
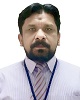
- Open Access
- Authors : Abid Siddique , Dr. Rehna V. J.
- Paper ID : IJERTV11IS010173
- Volume & Issue : Volume 11, Issue 01 (January 2022)
- Published (First Online): 05-02-2022
- ISSN (Online) : 2278-0181
- Publisher Name : IJERT
- License:
This work is licensed under a Creative Commons Attribution 4.0 International License
Fabrication and Testing of an Optimal H-Plane Sectorial Horn Antenna to Operate at 10.52 GHz
Abid Siddique
Engineering Department
University of Technology and Applied Sciences – Ibri Ibri, Sultanante of Oman
Dr. Rehna V. J.
Engineering Department
University of Technology and Applied Sciences – Ibri Ibri, Sultanante of Oman
AbstractThis paper deals with the design, fabrication and testing of an optimal microwave antenna, namely the H-plane sectorial horn antenna. The specific objectives of the research include, design study of H-plan sectorial horn antenna, arriving at the antenna design parameters for specified laboratory conditions, obtaining the reception characteristics of reference Pyramidal horn antenna, fabricating and testing the designed H- plane Sectorial horn Antenna and obtaining the reception characteristics of fabricated antenna. The antenna is fabricated using a CNC milling machine and tested using the LVDAM_ANT software. The antenna is intended to meet the following constraints in this proposed design; operating frequency of 10.52 GHz, maintaining a high power gain of around 71 dB over the entire operating frequency and directive range, and a narrow beam width in the direction of transmitting or receiving high distances.
KeywordsSectorial horn, antenna design, power gain, radiation pattern.
-
INTRODUCTION
Wireless communications are increasingly important in our lives, and are often considered as the fastest-growing networking industry sector. The antenna is a major component in any wireless technology systems. It can be said that the antenna is the eyes and ears of a wireless communication device. An antenna is an instrument designed to transmit and/or receive signals with minimum distortion to the message signal at the receiving end. A Horn antenna is often used as an antenna at microwave frequencies. The horn antenna is essentially a hollow pipe with a wide opening taking on various forms of cross sections. There are several common types of electromagnetic horn antennas including the E-plane, H-plane, conical and pyramid horns [1]. This paper deals with the optimized design, fabrication and testing of a H- plane sectorial horn antenna for microwave applications, due to its compact size, simple structure, preferred radiation, and high gain.
Specific parameters such as anticipated operating frequency, bandwidth and gain and radiation pattern are carefully considered in the design and manufacture of H-plane sectoral antenna. H – plane sectorial horn is therefore intended to meet the following constraints in this proposed design; operating frequency 10.52 GHz, maintaining a high power gain of around 71 dB over the entire operating frequency and directive range, and a narrow beam width in the direction of transmitting or receiving high distances. The realistic design is aimed at using balanced screws to support tunable bandwidth. H-sectorial antenna is designed to work on X-band (8- 12
GHz) using reasonable size and optimum gain but again a very wide radiation pattern with a long side loop. The antenna is fabricated using the CNC milling machine, with an Aluminum alloy of 3 mm thick and tested using the LVDAM_ANT Software. The paper is organized as follows. A comprehensive survey on designing Horn antennas in presented in Section 2. Section 3 deals with the proposed antenna design, followed by fabrication process in Section 4. An overview of LVDAM_ANT software used for antenna testing is given in Section 5. The results are discussed in Section 6 and the study is concluded in Section 7.
-
LITERATURE REVIEW
Horns are among the simplest and most widely used microwave antennas and they find applications in the areas of wireless communications, electromagnetic sensing RF heating and biomedicine [2]. The first horn antenna was constructed by an Indian radio researcher and one of the father of radio science Jagadish Chandra Bose, in the year 1897 [3]. Many researches are going on in the design of antenna system as it is the main source for any communication system, especially on horn antenna because of its extensive application [4].
Horn antennas can be designed in variety of shapes and sizes to fulfill many practical applications and can be used as feed element for other antennas such as reflectors [5]. There are several factors that influence the on-axis gain of horns. When calculating the gain, it is usually assumed that only the dominant mode TE10 is present and propagating within the antenna; however, this does not occur, because when the dimensions of the antenna become larger, other higher-modes will appear in the antenna aperture, changing its gain [6].
Another main consideration in designing horn antennas is impedance matching which is very desirable particularly with radio frequency transmission lines. Standing waves lead to increased losses and frequently cause the transmitted to malfunction [7]. When one considers a waveguide without a horn in operation, the sudden interface of the conductive walls or free air as the case may be for interception or transmission of microwaves cause an abrupt change in impedance at the interface. This often results in reflections, losses and standing waves. Also when the flare angle becomes too large as it tends to 90 degrees, the operation tends to assume that of a hornless antenna thereby resulting in losses, reflections and standing waves [8].
The integrity of signal received or transmitted depends largely on the design considerations of the horn antenna. Therefore, it is essential that, while deciding on the intended frequency of
operation, one need to define critical parameters upon which such design would be predicated such as the cut off frequency, hence the bandwidth of the horn antenna, the physical length dimensions, the dipole distance and depth and the hood size. For any decent design, good judgements on these parameters are extremely essential to the realization of any sound horn antenna with a decent beam pattern [8].
-
PROPOSED ANTENNA DESIGN
The horn is a waveguide which has been flared to a larger opening. The type, direction and the flare angle can have a big effect on the overall performance of the element as a radiator. Variety of shapes and sizes are connected to many practical applications such from communication systems, electromagnetic sensing, radio frequency heating, testing and evaluation, biomedicine and a reference source for other antenna testing. Sectoral and pyramidal horn antennas are associated with rectangular waveguides where the fundamental mode is the TE10, while circular waveguides feed conical horns and the fundamental mode is the TE11 [9].
An electromagnetic horn can take many different forms, four of them are shown in Fig. 1. Probably the most commonly occurring horn is the rectangular pyramidal one. The pyramidal horn shown in Fig. 1(c) is used as a primary gain standard since its gain may be calculated to within 0.1 dB by knowing its dimensions. By varying the rectangular-aperture dimensions is possible to control independently the beam widths in the two principal planes, E-plane and H-plane. Circular geometries are also in widespread use, and shapes such as elliptical and super-elliptical provide variation required for beam-shaping. A special case is the sectoral horn, which is flared in only one plane and thus radiating a broad beam in the plane orthogonal to the flare [10].
Fig. 2. Geometric parameters of H-plane sectoral horn.
The radiation pattern of a sectorial horn antenna will depend on A (the dimension of the horn at the opening) and R1 (the length of thehorn, which also affects the flare angles of the horn), along with b and a (the dimensions of the waveguide). These parameters are optimized in order to cater to the performance of the horn antenna, and are illustrated in Fig. 2.
A. Calculation of Design Parameters
The following design equations for the H-plane sectorial horn antenna geometry will be used in further analysis.
(1)
(2)
(3)
Substituting (2) in (4);
For Sectorial horn,
(4)
(5)
(6)
Fig. 1. Different configurations of electromagnetic horn antennas
The H-plane sectoral horn is one whose opening is flared in the direction of the H-field while keeping the other constant, and is shown in Fig. 1 (b). The horn is fed from a rectangular waveguide of interior dimensions a and b, with a, the broad wall dimension. The aperture is of width A in the H-plane. The H-plane cross section in Fig. 2 reveals the geometrical parameters.
For f = 10.52 GHz, and taking the tube wall waveguide dimensions of the reference pyramidal horn antenna at the laboratory conditions, width of the waveguide, a = 2.3 cm and height of the waveguide, b = 1 cm, R1 = 8.5 cm. Using the above design equations, the design parameters, A, , , are arrived at.
At operating frequency, f = 10.52 GHz; the operating wavelength,
The design parameters are shown in the geometry of H- plane sectorial horn antenna in Fig. 3.
Fig. 3. Design parameters and geometry of H-plane sectorial horn antenna.
-
ANTENNA FABRICATION
The AutoCAD program was used to design and fabricate the antennas as shown in Fig. 4a and b. The antenna structure is made of Aluminium alloy sheet of width 3 mm. Fig. 5, Fig. 6 and Fig. 7 shows the Clamp, Top cover and Base plate respectively. Designed parts are fabricated using a CNC milling machine and the base plate is shown in Figure 8. The material used is Aluminium sheet of 3 mm thickness. An ultrasound generated integrated horn antenna is shown in Fig. 9.
Fig. 4a. AutoCAD for antenna design
Fig. 4b. Antenna fabrication hardware
Fig. 5. Clamp
Fig. 6. Top Cover
Fig. 6. Base Plate
Fig. 8. A piece of the base plate of the horn antenna
Fig. 9. Ultrasound generated integrated horn antenna
-
OVERVIEW OF LVDAM_ANT TESTING SOFTWARE
The LVDAM-ANT is a Windows-based software supplied with the Festo Didactic Antenna Training and Measuring System (ATMS). This system is a powerful tool for the hands- on study of antennas in the 1-GHz and 10-GHz bands. In the Antenna Training and Measuring System (ATMS), LVDAM_ANT has two main functions: monitor the acquisition of antenna radiation patterns, and view the acquired radiation patterns for observation and measurement. LVDAM-ANT monitors the rotation of the receiving antenna when obtaining any antenna radiation pattern, and records the transmitted signal level at fixed angular intervals. Digital displays show the signal level and angular location of antenna currently obtained. If the patterns of antenna radiation have been collected, they can be transferred to an antenna file (filename.ant). Characteristics of the radiation pattern can be seen in either 2-D, 3-D, EH, or Cartesian view. In the two-
dimensional and Cartesian views, the radiation pattern characteristics of many antennas can be displayed simultaneously. In the Cartesian view, the radiation patterns of each antenna are shown as color coded polar plots of the signal level obtained versus the angular location of the antenna. The antenna radiation patterns are shown one at a time in the E-H and in three-dimensional views. The currently selected pattern of antenna radiation can be shown simultaneously in two orthogonal planes (EH view) or combined to form a three- dimensional representation of the pattern of antenna radiation (3-D view).
Data relating to the radiation patterns include the radiation patterns' maximum signal level (MSL), maximum signal location (MSP), and half-power beam width (HPBW). In the 2-D view, adjustable cursors allow for calculation of the relative signal level on each radiation pattern at any angular location. Testing antennas with LVDAM-ANT essentially involves obtaining radiation patterns of the antennas and showing them for observation and analysis in either a two- dimensional, Cartesian, three-dimensional, or EH view.
-
RESULTS AND DISCUSSION
Reception characteristics of designed H-plane sectorial horn antenna using LVDAM_ANT Software are shown in Figure 10. The designed H-plane sectorial Horn antenna is tested to obtain its H plane and E plane radiation patterns. The 2D, 3D, EH and Cartesian views of the test antenna in the H plane is shown in Figure 10 (a). The operating frequency is
10.525 GHz. Polarization is linear. The signal level is -28.8 dB. The 2D radiation pattern of the test antenna in the H Plane is shown in Figure 10 (b). The Half Power Beam Width (HPBW) is 62.84o.
Fig. 10 (a). 2D, 3D, EH and Cartesian views of Sectorial Horn H-plane Pattern
Fig. 10(b). 2D H-plane radiation pattern of Sectorial Horn Antenna
The 2D, 3D, EH and Cartesian views of the test antenna in the E plane is shown in Fig. 10 (c). The 2D radiation pattern of the test antenna in the E-Plane is shown in Fig. 10 (d). The Half Power Beam Width (HPBW) is 76.32o.
Fig. 10 (c). 2D, 3D, EH and Cartesian View of Sectorial Horn E-plane
Pattern
Fig. 10 (d). 2D E-plane radiation pattern of Sectorial Horn Antenna
-
CONCLUSION AND FUTURE SCOPE Waveguide flaring is the feature of horn antennas. This
increases the transmitting signal's directionality and boosts the corresponding impedance. This form is directional and has high gain. So, it has been used to communicate long distances. Due to its compact size, simple structure, preferred radiation, and high gain, H-plane sectorial antenna is selected and optimized for microwave applications. The H-plane sectorial horn is therefore intended to meet the following constraints in this proposed design; operating frequency 10.52 GHz, maintaining a high power gain of around 71 dB over the entire operating frequency and directive range, and a narrow beam width in the direction of transmitting or receiving high distances. Horn antennas have very little loss, so the directivity is roughly equal to its gain.
The realistic design is aimed at using balanced screws to support tunable bandwidth. Which is achieved successfully while maintaining a compact size but with a very large radiation beam width in the direction of the H- field, which
causes low orientation of the antenna and less radiating for high distance radiation. It is found that in comparison with a very low power gain, the radiation pattern is almost appropriate, which makes this antenna not ideal for middle and high range of microwave applications.
The study does not include the configuration of an H-plane horn antenna with a very high gain and a narrow radiation pattern while retaining its compact size. This can be a potential area to be carry out in future. A sectorial H- plane may also be planned in future using different sizes, then each approach may be evaluated based on one.
ACKNOWLEDGMENTS
This research work was sponsored by and carried out at the University of Technology and Applied Sciences, Ibri, Oman, from September 2020 to August 2021. Authors thank the college administration for providing all necessary tools, instruments and other facilities which has enabled to finish the work successfully. Special gratitude is expressed to the College Dean and Head of the Engineering Department, for their support.
REFERENCES
-
P. Gowtham Kumar, P. Chandrasekhar and S.B. Sai Rama Raju, Study on Slotted Waveguide Pyramidal Horn Antenna with Enhanced Directivity. IPASJ International Journal of Computer Science (IIJCS), 2015, Vol 3, Issue 3.
-
Konstantinos B. Baltzis Polynomial Based Evaluation of the Impact of Aperture Phase Taper on the Gain of Rectanguar Horns, Journal of Electromagnetic Analysis & Applications, 2010, Vol. 2, pp. 424 430.
-
Biswa Ranjan Barik and A. Kalirasu, Design of a UHF Pyramidal Horn Antenna Using CST, International Journal of Pure and Applied Mathematics, Vol 114, No. 7, 2017, pp. 447- 457.
-
U. C. Gope, S. Rana and N.K. Das Design, Fabrication and Simulation of Pyramidal Horn Antenna at 950MHz frequency, International Journal of Engineering and Applied Sciences (IJEAS), Vol 6, Issue-1, January 2019, pp. 4 8.
-
R. Kiran Chand, Dr. M. V. Raghavendra and K. Sathyavathi, Radiation Analysis and Design of Pyramidal Horn Antenna, International Journal of Engineering Research & Technology (IJERT), 2013, Vol. 2, Issue 10.
-
K. Liu, C. A. Balanis, C. R. Birtcher and G. C. Barber, Analysis of Pyramidal Horn Antennas Using Moment Method, IEEE Trans Antennas Propagat., vol. AP-41, October 1993, pp. 1379-1389.
-
Samuel Y. Liao, Microwave Devices and circuits, 3rd Edition, Prentice Hall, New Jersey, 1990.
-
Daniyan O.L, Opara F.E, Okere B.I, Aliyu N, Ezechi N, Wali J, Adejoh J, Eze K, Chapi J, Justus C and Adeshina K. O, Horn Antenna Design: The Concepts And Considerations, International Journal of Emerging Technology and Advanced Engineering, Vol 4, Issue 5, May 2014, pp. 706 708.
-
Guilherme Coelho Gr´acio Silva, H-Plane Sectoral Horn Antennas in Substrate-Integrated Waveguide Technology, November 2017.
-
Meena, Mahendra & Prakash, Ved. (2018). Simulation Results of Rectangular Horn Antenna, International Journal of Engineering and Technology. Vol 3, pp. 171-179