from the surface until the depth of 2.0 m. After that, layer of silty clay till depth 3.0m, then layer of compact sand till depth 6.36m, then layer of compact sand till depth 6.36m, then rock fragments and sand till depth 9.0m, after layer of
weathered marbleized limestone bed rock depth extended over depth >10.0m.
In profile #5, borehole no.9, drilling point E30, and soil layer consisting of stiff clay, extended from the surface until the depth of 2.0 m. After that layer of silty clay till depth 3.50m, then layer of silty sand till depth 6.0m, then layer of compact sand till depth 6.0m, then rock fragments and sand till depth 6.5m, after layer of weathered marbleized limestone bed rock depth extended over depth
>12.0m.
Through the drilling of bore holes no.10, the point E6, profile #1, Table 5 in site no.3 ( Batu cave), found the thickness of the soil layer abut 0.50m from the surface concentrations of iron oxide nodules and limestone fragments. Then layer of sand concentrations of some gravels till the depth 3.0m, then layers of silty clay and stiff clay till the depth 6.38m, after that non-stiff highly moisturized clay to the depth > 12 m.
Table 5: Viewing the description of boreholes in site #3 (Batu Cave)
In profile #2, borehole no.11, the point E32, found the soil layer extended from the surface until 0.50m concentrations with nodules of iron oxide and limestone fragments. Then layer of silty clay until the depth 3.0m. After that, layer of stiff clay to a depth > 12 m.
In profile #3, borehole no.12, the point E20, found the thickness of the soil layer about 0.50m from the surface concentrations with nodules of iron oxide and limestone fragments. After that, intact marbleized limestone rock extended over depth >12.0m.
In profile #4, borehole no.13, the point E25, found that the soil layer thickness about 0.50m from the surface concentrations with nodules of iron oxide and limestone fragments. After that, stiff clay layer until the depth of 1.50 m, then after, non-stiff clay highly moisturized extended over depth >10.0m.
In profile #5, borehole no.14, the point E15, found the thickness of the soil layer about 0.5m from the surface
concentrations with limestone fragments. After that, sand layer until the depth of 2.50 m, then after, rock fragment with and sand with thickness 0.50m until the depth of 3.0m. Then, weathered marbleize limestone rocks extended to a depth >12.0m.
In profile #6, borehole no.15, the point E30, found the thickness of the soil layer abut 1.25m from the surface concentrations with limestone fragments. After that, silty sand extended over depth >12.0m.
Through the drilling of borehole no.16, point E10, profile
#1, Table 6 in site no.4 (Petaling Jaya); found that the thickness of the clayey soil layer abut 0.75m from the surface, then layer of stiff clay until the depth of 2.75m. After that, layer of silty clay until the depths of 4.0m. Then, layer of silty sand and sand until the depth 6.38m, then rock fragment with and sand with thickness o.42m until the depth 6.80m. Then after, weathered marbleized limestone rock extended over the depth of 12.4m.
Table 6: Viewing the description of boreholes in site #4 (Petaling Jaya)
In profile #2, borehole no.17, the point E31, found thin crust soil layer abut 0.30m from the surface, then sandy gravel and rock fragments until depth of 3.0m. Then layer of stiff clay until the depth of 4.5 m. after that non-stiff clay highly moisturized extended over depth 9.0m. After that stiff clay and silty clay till depth 11.0m, then silty sand and sand till depth 13.0m, then rock fragment with and sand with thickness 1.0m till the depth 15.5m. After that, weathered marbleized limestone rock extended over the depth of >19.8m.
In profile #3, borehole no.18, the point E27, found thin crust soil layer abut 0.30m from the surface, then sandy gravel and rock fragments until depth of 4.0m. Then layer of compact sand until the depth of 8.0 m. Then layer of stiff clay until the depth of 11.0 m after that non-stiff clay highly moisturized extended over depth 15.0m. After that stiff clay until depth extended over the depth of
>19.8m.
In profile #4, borehole no.19, the point E22, found that the thickness of the soil layer abut 1.0m from the surface, Then, layer of sand till deptp.0m, then silty sand till depth 4.0m. after that, silty clay till the depth of 11.0 m then layer of stiff clay till the depth of 14.0 m. then after non-stiff clay highly moisturized extended over depth
>24.0m.
- MITIGATE THE HAZARD OF RADON GAS EMISSION IN KARST REGIONS
- IntroductionCarbonate Karst environments its geologic condition in everywhere round the planet earth. Its rocks characterizing by features containing fluids such as, cavities, sinkholes, underground streams, caves and channels pipes.
When the groundwater has dissolved a geologic formation of sedimentary rocks that located over carbonate kart environment bedrocks, high in uranium or radium,
radioactive radon gas will produce by those formations can more freely move into the houses and the buildings that located above this geologic formation. Besides, the most dangerous area in karst regions are the housing complex and high-rise building that constructed rounds these karst lakes and the mining pits that developed in the region to become lakes for tourism. The radon emission is so strong and can affect the people who stay round this lakes and create lungs problems to them.
Karst causes jaggedness; Fissures assist as conduits that channel radon gas to the surface, causing irregular flow rates and patterns of movement that can vary with the seasons and outer environmental conditions. Weather and seasonal conditions can cause spikes and dips in radon levels. The radon measurements in the internal seasonal variations are greater in karst areas than in non-karst areas.
Variability has showed in the environmental experimental study by many scientists, in the karst regions of Topical and semi-tropical regions. In a yearlong test of radon will give the best picture of actual radon level than short test in one day or in one week. The study has shown that; short-range in-door and out-doors testing for the houses and buildings round these karst sites have higher radon levels. However, there are structures in karst areas give wrong or negative results in hot morning and when tested in the night become colder give positive results or high radon problem.
In addition, in colder area, Short-term tests often show that houses have higher radon levels in colder months (November through February). However, there are houses that do test higher in warmer months (May through September). To account the variability of problems, a yearlong test will give the best picture of the houses actual radon level.
The unpredictability of studies shown that the karst has approximately one-third of the homes tested (with short-term tests) in karst areas in the summer time had
(False) negative results and when tested in the winter had a radon problem.
- The Hazard of Radon Gas Emission in Karst AreaRadon also is a potential health hazard in karst areas. The accumulation of radon gas beneath the ground floors of buildings can be harmful to humanity. Particularly those who existing adjacent to rock types which are relatively rich in radon and where open fissures exist such as karst lake.
In an area where the limestone is closely to a granite body, the radon problems can improve by ventilation. Distinctive plants associated with karst features which their life cycle or at least part of it depending on upon the distinctive environment of these systems. Even slight alterations or disturbances can have significant impacts upon these plants.
The buildings and housing complex that constructed in karst regions contaminated with greater levels of radon and other contaminants that affect the breathing and present in air within the buildings and housing complex in this regions. It will contaminated with radon of two pico- curies per liter (2 pCi/l) or the greater levels four pico- curies per liter (4 pCi/l) and other contaminants that affect the breathing and present in air within these housing and buildings. These contaminants include hydrocarbons, CO, CO2, ozone, olefins, and nitrogen oxides, sulphuric. Many areas with karst bedrock are composed of limestone; phosphate minerals that occur naturally in limestone contain small amounts of uranium. Uranium is a radioactive, toxic element that easily oxidized.
Because of the uranium degrading into lead, small amounts of radon are produce as a byproduct. The dissolved rock on top of a geologic formation is high in uranium or radium, radioactive radon gas produced by those formations can more readily move into houses and buildings above. Over a period, this radon gas can accumulate within solution-derived through Joints naturally occurring in the limestone bedrock that allow for fissures and cavities to form through solution processes. Over a period of time, and heavy rain events this radon gas can accumulate within solution that derived through these fissures and released into water reservoirs beneath the following surface, (Savoy, L. et. al. ,2011).
The structures that built above karst cavities are likely to diffusion of radon due to fluctuations in atmospheric pressure (Ruthven, C. et. el., 2002). Extensive amounts of radon are produce with in the soil overlying karst bedrock. This radon is release into water reservoirs beneath the surface after heavy rain events (Savoy, L. et. el, 2004).These potential geologic hazards can identify by geologists in order to mitigate the risks that are present by the karst bedrock.
A short-range test often shows that the houses have higher radon levels. However, there are houses that do test higher in a yearlong test will give the best picture of actual radon level. A karst variability study has shown that approximately one-third of the homes tested (with short- term tests) in karst areas is (false) negative results. In addition, when make (with long-term tests), they had a radon problem.
Radon infiltration is a problem to taken seriously. Prolonged exposure to the gas can result in serious health problems and even death. The radon gas is an environmental hazard associated with areas of karst topography, is known as deadly gas cause of lung cancer. These steps involving get in touch of indoor air stream with an adsorbent device for the removal of radon. Additionally, when design of building and housing structures needs development of new process to remove of radon from out- door, in-door air stream and other contaminants. Levels process greater than 4.0 pico curies are cause alarm. Houses in karst area with this category, such as allow for more ventilation of basements and crawl spaces in the houses, as well as sealing crawl spaces with plastic. The geophysical techniques viewing that radon gas existing in the water that in-fill the sinkholes and the cavities in most ER Tomography section of the sites under study. This led to conclusion that there is an effect and put the solution in these sites. ERT Sections from construction site #1 , site# 2, site#3 and site#4, displays the radon (pink colour, 1-3 ohm-m) in the underground water of the cavities and the sinkholes present in figure 24.
Radon infiltration is a problem to taken seriously. Continued exposure to the gas can result in serious health problems and even death. Radon is the second leading cause of lung cancer variability in radon concentrations within individual homes. This variability seemed related to differences between ground and outdoor air temperatures, and the ability of these temperature differences to move air through caves and channels of the underlying karst geology.
This air movement can alternately infuse the soils around a building with radon from the earth. Changing the concentration of radon in the soil gas surrounding a building can dramatically change the indoor radon levels in a matter of hours. Short-term radon measurements are not reliable indicators of the long-term radon concentration in buildings in karst areas. An agreement of new testing procedure was developing that (recommends 12-month testing in karst areas, warns against using shorter term testing, and calls for periodic retesting). Similar situations have since been identifying in both Canada and Europe.
Site #1, figure (19 – C): The Inverse model of electrical resistivity section for profile#3
Site #2, figure (20 – B): The Inverse model of electrical resistivity section for profile #2
Site #3, figure (21 – C): The Inverse model of electrical resistivity section for profile #3
Site #3, figure (21 – D): The Inverse model of electrical resistivity section for profile #4
Site #3, figure (21 – E): The Inverse model of electrical resistivity section for profile #5
Site #4, figure (22 – B): The Inverse model of electrical resistivity section for profile#3
Site #4, figure (22 – D): The Inverse model of electrical resistivity section for profile #4
Figure24: ERT Sections from selected sites under study; site 1, site2, site3 and site4, displays the radon in the underground water of the cavities and the sinkholes
- Preventing the Radon Gas From Enters Into Our HousesThe radon gas is an environmental hazard associated with areas of karst topography, is a known deadly gas cause of lung cancer. Radon is a radioactive gas. It comes from the natural decay of uranium that is founds in nearly all soils. It typically moves up through the ground to the air above and into the house through cracks and other holes in the foundation. The house traps radon inside, where it can build up. Any house may have a radon problem, new houses and the olds, well-sealed and draft homes, and homes with or without basements. Radon from soil gas is the main cause of radon problems. Sometimes radon enters the home through well water. Many studies discover that in some homes, the building materials can emit radon also. Radon gets into the homes or the buildings through the cracks in the solid floors and in the walls, construction joints, gaps in suspended floors, gaps around service pipes, cavities inside the walls, and the water supply.
Uranium can generate radon gas by fission. The radon gas will itself undergo further fission to produce radioactive (daughters). The alpha particles produced by radon daughters are not powerful enough to penetrate the human body from outside it. However, if radon draws in, the alpha particles generated by the daughters can cause harm to cells in the lungs and in a different place. This may result in an increased risk of cancer. The level of radon is often highest in the lower part of the building. Radon moves through the homes by diffusion and natural air movements and it can be distribute by mechanical equipment, such as a forced-air ventilation system. As radon moves away from the house’s foundation or other entry points, it combinations and is diluted into a greater volume of air.
In addition, more reduction often occurs in the upper levels of the home because there is more fresh air ventilation there. Greater reduction and less home vacuity may also occur when the home is more open to the outdoors during the non-heating season. The gas exposure in houses could be reducing through impermeable casing could install at floor level. In addition, fans could suck air- containing radon from underneath the ground floor and expel it directly into the atmosphere, where it goes away (Agius, R., 2009).
- Impact of Radon GasRadon is present approximately in entirely air. Usually, everyone breathes radon in every day at very low levels. However, people who breathe in high levels of radon are at an increased risk for developing lung cancer. Radon deteriorations quickly, giving off tiny radioactive particles. When breathe in, these radioactive particles can damage the cells that line the lung. Long-term exposure to radon can lead to lung cancer, the only cancer proven associate with breathe in radon. According to Yamada (2003), the radons half-life of 3.8 days is long enough for it to enter into indoor and cause an increase in the indoor concentration. However, this half-life is relatively too long to enter into the breathing territories and to expose the cells.
Radon gas that appear from the cracked in the buildings could affected the health of human. Radon cited as the second leading cause of lung cancer after cigarette smoking. This study is important, as it will be beneficial to people who live in karst area to understand more about radon and its effect to human health.
Radon-222s half-life of 3.8 days is long enough for it to enter into indoor and can cause the indoor concentration to increase but it is too long for it to enter into the breathing zones and to treat the cells. Poor indoor air quality in homes and office buildings has recognized as a primary factor that leads to lung problems in people. Radon is a primary contributor and levels above four pico-curies per liter (4 pCi/l) have statistically shown to increase the risk of cancer in personalities. Radon gas, which is a radioactive decay by-product of radium, constantly seeps from subterranean radium deposits and out through the surface of the earth’s crust, into the atmosphere surrounding the earth. It has a relatively short half-life, in the order of four days.
Thus, the radon gas concentration never reaches significant levels upon escaping into the earth’s atmosphere. Radon gas does not reach sufficient concentration levels, e.g., above (4 pCi/l) to pose a human health hazard. When an enclosed structure, such as a building, is located in an area of significant radon contamination, the radon seeps into the structure through cracks or other openings in parts of the foundation, basement or other parts of the building that are in contact
or near contact with the earth. If there is a poor exchange of interior and exterior air within the building, significant radon concentrations can develop within a building, which are above current limits (4 pCi/l).
- The Physical Properties of Radon
- IntroductionCarbonate Karst environments its geologic condition in everywhere round the planet earth. Its rocks characterizing by features containing fluids such as, cavities, sinkholes, underground streams, caves and channels pipes.
-
Radon is a chemical element with symbol Rn and atomic number 86. It is a radioactive, colorless, odorless, tasteless, noble gas, occurring naturally as a decay product of radium. Its most stable isotope, 222Rn, has a half-life of
3.8 days. Radon is one of the densest substances that remain a gas under normal conditions. In addition, the only gas under normal conditions has radioactive isotopes and is consider as health hazard due to its radioactivity.
Because of that gas specification, therefore not detectable by human senses alone. At standard temperature and pressure, radon forms a monatomic gas with a density of 9.73 kg/m3, about 8 times the density of the Earth’s atmosphere at sea level, 1.217 kg/m3. Radon is one of the densest gases at room temperature and is the densest of the noble gases. Although, colorless at standard temperature and pressure, when cooled below its freezing point of 202 K (71 °C; 96 °F), radon emits a brilliant radio luminescence that turns from yellow to orange-red as the temperature lowers. Radon radiances because of the intense radiation it produces. Radon is sparingly soluble in water, but more soluble than lighter noble gases. Radon is noticeably more soluble in organic liquids than in water. Being a noble gas, radon is chemically not very reactive. However, the 3.8-day half-life of radon-222 makes it useful in physical sciences as a natural tracer.
Radon is members of the (zero-valence elements) that are call noble gases. It is inert to most common chemical reactions, such as combustion, because the outer valence shell contains eight electrons. This produces a stable, minimum energy configuration in which the outer electrons are tightly bound.1037 kJ/mol is required to extract one electron from its shells (also known as the first ionization energy).
In accordance with periodic trends, radon has a lower electronegativity than the element one period before it, xenon, and is therefore more reactive. Early studies concluded that the stability of radon hydrate should be of the same order as that of the hydrates of chlorine (Cl2) or sulfur dioxide (SO2), and significantly higher than the stability of the hydrate of hydrogen sulfide (H2S).
Because of its cost and radioactivity, experimental chemical research is seldom perform with radon, and as a result, there are very few reported compounds of radon, all either fluorides or oxides.
Powerful oxidizing agents such as fluorine, thus forming radon di-fluoride, can oxidize radon. It decomposes back to elements at a temperature of above 250°C. It has a low volatility and thought to be RnF.
It has not possible to study of radon and the radioactivity of its compounds, Because of the short half- life. The theoretical studies on this molecule predict that it should have a Rn-F bond distance of 2.08 Ã…, for that the compound is thermodynamically more stable and less volatile than its lighter counterpart (XeF). The octahedral molecule RnF6 has predictable to have an even lower enthalpy of formation than the di-fluoride. The higher fluorides RnF4 and RnF6 have claimed, and are calculate to be stable, but it is unsure whether they have yet been produce. The (RnF) + ion have considered forming by the following reaction:
Rn (g) + 2 (O2) + (SbF6) (s)
(RnF) + (Sb2F11) (s) + 2 O2 (g)
-
- The Development of Process for Removal of Radon GasThis development provides a process for removal of radon and/or other contaminants from indoor air comprising the step of contacting of indoor air with an adsorbent, adsorbent comprising a silver-exchanged zeolite. In another representation of the process, other impurities, which may affect breathing, are sometimes present in air within buildings and these include hydrocarbons, CO, CO2, ozone, olefins, nitrogen oxides, sulfur oxides, water and microorganisms.
These impurities can remove, in addition to radon, if present, via a layering of adsorbents that are design for selective removal of the impurities within the adsorption system. One refinement is providing a process for the removal of impurities from indoor air contaminated with radon of 2 pCi/l or greater. Within a building comprising the steps of: contacting the indoor air stream with a porous material which releases silver ions upon contact with water for removing bacteria and molds; contacting the indoor air with a desiccant under conditions for removing moisture; and, contacting the indoor air with a silver exchanged zeolite capable of removing radon.
The process and device provide an ability to remove radon from indoor air in buildings; which may be competent at generally ambient conditions if desired. Using of an adsorbent having an affinity and capacity for radon. Radon is substantially or partially removed from the moisture-free air by passing the moisture-free air through the absorbent comprised of (Ag exchanged zeolite) to create (cleaned air), and is returned to the indoor air amosphere. The manifestation (cleaned air) describes as, the air stream that occurs by the absorber.
Radon formed as one intermediate step in the normal radioactive decay chains through which thorium and uranium slowly decay into lead. Thorium and uranium are the two most common radioactive elements on earth; they have been around since the earth formed. Their naturally occurring isotopes have very long half-lives, approximately billions of years. Thorium and uranium, their decay product radium, and its decay product radon, will therefore continue to occur for tens of millions of years at almost the
same concentrations as they do now. As radon itself decays, it produces new radioactive elements called radon daughters or decay products.
Unlike the gaseous radon itself, radon daughters are solids and stick to surfaces, such as dust particles in the air. If such contaminated dust inhaled, these particles can stick to the airways of the lung and increase the risk of developing lung cancer.
Different to all the other intermediate elements in the aforementioned decay chains, radon is gaseous and easily breathes in. Thus, naturally occurring radon is responsible for the majority of the public exposure to ionizing radiation. It is often the single largest contributor to an individual’s background radiation dose, and is the most variable from location to location. Despite its short lifetime, some radon gas from natural sources can accumulate to far higher than normal concentrations in buildings, especially in low areas such as basements and crawl spaces due to its heavy nature. It can also found in some spring waters and hot springs.
- The Potential Entry of Radon Gas in the Houses: The potential entry points of Radon gas in the housing construct over Carbonate Karst environment is:
- Cavities inside the walls
- Cracks in the footing and solid floor 3- Construction joints
- The Development of Process for Removal of Radon GasThis development provides a process for removal of radon and/or other contaminants from indoor air comprising the step of contacting of indoor air with an adsorbent, adsorbent comprising a silver-exchanged zeolite. In another representation of the process, other impurities, which may affect breathing, are sometimes present in air within buildings and these include hydrocarbons, CO, CO2, ozone, olefins, nitrogen oxides, sulfur oxides, water and microorganisms.
- Cracks in walls
- The water supply pipes
- Cracks round surface pipe 7- Cracks in suspended floor 8- The floor drain
The potential entry points of Radon gas in a home construct over Carbonate Karst environment and Gas emission purifications instrument display in figure 25.
Figure25: Display the Potential Entry Points of Radon Gas in a Home Construct over Carbonate Karst environment and the Design of Gas Emission
Purifications instruments by (Yassin,R. R.,2012)
- GEO-TECHNICAL TABLE FOR ENGINEERING CLASSIFICATION OF KARST GROUND CONDITIONSThe first engineering classification of karst ground conditions present by A. C. Waltham and P. G. Fookes, (2005) which describe simplified guidelines to the potential variation in landforms and the characteristics of the morphological features and ground cavities that possibly come upon in civil engineering works on karst terrains. This classification divides the Karst terrains in planet earth into five classes. The five classes illustrate the karst terrains in terms of the difficulty and complexity that come across the foundation engineers. In these classifications, the
carbonate karst regions in Malaysia classified as extremely karst terrains.
Through the study of karst regions found that the strength of karstification are different from one site to another in same region. A geo-technical table for engineering classification of karst ground conditions which presented by Yassin, R.,R. &Almashaiky, D. (2005). This table classifies the karst terrains in desert and semi desert regions to five classes after discovering complex and extreme subsurface karst through applying of geotechnical survey. After that this table developed to classify the karst in semi wet and wet tropical region presented by Yassin,
- R. & Haji, Taib, S. (2012) which describe the subsurfacefeatures that come upon in engineering works on karst terrains, after applying of geotechnical survey.
This table applies in Malaysia peninsular and includes five (5) classes, start from Youthful karst (KaI) and ending with extremely complex karst (KaV) as show in the following:
- KaI: Youthful karst:Found only Infrequent sinkholes, with unvarying and even rockhead pinnacle, certain of minor and isolated remnant features, rare cavities, caves are rare and small, with several sizes less than 3.0m across.
- KaII: Adult karst:Small dripping out sinkholes, small open stream sinks, small features and cracks are widespread within few meters nearest to the surface, small cavities, various type of small caves with size greater than 5.0m across.
- KaIII: Mature Karst:Numerous types of medium dissolution and subsidence sinkholes, wide distribution of medium collapse and buried sinkholes, pinnacles release with tall 5.0 -20m across, loose pillars, medium- range of dissolution depresses and fractures, Medim cavities, Caves with several sizes, greater than 15.0m across.
- KaIV: Complex Karst:Numerous types of large dissolution sinkholes, various types of large subsidence or collapse sinkholes, numerous types of large spread buried and collapse sinkholes, large of relief rockhead Pinnacles, wide-range of dissolution depresses and major fractures, large cavities, multilevel caves, with several sizes greater than 50.0m across.
- KaV: Extremely Complex:
Extra-large sinkholes of all types, extra-large buried sinkholes in-fill with compact sediments; greater of relief rockhead pinnacles, deep soil fissures, complex dissolution cavities, numerous type of complex cave systems with chambers and galleries, caves with size greater than 100.0m across.
In accordance to the above classification of karst ground conditions that presences of the morphological features, the karst level in site#1, Tasik University Malaya observed in the ERT sections between profile # 1 and profile# 4 is an older or mature karst type KaIII due to presence deep Gutter or channel extend between pinnacles from profile#1 to profile#4. Furthermore, mature buried sinkholes observed in the sections of profiles #2 & #3.
The karsts level in site #2, Jalan Perlimen, observed in the ERT sections between profile # 1 and profile #2 is a complex karst KaIV due to presence mature buried sinkholes, cavities and relief pinnacles. Also, observed in
the ERT sections between profile # 3 and profile #5 deep gutter and caves.
In addition, the karsts level in site#3, Batu Cave observed in the ERT sections between profiles #1 and profile #6 is a complex karst type KaIV due to presence underground channel fill with sediments, buried sinkhole extend between pinnacles from.
Finally, the karst level in site#4, Petaling Jaya, observed in the ERT sections between profile #1 and profile #4 is older or mature karst type KaIII due to presence many pinnacles and various type of buried sinkholes.
APPLYING OF ENGINEERING SURFACE AND SUBSURFACE REMEDIATION TECHNIQUES
In the past decades, Tin ex-mining activities were widespread over the limestone formation of Kuala Lumpur and Selangor. These activities left behind a numerous of ponds and holes in-fill with rock fragments consisting of sand and clay slime, forming a highly various overburden on the limestone. Many ex-mining areas have become garbage dumping grounds and thus making the overburden material above the limestone more complicated.
Due to rapid development in the states the land is getting limited, previously ex mining, areas out of control become prized lands for developments. Conventional pile driving and bored piling come across doubts and difficulties due to various nature of the overburden materials and irregular Karstic features of limestone.
An overview of surface and subsurface remediation procedures that can use with the integrated techniques in site characterization will discuss. This include the application of these processes and its strategies which resulting in maintenance, monitoring the karst features, the costs and the timing of remedial solutions that can affect the success.
Geological and geotechnical Surface and Subsurface site characterization, modeling, monitoring, and remediation are important to hazard mitigation, economical safe, responsible development, operation of remediation and maintenance works. Remediation methods, considered as chemical or physical, are covering to provide wide- ranging overview for remediation. It has planned to provide in-depth detail for any one technique, to serve as initial supervision for remedy selection choices.
Several problems faced the geo-engineers and civil engineers when constructing in carbonate karst environments, such as the settlement of footing, length of piles, sinkholes problems and the effect of earthquakes and heavy Rains in creating the sinkholes, determination of sinkholes, voids, cavities and caves. Engineering subsurface remediation techniques are available in repairing any future construction site threatened by karst features such as, cover-collapse sinkholes, dissolution sinkhole, subsidence sinkhole, channel pipes, cave and cavity.
The types of foundation for any construction project over carbonate karst regions depend upon the expected foundation loading and the degree of maturity of the karst features. The greatest danger to the site is when foundations are positioning over sinkholes. In this case, two additional factors need to take into consideration; the overloading aspect and water seepage into sinkholes through the soil cover. The most significant challenge is monitoring of fluids paths and velocities that seepage from surface and travel through the sinkholes and subsurface fractures.
If the level of karst ground condition under construction site has known and classified, the most economical point of view in developing this site is to mitigate or minimize the risk of structures that has found over the area by; involves determining of the safest direction in changing the plans location. If possible, the most important sections and those with great size constructions structures has to be placed in the safest region, while the problem areas can be allocated for non- critical facilities, such as grass field, parking lots, golf courses and roadways etc. Controlling the surface and subsurface water drainages must put in the plans when the work is commencing at these respective sites.
-
- Sinkhole Remediation TechniqueThe term sinkhole is a depression in the ground caused when ground water erodes the subsurface rock and allowing the clay above it to sink. These holes its ranges in various size mainly from about 0.9 m to more than 23.0 m wide and its depth ranges mainly from 1.0 meters to more than12.0 meters.
Begin the process of sinkhole repair by testing the width and depth of the sinkhole through the geophysical section. If the hole is very large, the best method of professional sinkhole remediate if can is to excavate to the bedrock and re-fill or reinforce as needed. Repair methods are varied, and the method used depends largely on finances and the location of the sinkhole. The method and materials needed to fill in a small sinkhole can be quite different for those needed to repair a sinkhole in a construction site over a highly karstic carbonate area. Repairing tools and material vary to a great extent. The two primary goals of sinkhole repair are, the stabilization of the area and the prevent re-appearance.
In addition, the best method of sinkhole repair varies with the size and stability of the hole. For a smaller hole
with compact ground at its lowest level, replacing soil and repairing ground cover will be enough. A sinkhole with very large holes with unstable sides or low points where that can excavate it to the bedrock and can repaired by layering varying sizes of stone topped by gravel and soil as in following.
If the size of sinkhole is large, the first stage of sinkhole remediate includes excavating the hole and its throat plugged by concrete block. The second stage filling the sinkhole with gravels and stones by utilizing the reverse graded filter technique. The third stage, on top of the layered stone, places a synthetic construction fabric, also known as a geotextile. The fourth stage, fill the empty spaces by spray with geopolymer solution. The fifth stage covers the surface with thin layer of bentonite clay then thin layer of soil to prevent the water seepage. The sixth stage rolling and compaction processes to increase the stiffness of the surface soil. Sinkhole remediation by utilizing of the reverse graded filter technique is one of engineering subsurface remediation technique presented in figure 26.
At a less imperative way rapidity can be use is in filling the sinkhole by applied of reverse graded filter technique. This way will allow water to seep into the ground while the material has held back in the hole. In the case of great sinkholes, the graded filter construction is essentially the same, but the final layers are fine gravel, coarse sand and fine sand. The upper most layer of bentonite clay that blocking the water seepage.
A smaller soil cover collapse sinkhole in a site of about 0.9 m. to 1.80m wide and depth ranging from 0.9 m to 1.8 m with a solid base and sides can usually be repaired by adding soil and rock fragments. Repeat that process until the packed of soil has filled the hole to surface level. If ground cover was lost again, it should be adding more and re- fixed. While the sinkhole repair in a site with a large hole of about > 1.80m to less than 10.0m wide and depth ranging from 1.80 m to less than 10.0 m need to excavate the hole and covering the bottom of the excavated hole with a layer of stones about the size of cabbages. On top of these stones, place a layer of smaller stones, about the size of billiard balls. Fill in the layers of stone with a layer of gravel.
Figure 26: Diagram section presenting the sinkhole remediation by utilizing of the reverse graded filter technique is one of engineering subsurface remediation
technique
On top of the layered stone, place a synthetic construction fabric, also known as a geotextile, over the gravel. This powerful, stretch-resistant fabric will help prevent layers of sand and soil from washing away through the stone layers. Layer sand on top of the construction fabric and layer soil on top of the sand to ground level.
It is better to construct the plug or the drain stopper directly at the contact with the bedrock drain to providing a better seal to stopping additional loose soil material, settlement or sinking from flushing down into the fissure in the bedrock that returns water to the aquifer. During repair, the sinkholes triggering mechanism must be address in order to minimize additional soil settlement or sinking. The water is generally the primary cause mechanism, it is necessary to identify and remove the problem to the degree that the possible source of the water that may have caused the sinkhole.
Grate or massive sinkhole remediation can start up by using of concrete bridge beam in order to transfer the load of constructions to the both sides of the massive sinkhole. The sinkhole remediating by applying of concrete bridge beam is one of engineering subsurface remediation technique presented in figure 27.
Sinkholes in which no bedrock is encountering, and where the soil cover is thick, require careful geologic analysis followed by various costly repair methods, ranging from pressure grouting or penetration grouting in the subsurface, to driven piles having concrete caps and gravel-mat backfilling.
Figure27: presenting the Sinkhole remediation by using of concrete bridge beam is one of engineering subsurface remediation technique
- Deep Injection and ProcessDeep injection and process gives long-term stability to the problematic area affected by karst features. Its a combination of chemical, compaction and permeation grouting that will not cause any environmental amage, stabilizes the soil, can fill and seal the channel, and can cover the channel pipe, filling the grate cavities, small voids and micro-fissures because geo-polymer have expansion characteristics.
Deep injection is a combination of chemical, compaction and permeation grouting that will not cause environmental damage, stabilizes the soil at perimeter of the throat, can fill and plug the throat, and can cap the soil pipes, filling the voids and micro-fissures within the soil at the injection site. The geo-polymer is the key factor in the repair. While traditional chemical grouting fills the soil cavities and pores with a waterproof material, it does not have expansion characteristics of the geo-polymer process. When comparing between deep injections methods with traditional repair methods determine there are many challenges associated with sinkholes and the traditional methods that has used to repair them:
- Heavy machinery is often required to repair sinkholes.
- The equipment can require a lot of work force to get it to the site and to operate it.
- A wide variety of materials and mixes has typically needed for a repair, including concrete, soil, grout, synthetic filter fabrics, and various sizes and mixtures of crushed stone.
- Injection holes for the repair materials are large and very invasive.
- High pressure is required to pump the heavy grout to the location of the repair.
- There is no expansion strength in the repairing materials used, so over lift occurs to compensate for any shrinkage that may occur.
- More water has introduced when using a cementations grout adding more weight (approximate of 135 lbs. per cubic foot) to already distressed soils.
- Deep Injection Technique is one of the best ways to repair a shallow sinkhole, because severe structural damage can result from shallow sinkholes, immediate and conclusive sinkhole repair is critical.By injection of expanding geo-polymer, resins applied for soil stabilization that is an engineering procedure, where by the bearing capacity of foundation soils may continuously improved down to reach considerable depths, or just at a specific depth. Chemical Grouting – is a form of permeation grouting which is an efficient and cost- effective grouting technique which using grout to fill small voids in the soil with chemical solutions. Solution grouts commonly used include acrylamides, polyurethanes, acrylates, epoxies, and sodium silicates. There are two major types of chemical grouting: structural and water control. The deep grouting by injection of chemical solution forms is one of engineering subsurface remediation technique presenting in figure 28.
Figure28: Diagram section presenting the deep grouting by injection of chemical solution forms is one of engineering subsurface remediation technique
- The Surface Remediating TechniquesThe soils best suited for this technique are granular soils, with significant fine sand content, to strengthen the ground and prevent excessive movement. Chemical grout is force through joints and gaps in the surrounding soil where it solidifies with the soil to form relatively impermeable mass. When it is injecting into cracks, the solution grout forms a waterproof bond and could offer a less expensive method to seal joints and fractures.
The surface remediating techniques includes the surface cover, soil stabilization by using of Bentonite/ Cement grouting compaction technique and this can be utilize to fill the small voids and fissures in the soil. This technique is best appropriate for the granular silty, sandy
and clayey soil with significant fine sand content that strengthen the ground and prevent excessive movement in the future. It has solidified with the soil to making it appear relatively impermeable and hard. The Soil stabilization by using of Bentonite/ Cement grouting technique is one of engineering subsurface remediation technique present in figure 29.
The geopolymer liquefaction mitigation is a technology to reduce the risk of earthquake caused liquefaction phenomenon under existing structures. its improves the situation by causing cementation through chemical binding of sand particles, providing cohesion to the soil, increasing in-situ stresses in the surrounding soil due to volumetric expansion and compaction.
Figure29: Diagram section presenting the soil stabilization by using of Bentonite/ Cement grouting technique is one of engineering subsurface remediation
technique
Chemical grouting equipment is well suited for tunneling applications in urban environments, either for stabilizing soil around break-ins or breakouts, or for mitigating settlement of overlying structures within the influence of the tunnel alignment. Is commonly can used for water control as a cost-effective process to stop leaks in the tunnels, mines, underground tanks and pipes, and around underground conduits. The work may getting done via the interior or exterior of a structure, depending on access constraints. Grout has injected in drilled holes along the flow paths, sealing and preventing flow through them.
Geopolymer pillar is a Power Pile, which is a distinctive solution to ground improvement and stabilization. It is especially suitable for treatment of weak foundation soils suffering from settlement or subsidence. The result is a dramatic improvement of the foundation soil and increased load bearing capability. The geopolymer materials have used in special engineering treatment, but it retains the same basic physical properties and resistance as all the geopolymer materials. It is long lasting and well
proven. Pillars can available to lengths up to 25 meters and are individually designs to the needs of every project.
Power Pile Polymer Pillar effects foundation soil due to the following factors, soil replacement, soil compaction, load transfer, skin friction, cohesion and base resistance. It can be installed directly beneath concrete floor slabs and building foundations. It is perfect for difficult admission because no large piling rig required. In addition, the technique has minimal disruption, noise, dust, or confusion. It does not require load transfer structures. The procedure has carried out in the following phases:
First, drilling of 30 – 50 mm hole and installing a flexible expansion element.
Second, inject of the expanding geopolymer inside the flexible expansion element.
- The Type of Piles That Frequently Must EmployedIn the study region the type of piles that had often employed with the respect to the effect on the soil are driven piles. Driven piles are considers displacement piles. In the process of driving the pile into the ground, as the pile
shaft enters the ground the soil is move radially. There may also be a component of movement of the soil in the vertical direction. Piles with respect to type of material that used are concrete piles and can be dividing to pre-cast concrete piles. Pre- cast concrete Piles or Pre-fabricated concrete piles it is forms and reinforced in a high-quality controlled concrete. Usually used of square, triangle, circle or octagonal section, they are produce in short length in intervals between 4.0m and 6.0 m.
The risk of the problem area also can minimize by usage of piles with different exhibition. The classification of piles with respect to load transmission and functional behaviour is:
– End bearing piles (point bearing piles)
– Friction piles (cohesion piles)
– Combination of friction and cohesion piles
End bearing piles; are the types of piles that transfer their load to a stable stratum located at a considerable depth below the base of the structures, and then derive most of their carrying capacity from the penetration resistance of the soil at the toe of the pile. The piles behave as an ordinary column and should design as such. Even in weak soil a pile will not fail by buckling and this effect need ony be considered if part of the pile is unsupported, i.e. if it is in either air or water. The load is transmitting to the soil through friction or cohesion. However, sometimes, the soil surrounding the pile may adhere to the surface of the pile and causes “Negative Skin Friction” on the pile.
This, sometimes have considerable effect on the capacity of the pile. Negative skin friction caused by the drainage of the ground water and the consolidation of the soil. The founding depth of the pile is influenced by the results of the site investigate on and soil test. End bearing piles driven down to the bedrock strata present in the diagram section in figure -30. In carbonate karst regions under study the length of piles is not similar due to the dissimilarity in bedrock depth.
Uneven subsurface karst topography and presence various size of pinnacles and gutters. In addition, presence of hanging layers, cliffs, floating, these karst topography features need to investigate by geophysical technique to
imaging the real depth of carbonate rock such as marbleized limestone or dolomitic limestone.
The other type of piles is Friction or cohesion piles. These piles also transfer their load to the ground through skin friction. The process of driving such piles does not compact the soil appreciably. These types of pile foundations have commonly known as floating pile foundations. Carrying capacity is deriving mainly from the adhesion or friction of the soil in contact with the shaft of the pile. These piles transmit most of their load to the soil through skin friction. This process of driving such piles close to each other in groups greatly reduces the porosity and compressibility of the soil within and around the groups.
Therefore, piles of this category are sometimes calling (compaction piles). During the process of driving the pile into the ground, the soil will become molded as a result loses some of its strength. Therefore, the pile is not able to transfer the exact amount of load that it is intend to immediately after it has driven. Usually, the soil recovers some of its strength three to five months after it has driven. Friction piles driven down into the unconsolidated layers present in the diagram section in figure 31.
In carbonate, karst regions under study the presence of thick unconsolidated layers reach a depth more than 45.0m in some sites by using drilling investigation, such as clay soil, silty clay and silty sand and sand. This layers low cohesion to more than 20m depth due to present of soil pipes and cavities and internal channels. In this case the depth in these sites need to investigate by geophysical technique to imaging the real depth of these layers and these features to know the length of pile need to use in these sites.
The combination of friction piles and end bearing pile need when the bearing stratum is not hard, such as non-stiff clay. An extension length has needed to lead the piles driven, far enough into the lower material to develop adequate frictional resistance. A farther variation of the end bearing pile is piles with enlarged bearing areas. This achieved by forcing a bulk of concrete into the soft stratum immediately above the non-stiff layer to give an enlarged base.
Figure 30: diagram section present the End bearing piles driven down to the bedrock strata
Figure 31: Diagram section present the Friction piles driven down into the unconsolidated layers
- The Bored Pile TechniqueTo support high building producing heavy vertical loads another type of reinforced concrete pile used is bored pile. Which is a (cast-in-place concrete pile) have to cast on the construction site by using bored piling machine that has specially designed drilling tools, buckets and grabs. Its using to remove the soil and the rocks. Normally, it can be drilling into 50 m depth of soil. The advantage of bored piling is the drilling method produces lower noise level and little vibration.
This drilling method is depending on the soil condition. Soil investigation must do by the piling contractor so can decide which the correct drilling technology has to be carrying on. Also, to minimizing of the disturbance of the surrounding soil. For cohesion-less soils such as sands, gravels, silts, etc., whether its under the water table or not, the pile bore hole must be supported using steel casing or stabilizing mud such as bentonite suspension. After these, reinforcement bar will put into the borehole and concrete will be pour into the borehole.
The bored pile construction method include in the first stage staking out and referencing of the proposed pile location, checking of pile center location and the reference scale. Second the pre- poring using drilling
bucket, then driving of temporary steel casing by gravity, checking of the inclination<1:100 and deviation10m. After that drilling below the casing, polymer based bentonite add, bucket, new bentonite, sonic logging test, installation of first and second rebar Gages, then if need third rebar Gage, after that platform and tremie pipe. Then the placing of good quality fresh concrete and slump test, used of sounding method, next, the tremie pipe removed, as well the gradual extraction of temporary case, final, is cleaning of the area.
The main advantages of bored piles over other types of piles are hat the variable lengths can extended through soft swelling or compressible soils, into suitable bearing material. Piles can extend to depths below seasonal moisture variation. Large excavations and subsequent backfill will reduce. In fig no. 32, photographs and diagram section present the stages of bored pile driven down to the bedrock strata. A modified rock- coring tool used for bored pile construction in limestone areas. Assists tool such the casing used to penetrate into the rock strata to the required depth and therefore prevents problems such as the collapse of loose soil surrounding the bored hole.
Figure 32: photographs and diagram section viewing the stages of bored pile driven down to the bedrock strata in Kuala Lumpur
In Malaysia Bored piles generally used for high-rise buildings. The size of bored piles has been installing ranging between 600mm, 1500mm, to maximum 3000mm diameter. In some construction sites the presence of thick overburden layers more than 60m. The bored pile machines have difficulty to reaching the required depth in this case the barrettes has used, Yee & Yap, (1998). In addition, due to the highly variable ground conditions in limestone areas that require significant input from site personnel and in addition to good geotechnical design, the observational approach stay adopted recommend for bored pile construction in limestone areas. Any unexpected geological formations, uncertainties to detect, and changes to the design can make immediately to ensure safe and cost effective design. Therefore, foundation construction in limestone areas expected to involve considerably more input from the designer during the construction stage as compared to other less complicated geological formations. Due to large differences in pile, length caused by irregular bedrock profiles, etc. which should be in place during the design stage such as criteria for compensation piles. In other mean, in Malaysia, the construction method for bored piles in limestone areas must modified to ensure proper formation of the piles.
- The Jacked-In Piles Technique
- Sinkhole Remediation TechniqueThe term sinkhole is a depression in the ground caused when ground water erodes the subsurface rock and allowing the clay above it to sink. These holes its ranges in various size mainly from about 0.9 m to more than 23.0 m wide and its depth ranges mainly from 1.0 meters to more than12.0 meters.
The jacked-in piles methods in limestone formations in Malaysia limestone have been installs with varying degree of success. Jack-in piling is a hydraulic system, is modern technique, by which pre-formed piles. Different sites required different size of piles depending in the condition and size and available space of the site such as Spun piles, precast standard RC square piles, H-piles and steel pipe piles, which are hydraulically jack into the ground as displacement piles.
Various problems such as rotation, distortion, extreme tilting, deflection, cracking,crushing, buckling, bending, etc. this may be leading to high percentage of damaged piles could be expected if insufficient attention is given during the design and construction of the piles in limestone foundations. Some design characteristics related to driven and jacked-in piles in limestone areas are as sloping bedrock or steeply inclined bedrock surface, floating boulder and cavity. Construction procedures required to carry out Jack-In pile works, including material and equipment required for the installation of pile. The details of the procedures contained possibly reviewed occasionally and modified based on actual requirement. The pile has to installed will be of a specified size by jacking in through overlaying soil to the bedrock strata. The setting out the pile positions would be where every pile position is pre-survey by the land surveyor with reference to the grid lines. The pile position will pegged using a mild steel bar.
The jacking method for the piling machines YZY 800T, YZY 600T, YZY 380T & YZY 250T including
insert of pile into the Jack-In system clamp by using the on-board crane. Clamped the pile and detach the crane cable. Final vertical checking and positioning, through moving in the X and Y directions. Commence jacking pile by applying jacking force onto the clamp device to press down the pile. YZY 100T with the pile slotted to the jacking helmet, lower the pile to position where the pile toe almost reach the ground when the pile is free from the platform. Final vertical check and position the pile. Commence jacking pile by operating the jack cylinder to press down the pile. Pile jointing is necessary then the pile will joined in accordance to conventional method or by way of MIG / CO2 welding. The installation process of pile is jacked into the ground with a jack-in force adjusted in steps up to between 1.8-2.5 times working load , figure – 33, displaying the hydraulic Jack-in piling machines in construction site.
When the jacking pile reaches certain depth and (refuses penetration) at the desired corresponding pressure, the pile may have set. The set of the pile is completed when the jacking force is taken as 2.0 times the pile working load and is maintained for 30 seconds with residual settlement not more than 5mm. Once set the extruding length of the pile shall cut off by usage of a diamond cutter to facilitate movement of the machine.
Subsequently, from early 1990 Jack-in pile technique has applied in Malaysia, (Sew & Meng, 2009).The advantages of Jack-in piling technique are:
- Environmental Friendly Noise, pollution free – vibration-free, no mud slurry, minimal or no excavated material to be disposed. Proficient piling system, in open land or nearby building exits.
- Sound quality compared to bored piles, as piles are pre-cast and install by jacking in
- No hard driving, no uncertainty of in-situ underground concrete casting
- Considerable faster than constructing of bored piles
- Capacity of each pile installed is verified by a jack-in force up to two times design loads (DL) or higher
- Obstructions in the dump material are not a disquiet when the pile is jacked in under force of 2DL or higher
- The problems will push to side or down to the bedrock
- Lower Risk of machine toppling as compared with conventional leader type machines
- Every pile is jacked up to between 1.8 times – 2.5 times working load
- Able to succeed good verticality to the piles
- Side lacking for confident space fast and accurate
Figure33: Displaying the hydraulic Jack-in piling machines in construction site in Kuala Lumpur
Jack-in piling machines low capacity machines of 100 to 130 tons, medium capacity machines of 240 to 420 tons and the high capacity machines of 600 to 800 tons. The requirement to operate Jack-in piling technique is the ground must be level and compact, the pile location must be clearly marked and a separate crane is use for longer piles 10m or more.
- R. & Haji, Taib, S. (2012) which describe the subsurfacefeatures that come upon in engineering works on karst terrains, after applying of geotechnical survey.
- RESULTS AND DISCUSSIONThis geo – Environmental study of field models demonstrated that the Electrical Resistivity Imaging technique is a useful geophysical tool that can be used to image and characterize the surface of bedrocks and its features in the subsurface karst terrain for many reasons:
- ERT is a version of the traditional resistivity methodology, which generally means that it is still limited by the common limitation of established resistivity methods. The two advantages of ERT are an automated data collection having a limitation control of data quality in the field, and imaging inversion processing that are capable of portraying sharp geological contacts as gradational boundaries or zones.
- The variation in the geology due to irregular distribution of pinnacles and gutters in these karst terrains being analyze can represent an imperative consideration that might affect the accuracy and the reliability of this technique.
- A comparison of the depth to limestone, determined from pre-existing borings interpreted from ERT profiles, showing an average difference of 1 m, with a maximum of 3 m in the interpretation of several profiles.
- The bedrock-surface for all site sections produced to provide a reasonable representation of the bedrock surface, and has verified by data from exploratory boreholes.
- This field example demonstrates that ERT is a useful geophysical tool for characterizing the bedrock surface in covered karst terrains.
- ERT with 5.0 m electrode spacing provide sufficient resolution that pinpoints the locations offilled sinkholes, cavities and other geophysical anomalies.
- The interpretation of the resistivity data indicates that reactivated sinkholes have produced by a large cavity, and most of the soil in the sinkhole appears to have flowed into the subsurface via solution-widened system of joints.
- Many sinkholes and cavities were spotted underneath the surface in the ER Tomography sections, in the selected site under study, supporting the theory that all these cavities came from pre-existing features that have rapidly in- filled with clay. Though only a few have discovered, which gives credence to the speculation that it is newly developed feature that were as result of collapse or subsidence.
- The overlying sandstone which discover in multiple locations on the surface at site no.1, site no.2, and no.3, are mostly characterized by; high resistivity, and was interpreted as sandstone dry and friable, due to the ex-mining excavation operation, had been stripped by earth-moving equipment from the pits of the Tin-mine, which are located adjacent to the study sites. At site no.4, look like used to seal the subsidence area at this location.
- The isolated areas of high resistivity near the surface perhaps caused by the concentrations of residual cherty conglomerate, iron oxide nodules, or limestone fragments.
- The Electrical Resistivity Imaging technique is capable of constraining the subsurface geological information, which enables geologists to put into the operation projects, and decide the implement required for prospecting in these karstic regions.
- Most of the Karst features at Kuala Lumpur and Selangor that are buried beneath the alluvium appears at two scales; the smaller scale are in the depth below the ground surface, while the larger scale are in the depth on the dissolution of discontinuities below the bed rock
- Estimation of the larger-scale karst directions based on the patterns of streams and their relationship with fractures lines.
- The structural study on Selangor and Kuala Lumpur by employing Radar Satellite image, showing several sets of lineaments representing (fractures and Faults), originating from many directions, which are NW-SE, N-S, NE-SW, WNW-ESE and ENE-WSW, with very few found in he E-W direction.
- Furthermore, this study found that most of drainage patterns created along each fold outcropping within the study area have based on the direction of the fracture and fault. Due to the orogenic movement during the earlier period affecting the study area, leading to deep erosion processes in the orientations, which reflects a strong linkage between the orientation of the lineaments and the drainage patterns.
- Consequently, the research demonstrated an existing relationship between the karst in Kuala Lumpurs limestone and visible surface of drainage patterns.
- This research shows the best surface and subsurface geotechnical remediation process that can use with the integrating techniques in site characterization, modeling and monitoring the karst features which are important to hazard mitigation, economical safe, responsible development operation.
- This study clarified that the marbleized limestone bed rock in site 1 (Tasek university Malaya) and site 4 (Petaling Jaya) is deep only what observed in ER Tomography sections of both sites.
- This study clarified that the marbleized limestone bed rock in site 2 (Jalan Parlimen) and site 3 (Batu cave) is shallow and contain numerous combined caves system and cavities what observed in ER Tomography sections of both sites.
- In Batu cave site area the electrodes in the ERT survey conducted near the limestone hill behind the dark cave to follow the extended of limestone rocks from the surface to the subsurface and if there extended of the caves in the subsurface of this study area.
- This E. R. Tomography technique is limited to the depth of 28.6 m. This limitation severely limits our attempt to understand the activities of the underground combined caves systems and cavities that discovered in these sites.
- In this karstic environment, the construction of structures needs to the development of new process to removing of radon from the in-door and out-door air stream. In the buildings and housing complex, that contaminated with radon of two pico-curies per liter (2 pCi/l) or the greater levels at four pico-curies per liter (4 pCi/l) and other contaminants that affect the breathing present in air within buildings, these include hydrocarbons, CO, CO2, Ozone, olefins, nitrogen oxides andsulfur. This step includes contacting of in-door air stream with an adsorbent device for the removal of radon.
- The environmental experimental study of these karstic regions showed variability. In a yearlong test of radon will give the best picture of actual radon level than short test in day or in week. The study has shown that Short-range indoor and outdoor tests for the houses and buildings round these karst sites have higher radon levels. However, there are structures in karst areas give wrong or negative results in hot morning and when tested in the night become colder and give positive results or high radon problem. Besides, the geophysical techniques viewing that radon gas existing in the water in-fill sinkholes and cavities in most ER Tomography section of the sites under studied.
- In accordance to geo-technical table for engineering classification of karst ground conditions, the karst level in site#1, Tasik University Malaya observed in the ERT sections between profile # 1 and profile# 4 is an mature karst type KaIII due to presence deep Gutter or channel extend between pinnacles from profile#1 to profile#4. Furthermore, mature buried sinkholes observed in the sections of profiles #2 & #3.The karsts level in site #2, Jalan Parlimen, observed in the ERT sections between profile # 1 and profile #2 is a complex karst KaIV due to presence mature buried sinkholes, cavities and relief pinnacles. Also, observed in the ERT sections between profile # 3 and profile #5 deep gutter and caves.
Furthermore, the karsts level in site#3, Batu Cave observed in the ERT sections between profiles #1 and profile #6 is a complex karst type KaIV due to presence underground channel fill with sediments, buried sinkhole extend between pinnacles from.
And finally, the karst level in site#4, Petaling Jaya, observed in the ERT sections between profile #1 and profile #4 is an mature karst type KaIII due to presence many pinnacles and various type of buried sinkholes.
- The karst level found in the study area between, type mature karst type KaIII and complex karst type KaIV.
- Due to rapid development in the states the land is getting limited, previously Ex-mining, areas out of control become prized lands for developments. In make driven of conventional pile and bored piling come across doubts and difficulties due to various nature of the overburden materials and irregular Karstic features of limestone that need unmistakably site characterization to evaluate anddetermine the presence of subsurface Karst features.
- SUGGESTIONS FOR FUTURE SURVEY IN KUALA LUMPUR AND SELANGORThis geo – Environmental research study describes the integration of geological, geophysical, structural, satellite imaging and environmental techniques in evaluated, determined and monitoring the presence of subsurface Karst features and its deposits located at selected ex- mining sites over carbonate karst environments in Kuala Lumpur and Selangor, West Malaysia.
The hazards of the sinkholes and other karst features such as, cavities and dissolution channel pipe can cause problems to the construction projects in the near future. Moreover, the borings within these karsts regions is incapable of providing sufficient subsurface data for analysis. This might misrepresent the subsurface geological model, which might in turn lead to additional cost for corrective design. The results of applying the techniques have discussed and the suggestions are:
-
- Using the borings method to support the result of the (ERT) techniques survey in the positions that needs early plans to minimize the hazard of sinkhole and other karst features in any construction sites over covered karstificated carbonate bedrock before starting of any construction project.
- Future survey by applying Electrical Resistivity Tomography the three dimension (ERT) technique which must be developed using ERT survey technique and 3D software to provide a clear image for the subsurface features and structures, and also to provide clear indications of their directions and extent under the subsurface.
- The best plan in future survey is by applying the three dimension (ERT) technique using a space interval not more than 10m between two parallel lines, because of high lateral variation in the subsurface topography and lithology. Besides, provide the clear images to the subsurface.
- In the environmental experimental study of these karstic regions show that in a year-long test of radon will give the best picture of actual radon level than short test in day or in week. The variability study in karst environmental has shown that Short-range indoor and outdoor tests for the houses and buildings round these karst sites have higher radon levels. However, there are structures in karst areas give wrong or negative results in hot morning and when tested in the night become colder and give positive results or high radon problem. Besides, the geophysical techniques viewing that radon gas existing in the water in-fillsinkholes and cavities in most ER Tomography section of the sites under studied.
- the construction of structures needs to designing and developing of new processes to remove of radon from out-door and in-door air stream in the buildings and housing complex that constructed then contaminated with radon greater levels and other contaminants affect breathing present in air within buildings, these include Hydrocarbons, CO, CO2, Ozone, Olefins, Nitrogen oxides, Sulfur. This steps comprising contacting of out-door and in-door air stream with an adsorbent device or the removal of radon.
- The requirement of new construction design using light material in these karst sites to avoid any collapse in the structures in the near future. The karst level found in the study area between, type mature karst type KaIII and complex karst type KaIV.
-
- CONCLUSIONS
This geo – Environmental research study focuses on evaluated, determined and monitoring the potential geo- hazard of various classes of carbonate karst features and its deposits, extend in the surface and subsurface of selected ex-mining sites that situated over covered carbonate karst environment in Kuala Lumpur and Selangor, West Malaysia, via the integration of geological, geophysical, structural satellite imaging and , environmental techniques.
Electrical resistivity tomography (ERT) has employed in order to realize the research objectives and to image the subsurface karst features across the several sites. The resistivity maps successfully imaged many features with different shaped-anomaly. The tomography data from different profiles were interpreted using boring samples extracted by auger to improve the results of (ERT). The interpretation of the resistivity data was using to generate the geological model of the specified area. The variation in the geology due to irregular distribution of pinnacles and cutters in these karst environments under study can be an important factor, affecting the accuracy, integrity and reliability of this technique. An ERT with 5m electrode spacing provide an adequate resolution to pinpoint the locations of in-filled sinkholes, cavities and other geophysical anomalies. Many sinkholes and cavities have detected underneath the surface in the ER Tomography sections in the studied area, supporting the theory that all these cavities were of pre-existing feature that had rapidly filled with clay. Only a few theories gave credence to the thought that these sites are new, and might collapse due to subsidence. Cassiterite minerals (Tin-bearing alluvium) have discovered to accumulate in rich deposits of clastic sediments, silty sand with some clay, and covered by a layer of sand and gravelly sand. Zones of high-conductivity and low resistivity in the Wenner ERT sections represent clay-filled fractures, caves, or small cavities.
The structural study on Selangor and Kuala Lumpur shows several sets of lineaments representing (fractures and Faults), and originating from multiple directions, which are NW-SE, N-S, NE-SW, WNW-ESE and ENE-
WSW, with a few found in the E-W direction. Furthermore, this study stipulated that the fracture orientations based on the direction of drainage patterns along the creation of each structural fold out cropping the study area, reflecting a strong linkage between the orientation of the lineament and drainage pattern. The isolated areas that have characterized mostly by high resistivity and interpreted as dry and friable sand due to ex- mining excavating operation from the pits of tin mine located near those sites. Electrical Resistivity imaging technique is able to clarify the subsurface geological information in these karstic regions. In addition, this study had shown an impression of surface and subsurface remediation process that can use with the integrating techniques in site characterization. The implementation of these processes and its strategies which resulting in monitoring, operation, maintenance, and the costs that can impact the effectiveness, timing and cost of remedial solutions.
In accordance to geo-technical table for engineering classification of karst ground conditions, the karst levelin site#1, Tasik University Malaya observed in the ERT sections between profile # 1 and profile# 4 is an mature karst type KaIII due to presence deep Gutter or channel extend between pinnacles from profile#1 to profile#4. Furthermore, mature buried sinkholes observed in the sections of profiles #2 & #3. The karsts level in site #2, Jalan Parlimen, observed in the ERT sections between profile # 1 and profile #2 is a complex karst KaIV due to presence mature buried sinkholes, cavities and relief pinnacles. Also, observed in the ERT sections between profile # 3 and profile #5 deep gutter and caves. In addition, the karsts level in site#3, Batu Cave observed in the ERT sections between profiles #1 and profile #6 is a complex karst type KaIV due to presence underground channel fill with sediments, buried sinkhole extend between pinnacles from. Finally, the karst level in site#4, Petaling Jaya, observed in the ERT sections between profile #1 and profile #4 is an mature karst type KaIII due to presence many pinnacles and various type of buried sinkholes. The karst level found in the study area between, type mature karst type KaIII and complex karst type KaIV.
Through the field investigation of the drainage features pattern of the whole of the Klang basin on the old geological map and aerial photograph shows that these orientations are visible outside and inside this basin but the control exerted by the orientations of structure is very clear. The drainage pattern has closely adjusted to structure.
Due to rapid development in the states the land is getting limited, previously ex mining, areas out of control become prized lands for developments. In make driven of conventional pile and bored piling come across doubts and difficulties due to various natures of the overburden materials and irregular Karstic features of limestone that
need unmistakably site characterization to evaluate and determined the presence of subsurface Karst features.
Also, in the construction of structures needs to development process for removal of radon from out and in- door air stream and other contaminants affect breathing present in air within the buildings and housing complex in these karstic regions.
This E. R. Tomography technique is being limited to a depth of 28.6 m. This limitation severely limiting our attempt to understand the activities of the underground combined caves systems and cavities that discovered in these sites.
Note:
This geo-environmental research study presented by the author* Riyadh R. Yassin, in the eighth physics, maths and Geosciences conference, Bangkok Thailand, December 2012.
REFERENCES:
-
-
- Anderson, N. L., Derek, B. A., and Ismail, B. A.(2007).Assessment of Karst Activity at Highway Construction Sites using the Electrical Resistivity Method, Missouri, USA.
- Alexander, J.B. (1956). Geological map of Genting Sempah area, Kuala Lumpur (Old series sheet 3B/12), 1:63360.
- Alexander, J.B. (1965). Geological map of Malaya, 6th edition (Diamond Jubilee) 1963, 1 :500 000
- Beck, B. F., & Herring, J. G. (2001). Geotechnical and Environmental Applications of Karst Geology and Hydrology, Balkema Publishers, pp. 341-346.
- Benson, R.C., Kaufmann, R.D., Yuhr, L.B., & Martin, D.(1998). Assessment, prediction and remediation of karst conditions on I- 70, Frederick, Maryland, the 49th Highway Geology Symposium, Prescott, Arizona, Sept. 1014, 1998. (Arizona Department of Transportation, Material Group, Arizona Geological Survey), pp 313325.
- Bögli, A. (1980). Karst Hydrology and Physical Speleology, Springer -Verlag, Berlin.
- Chan, S. F. (1986).Foundation problems in limestone areas of peninsular Malaysia. Geotech, Engineering Div., IEM, Sept.1986, pp.98.
- Chang, K.S. & Wong, S.L. (2009). The limestone hills & caves of the Kinta Valley, Malaysian Nature Society, Kuala Lumpur, Malaysia, 151p.
- Chow, W.S., Jamaludin, O., & Loganathan, P. (1996). Geotechnical problems in limestone terrain with emphasis on cavities and sinkholes, Seminar in Environmental Geology, UKM, Bangi, pp.102117.
- Cobbing, E.J., Pitfeld, P.E., Derbyshire, D.P. & Mallick, D.I. (1992).The granites of Southeast Asian tin belt, Overseas Memoir 10, British Geological Survey of London, pp. 78-86.
- Cooper, A. H., Farrnt, A. R., & Price, S. J. (2011). The use of karst geomorphology for planning, hazard avoidance and development in Great Britain, Geomorphology Vol. 134, pp 118- 131.
- Dahlin T., Loke, M.H. (1998). Resolution of 2D Wenner resistivity imaging as assessed by numerical modeling, Journal of Applied Geophysics. Vol38: 237-249.
- Dahlin T. (1996). 2-D resistivity surveying for environmental and engineering applications, First Break, 14(7), pp. 275-283.
- David, P., Panagiotis, T., & Konstantinos, A. (2008). Electrical resistivity tomography mapping of beach rocks, application to the island of Thassos (N. Greece), Environ, Earth Science (2009) 59:233240 DOI 10.1007/s12665-009-0021-9.
- Douglas, I.(1968). Erosion in the Sungei Gombak catchment, Selangor, Malaysia. Jour. trop. Ceog., 26, p. 1- 16.
- Fischer, J. A., & Candace, R. (1989). Foundation Engineering Construction in Karst Terrain, Current Principles and Practices, Proc. Conf., Eveston, Illinois, ASCE, USA, 1989, pp. 2942.
- Fontaine, H., Ibrahim, B. A. (1995). Biostratigraphy of the Kinta Valley, Perak, Geol. Soc. Malaysian Bulletin, Vol. 38, pp.159- 172.
- Franklin, A.G., Patrick, D.M., Butler, D.K., Strohm, W.E. Jr., & Hynes-Griffin M.E. (1981). Foundation considerations in sting of nuclear facilities in karst terrains and other areas Susceptible to Ground Collapse, Geophysics & Geothermic Division, Geology Department, University of Athens, Panepistimiopolis, Ilissia, Athens.
- Gobbett, D. J. (1964). The Lower Palaeozoic rocks of Kuala Lumpur, Malaysia, Federation Museums Jor. 9, (New Series) p. 67- 79.
- Gobbett, D. J. (1964). The Lower Palaeozoic Rocks of Kuala Lumpur, Malaysia, Federation Museums Journal, 9, 67-79.
- Gobbett, D.J. & Hutchison, C.S. (1973). Geology of the Malay Peninsula, New York: Wiley-Interscience.
- Gobbett, D.J., (1965). The formation of limestone caves in Malaya, Malaya Nature Journal, 19 (1): pp. 4 – 12.
- Griffiths, D.H., & Barker, R.D. (1993). Two-dimensional resistivity imaging and modeling in areas of complex geology. J Appl. Geophysics 29: 211226.
- Hoek, E. (1983), 23rd Ranking Lecture: strength of jointed rock masses, Geotechnique, Vol. 33 No. 3, pp. 187 – 223.
- Hoover, R.A., & Saunders, W.R. (2000). Evolving Geophysical Standards, The First International Conference on the Application of Geophysical Methodologies & NDT to Transportation Facilities and Infrastructure Conference Proceedings, Missouri Department of Transportation.
- Hu, R.L., Yeung, M.R., Lee, C.F., Wang, S.S., & Xiang, J.X. (2001). Regional risk assessment of karst collapse in Tangshan, China, Environmental Geology 2001, 40, pp. 1377-1389.
- Hussein, I. E., Kraemer, G. & Myers, R. (2000). Geophysical characterization of a proposed street extension in Cape Girardeau, Missouri, Proceedings of the First International Conference on the Application of Geophysical Methodologies & NDT to Transportation Facilities and Infrastructure.
- Hutchinson, C.S. (2007). Geological evolution of Southeast Asia (second Edition), Geological Society of Malaysia, (Publ), 433 p.
- Ingham, F.T., & Bradford, E.P. (1960). The geology and mineral resources of the Kinta Valley, Perak, Geological Survey District Memoir 9, Federation of Malaya Geological Survey, Ipoh, p. 347.
- Ioannis, F. L., Filippos, I. L., & Melanie, B. (2002). Accurate Subsurface Characterization For Highway Applications Using Resistivity Inversion Methods, Geophysics and Geothermic Division, Geology Department, University of Athens, Panepistimiopolis, Ilissia, Athens.
- Jennings, J.N. (1985). Karst geomorphology Oxford: Basil Blackwell.
- Kaderabek, T.J., Reynolds, R. T. (1981). Miami limestone foundation design and construction,
- Geotechnical Engineering Division, GT7, Jul. 1981, pp. 859 – 872.
- Klimchouk, A. B., Ford, D. C., palmer, A. N. & Dreybrodt, W. (2000). Evolution of Karst Aquifers, the National speleological Society, Huntsville, Alabama.
- Komoo, I. (1989). Engineering geology of Kuala Lumpur, Malaysia. Proceedings of the international conference on engineering geology in tropical terrains. Bangi, Malaysia. 26-29 June 1989, pp. 262 – 273.
- Loke, M.H., & Barker, R.D. (1996). Rapid least-square inversion of apparent resistivity pseudo section by a quasi-Newton method, Geophysics Prospect 44: 13152.
- Loke, M. H., &Barker, R. D. (1994). Rapid least-squares inversion of apparent resistivity pseudo-sections Extended Abstracts of Papers 56thEAGE Meeting Vienna, Austria 6-10 June 1994, p.1002.
- Loke, M.H. (1994). The inversion of two-dimensional resistivity data, Unpubl, PhD thesis, University of Birmingham.
- Loke, M.H. (1999). Time-lapse resistivity imaging inversion. Proceedings of the fifth Meeting of the Environmental and Engineering, Geophysical Society, European Section, Em1.
- Louis I.F., Bastou, M. (2002). Accurate Subsurface Characterization For Highway Applications Using Resistivity Inversion Methods, Geophysics & Geothermic Division, Geology Department, University of Athens, Panepistimiopolis, Ilissia, Athens.
- Ludovic, S., Heinz, S., Daniel, H. (2011). Radon and CO2 as natural tracers to investigate the recharge dynamics of karstaquifers, Journal of Hydrology , Volume 406, Issues 34, 6 September 2011, Pages 148157
- Mitchell, J.M. (1985). Foundations for the Pan Pacific Hotel on pinnacled and cavernous limestone. In: Proceedings of the 8thSoutheast Asian Geotechnical Conference, Kuala Lumpur, 11- 15 March, 1985, 1. Kuala Lumpur: Organizing Committee, 1985, P4/29 – P4/44.
- Muhammad, R. F., & Yeap, E. B. (2002). Estimating Dissolution Rates in Kinta and Lenggong Valleys the Micro Erosion Meter, Geol Soc Bull. 45, p26-27.
- Muhammad, R. F. (2003). The Characteristic and Origin of the Tropical Limestone Karst of the Sungai Perak Basin, Malaysia, Unpubl, PhD, University of Malaya, 443p.
- Muhammad, R. F., Al-Kouri, O. & Yassin, R. (2010). Geophysical and geospatial database of sinkhole occurrences distribution after earthquake tsunami, Department of Geology, Faculty of Science, University of Malaya.
- Muhammad, R. F. (2003). The Characteristic and origin of the tropical limestone Karst of the Sungai Perak Basin, Malaysia, University of Malaya, PhD thesis, p. 443.
- Muhammad, R. F. &Yeap, E. B. (2002). Estimating Dissolution Rates in Kinta and Lenggong Valleys the Micro Erosion Meter, Geol Soc Bull. V45, pp. 26-27.
- Neil L. Anderson, Derek, B. Apel & Ahmed, Ismail, (2007). Assessment of Karst Activity at Highway Construction Sites Using the Electrical Resistivity Method, Missouri, USA”.
- Niel A. Yahia, Yassin, R. R., & Sameer, R. H. (1994) , Applying of compound geophysical techniques to detecting and locating the Weakness zone and the water seepage in the body of the AL- Tharthar dam , samara town, Salahuddin province / Iraq Geosurv. / Baghdad Iraq.
- Paton, J.R. 1964. The origin of the limestone hills of Malaya. Journal Tropical Geography, 18, 134 147.
- Pierson, B.J. (2009).The limestone hills of the Kinta Valley a part of Malaysias geological heritage worth preserving. First Break, vol. 27, pp. 97-100.
- Pollalis, S., (2002). The Petronas Twin Towers, Kuala Lumpur, Malaysia, International Cooperation and Information Transfer in the Realization of the Worlds Tallest Buildings, Harvard School Design, Publishing Division, Harvard Design School, 1 -33.
- Price, N.J. (1966).Faults and Joints developments in brittle and semi-brittle rocks. 164, Pergamum Press Ltd.
- Psomiadis, D., Tsourlos, P., & Albanakis, K. (2008). Electricalresistivity tomography mapping of beach rocks, application to the island of Thassos (N. Greece) Environ, Earth Science (2009) 59:233240 DOI 10.1007/s12665-009-0021-9.
- Reitz, H.M. & Eskridge, D.S.(1977). Construction methods which recognize the mechanics of sinkhole development in bowling green, Western Kentucky University, Department of Geology and Geography, pp. 432-438.
- Rose, A.W., Hawkes, H.E., & Webb, J.S. (1979). Geochemistry in mineral exploration: Academic Press, 657 p.
- Riyadh, R. Y., Samsudin H. T., Ros, F. M. (2012). Integrated techniques to evaluate, determined and monitoring the presence of subsurface karst features and its deposits in selected carbonate karst environment in Kuala Lumpur and Selangor, Peninsular Malaysia , The South East Asia 8th physics, maths and Geosciences Congress, Bangkok Thailand, December 2012.
- Seng, C. K.,, &Wong, S.L. (2009). The limestone hills and caves of the Kinta Valley, Malaysian Nature Society, Kuala Lumpur, Malaysia, p. 151.
- Sowers, G.F.(1996). Building on sinkholes: Design and construction of foundations in karst terrain American Society of Civil Engineers, New York, p. 115.
- Sowers, G. F.(1976). Failures in limestones rocks in humid subtropics. Proceedings of American Society for Civil Engineers, 101 (GT8), pp.771- 787.
- Stauffer, P.H. (1968), The Kuala Lumpur fault zone: a proposed major strike-slip fault across Malaya, Newsletter Geological Society Malaysia, 15, 2-4.
- Stauffer, P.H. (1968), The Kuala Lumpur fault zone: a proposed major strike-slip fault across Malaya. Geological Soc, Malaysia Newsletter, 15, p. 2-4.
- Strahler, A.N. (1957), Quantitative analysis of watershed geomorphology. Amer. Geophysics, Unioll Trail S., 38, p. 913- 920.
- Tan B.K., Ch’ng, S.C. (1987). Urban geology of Ipoh and Kuala Lumpur, Forum on urban geology and geotechnical engineering in construction, 1st July, 1993, P. J. Malaysia, organized by IEM & GSM, pp. 1-25.
- Tan, B. K. (1983). Geology and urban development of Kuala Lumpur, Malaysia, geological society of Hong Kong, Bullten No.3, oct.1987, pp.127-140.
- Tan, B.K. & Komoo, I. (1990). Urban geology, case study of Kuala Lumpur, Malaysia, Engineering Geology, 28, 71-194.
- Tan, B.K. (1987). Some geotechnical aspects of urban development over limestone terrain in Malaysia. Bullten of the international association of engineering geologist, pp. 35, 57, 63.
- Tan, B.K.(1987). Geology and urban development of Kuala Lumpur, Malaysia. In: Proceedings of LANDPLAN III Symposium, Hong Kong, December 1986, Association of Geoscientists for International Development, Hong Kong, 127- 140.
- Tan, B.K. (1996). Project KLCC: Geology, soils and foundations. Warta Geology, 22 (2), 73-74.
- Telford, W.M., Geldart, L.P., & Sheriff, R.E. (1990). Applied Geophysics, (2nd edition). Cambridge University Press, New York.
- W., Zhou, & B.F., Beck, J.B. (2000). Stephenson Reliability of dipole-dipole electrical resistivity tomography for defining depth to bedrock in covered karst terrains.
- Waltham, A. C., & Fookes, P. G. (2005). Engineering classification of karst ground conditions, Quarterly Journal of Engineering Geology and Hydrogeology. vol. 36, pp. 101-118. William, E. D., Jonathan, E. N., Carpenter, P. J., Kaufmann, R. D.,& Bradley, J.C.( 2002). Geophysical Surveys of a Known Karst Feature, Oak Ridge Y-12 Plant, Oak Ridge, Tennessee.
- Waltham, A. C., & Fookes, P.G. (2003). Engineering classification of karst ground conditions. Quarterly Journal of Engineering Geology and Hydrogeology, 38, 101-118.
- Waltham, A.C., Bell, F.G., & Culshaw, M.G.( 2005), Sinkholes and subsidence: karst and cavernous rocks in engineering and construction. Praxis Publishing, 381.
- White, W.B. (1988). Geomorphology and hydrology of karst terrains. Oxford: Oxford University Press.
- Wilford, G.E. (1964). The geology of Sarawak and Sabah Caves: 12 – 16, Bull.6, Geol. Survey Dept. Borneo Region, Malaysia.
- Wu, Yongle, & Mei, Y. W. (1982). Multi-phase intrusion and multi-phase mineralization in Xihuashan tungsten ore field, in Hepworth, J.V., and Yu Hong Zhang, eds., Tungsten geology, Jiangxi, China: Bandung, Indonesia, ESCAP/RMRDC, p. 437- 449.
- Yahia, N.A., Yassin, R.R., & Abdel Qadir, S. O. (1992). The results of Applying geophysical techniques in Prospecting and Investigation of Bauxite Ore deposits in subsurface karst terrains / Iraqi western desert, Rep.no.2262,geo-surv, Baghdad Iraq.
- Yahia, N.A., Yassin, R.R., Hijab, S. R. (1994). The application of complex geophysical techniques to detecting and locating the Weakness zone and the water seepage in the body of the AL- Tharthar Dam , samara town, Salahuddin province / Iraq . Geosurv. / Baghdad Iraq. NI-38-10, SEGSM, 2265.
- Yahia, NA., Yassin, R. R., Abdel Al-Qadir S.O. (1992). Results of applying integrated geophysical techniques in Prospecting and Investigation of Bauxite ore deposits in subsurface karst terrains / Iraqi western desert, Geosurv. / Baghdad Iraq.
- Yassin, R. R. (2002). Evaluating the presence of karst Bauxitic clays deposits in parts of Western Desert of Iraq by the application of VLF electromagnetic and Electrical resistivity techniques, Geology Department, college of science university of Baghdad. / Baghdad Iraq, 223p.
- Yassin, R. R., Hj- Taib, S., & Muhammad, R. F. (2013). Reliability of Wenner ER Tomography and satellite image techniques in recognizing and assessing the geohazard development of subsurface carbonate karst features in selected construction sites in (Kinta valley) Perak, Peninsular Malaysia. The fifth regional conference on Geological Engineering, Kuala Lumpur, Malaysia.ISBN:978-967-0380-23-0.
- Yeap, E.B. (1985). Irregular topography of the subsurface carbonate bedrock in the Kuala Lumpur area. In: Proceedings of the eighth Southeast Asian Geotechnical Conference, Kuala Lumpur, 11-15 March, 1985, Kuala Lumpur, Organizing Committee, 1985, P4/1-P4/12.
- Yeap, E.B. (1987). Engineers geological site investigation of former mining areas for urban development in Peninsular Malaysia. Geological Society of Hong Kong Bulletin, 3, 319 334.
-