
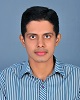
- Open Access
- Authors : Jithin Kumar I J , Athira Rajan
- Paper ID : IJERTV9IS040080
- Volume & Issue : Volume 09, Issue 04 (April 2020)
- Published (First Online): 12-04-2020
- ISSN (Online) : 2278-0181
- Publisher Name : IJERT
- License:
This work is licensed under a Creative Commons Attribution 4.0 International License
Design and Implementation of Z Source Resonant Converter for EV Wire Less Charging Application
Athira Rajan |
Jithin Kumar I J |
PG Student |
Assistant Professor |
Electrical and Electronics Engineering |
Electrical and Electronics Engineering |
NSS College of Engineering |
GEC Palakkad |
Kerala, India |
Kerala, India |
Abstract Wireless Power Transfer (WPT) technology is an emerging research area due to its safety and convenience. A Conventional WPT system has Front End PFC and DC-DC Boost Converter which makes the system bulkier. The Z Source Inverter (ZSI) was introduced into WPT systems to improve the system performance. The ZSI regulates the input voltage in WPT systems without Front End Converters and makes the inverter bridge immune to Shoot Through (ST) states. The results are through simulations and Finally, the designed system is implemented experimentally
-
INTRODUCTION
WPT technology deliver power through an electromagnetic field without any physical connection between the transmitter and receiver [1]-[3]. Recent advancements in this field have led to more stringent design requirements being proposed and studied by researchers, such as efficiency improvement [4]- [8], coupling variation [9], [10], foreign object detection [11], [12], and output regulation [13]-[15]. Electronic technology plays a crucial role in these research studies and spurs WPT technology development. The voltage source inverter (VSI) is an essential part of a WPT system that generates high- frequency ac power for transmission across a wireless media. Unfortunately, the output voltage of conventional VSI is always is equal to or lower than the input voltage, which limits this inverters application in small-voltage or wide input situations. To address this barrier, front-end converters, such as boost converter or buck-boost converter are inserted between a dc source and a VSI to boost the dc-rail voltage [16], [17]. However, this require more space and increase the cost of the system. To add one additional IGBT/MOSFET one extra heat sink and associated drive circuitry is to be accommodated. Considering the incremental cost and design complications, the ZSI presents a better alternative to front- end converters in WPT system.
When compared with a conventional VSI, the ZSI has an input diode DS and a Z-source network added between the dc voltage source and the VSI [18]. The Z-source network consists of two identical inductors (L1 and L2) and capacitors (C1 and C2) to boost the output voltage by shorting one or two legs of the rear-end inverter bridge. This is also referred to as the shoot-through (ST) state.
Guidelines to design Z-source network based on steady state parameters were presented in [19]. Since the network is connected to a three-phase VSI, the output current of the
network is regarded as a constant current. Meanwhile, the current in the network of WPT system is approximately sinusoidal over part of one switching period due to a sinusoidal resonant current. The mathematical analysis in [19] is modified when applied to a WPT system. In [20], the benefits of a ZSI in resonant converters are analyzed. These benefits include improved robustness and reliability, buck/boost function, and high efficiency over wide input and load ranges.
The ZSN in the proposed ZSRI provides the unique feature of inherent power factor correction (PFC) without adding extra switching devices. It is possible because it adds the unique features of immunity to the H-bridge inverter during shoot- through states. This characteristic makes the input current as a sinusoidal waveform and in phase with the ac input voltage. This variable also provides a boost factor to the system. However, to regulate the output voltage, the proposed ZSN- based inverter uses the active state duty cycle, which is a common control variable used in series resonant inverters. Both control variables are used in the series resonant H- bridge inverter and the ZSRI does not require additional control circuitry to provide power factor correction. In other words, because of the ZSN, the ZSRI can perform power factor correction as well as dc/ac conversion in single stage.
-
OPERATION OF Z SOURCE RESONANT
CONVERTER
The ZSRC has more states in one switching cycle compared with other DC- AC systems. It is important to clarify all these states to understand the ZSRC. The boost ratio of ZSN is still related to the total shoot-through state duty cycle among these states. The operation principle of the ZSRC is described based here with an example of the phase-shift control method. Assuming that the ZSN is symmetrical (C1 = C2 = C, and L1 = L2 = L) therefore, VC1 = VC2 = VC, and VC1 = VC2= VC. Also, the resonant frequency of L and C in ZSN is at least ten times smaller than the switching frequency. Hence, the ZSN inductor current and the ZSN capacitor voltage are considered to be constant in one switching cycle. Fig. 2 shows the conducting devices in different statesactive state, shoot through state, and zero state.
Fig 1. Circuit of WPT System with a ZSI
-
Active State: During the two active states time interval [see Fig. 6(c) and (g)], the diagonal switches are on, and the input side diode D1 is conducting. The resonant network draws current from both the ZSN inductor and capacitor. The difference between load current and ZSN inductor current is provided by a series connection of the two ZSN capacitor and dc source. The current going through the switches are only load current The ZSN inductor voltage for this time interval is given as
-
Shoot-Through State: Three of the switches are ON. The two horizontal switches are carrying the load current and the switches in one-phase leg are carrying the ZSN inductor current. Hence, there is one switch carrying the sum of the two currents. Since the flow of ZSN inductor current is always in one direction and the load current would be bipolar, these two currents either subtract from each other or add together, contributing to the sum with their absolute value. Fortunately, phase-shift control only allows different polarity currents going through the same switch in shoot-through state. Here, the ZSN capacitors will charge ZSN inductors (this is how the ZSRC can boost the voltage). The ZSN inductor voltage for this time interval is given as
-
Zero State: During the zero states time interval, two horizontal switches are ON. The ZSN is isolated from the load. The load current is freewheeling and the ZSN inductors charge the ZSN capacitors. The ZSN inductors voltage for this time interval is given
as Fig 2. ZSRC circuit diagram in different states
These three states are all the possible states in ZSRC. Different allocation of these three states along one switching period would generate different load regulation characteristic.
Fig 3. Time domain waveforms for phase shift control in ZSRC
-
-
SIMULATION OF WPT SYSTEM WITH ZSI
Simulation of Z Source Resonant Converter for EV Wireless Charging is done using MATLAB/Simulink 2016
-
Simulation Parameters
Simulation parameters are shown in table 1.
TABLE 1
The input voltage of the ZSRC is Vs=33V and the output voltage and current is Vo=88v and Io=2.28A with the resonant frequency of 20kHZ. Assuming that the ZSN is symmetrical (C1 = C2 = C, and L1 = L2 = L) therefore, VC1 = VC2 = VC, and VL1 = VL2 = VL. Also, the esonant frequency
of L and C in ZSN is at least ten times smaller than the switching frequency. Hence, the ZSN inductor current and the ZSN capacitor voltage are constant in one switching cycle.
The significant part of the design is choosing the inductor and capacitor values and operating frequency. On the contrary, use of the low frequency leads to increases both on the size and also the cost of inductors and capacitors. Thus, there is a trade-off between the size and efficiency in determining the operating frequency of the converter. The frequency is selected as 20kHz.
-
Simulation Result
The complete model of the developed WPT is shown in Fig.4
Fig 4. Simulation Diagram of ZSRC for WPT
Simulation has been performed and the results are presented for each converter stage. The circuit consists of a high frequency (HF) Z Source Resonant Converter. High frequency switching is implemented using MOSFET switches. This is the high frequency link. A HF transformer provides voltage transformation and isolation between the DC source and the load. At the output side, a full bridge rectifier is connected to load. For analytical study, a resistive load is selected. The closed loop is controlled for constant output.
Fig 5. Waveform of Gating signal for MOSFETS
Fig 6. Waveform of output voltage and output current of the ZSRC
Fig 7. Voltage across the H bridge at the primary side
-
-
HARDWARE IMPLEMENTATION
The main circuit diagram consists of four sections, converter section, control section, driver section and power circuit. The converter section consists of four IRFP460N MOSFETs. Even if there is any fluctuations in the input the output will be maintained constant by the program stored in the microcontroller. Microcontroller sends the corresponding signals which will be too feable to drive the MOSFET switches, so a driver circuit consisting of TLP250 Driver are used which boosts the amplitude of the signals enough to drive the switches.
Fig 8 Complete Hardware Setup
Fig 9 Wireless Power Transfer Setup
Fig 10 Output Obtained
-
CONCLUSION
A new circuit topology for high power WPT applications using a full bridge Z-source resonant inverter has been developed and simulated. The control methods in the system with the insertion of shoot-through modes of the Z-source inverter have been investigated. In simulation, ideal MOSFETs and diodes were used. The output voltage of 27 V and output current of 0.9 A, i.e output of 23.5 W is obtained for the coupling coefficient k of 0.2. In the experiment, an output current of 0.9 A, and output voltage of 16 V was obtained. The slight difference between the simulation and experimental results is due to the following reasons: Core losses, simulation-ideal diode and MOSFET switches were used. Nevertheless, in both the simulation and experiment, the results obtained are very close to the analytical design value. This, in turn, validates the designed parameters.
-
REFERENCES
vehicles, in Cyber Technology in Automation, Control and Intelligent Systems (CYBER), pp. 191-194, 2013.
[6] B. Kallel, O. Kanoun, T. Keutel, and C. Viehweger, Improvement of the efficiency of MISO configuration in inductive power transmission in case of coils misalignment, in Instrumentation and Measurement Technology Conference (I2MTC) Proceedings, pp. 856-861, 2014. [7] M. Fu, T. Zhang, C. Ma, and X. Zhang, Efficiency and Optimal Loads Analysis for Multiple-Receiver Wireless Power Transfer Systems, IEEE Trans. Microw. Theory Techn., Vol. 63, No. 3, pp. 801-812, Mar. 2015. [8] Z. Low, R. Chinga, R. Tseng, and J. Lin, Design and test of a high-power high-efficiency loosely coupled planar wireless power transfer system, IEEE Trans. Ind. Electron., Vol. 56, No. 5, pp. 1801-1812, May 2009. [9] O. Jonah, S. V. Georgakopoulos, D. Daerhan, and Y. Shun, Misalignment-insensitive wireless power transfer via strongly coupled magnetic resonance principles, in Antennas and Propagation Society International Symposium (APSURSI), pp. 1343-1344, 2014. [10] H. Feng, T. Cai, S. Duan, J. Zhao, X. Zhang, and C. Chen, An LCC-compensated resonant converter optimized for robust reaction to large coupling variation in dynamic wireless power transfer, IEEE Trans. Ind. Electron., Vol. 63, No. 10, pp. 6591- 6601, Oct. 2016. [11] N. Kuyvenhoven, C. Dean, J. Melton, J. Schwannecke, and A. Umenei, Development of a foreign object detection and analysis method for wireless power systems, in Product Compliance Engineering (PSES) Proceedings, pp. 1-6, 2011 [12] G. Jang, S. Jeong, H. Kwak, and C. Rim, Metal object detection circuit with non-overlapped coils for wireless EV chargers, in Southern Power Electronics Conference (SPEC), pp. 1-6, 2016. [13] L. Tan, S. Pan, C. Xu, C. Yan, H. Liu, and X. Huang, Study of constant current-constant voltage output wireless charging system based on compound topologies, J. Power Electron., Vol. 17, No. 4, pp. 1109-1116, Jul. 2017. [14] L. Sun, H. Tang, and C. Yao, Investigating the frequency forloadindependent output voltage in threecoil inductive power transfer system, Int. J. Circ. Theor. Appl, Vol. 44, No. 6, pp.
1341-1348, Aug. 2015
[15] L. Zhang, X. Yang, W. Chen, and X. Yao, An isolated soft- switching bidirectional buck-boost inverter for fuel cell applications, J. Power Electron., Vol. 10, No. 3, pp. 235-244, May 2010. [16] R. Mosobi, T. Chichi, and S. Gao, Modeling and power quality analysis of integrated renewable energy system, in National Power Systems Conference (NPSC), pp. 1-6, 2014 [17] F. Peng, Z-source inverter, IEEE Trans. Ind. Appl., Vol. 39, No. 2, pp. 504-510, Mar. 2003. [18] S. Rajakaruna and L. Jayawickrama, Steady-state analysis and designing impedance network of z-source inverters, IEEE Trans. Ind. Electron., Vol. 57, No. 7, pp. 2483-2491, Jul. 2010. [19] H. Cha, F. Peng, and D. Yoo, Z-source resonant DC-DC converter for wide input voltage and load variation, in Power Electronics Conference (IPEC), pp. 995-1000, 2010. [20] H. Zeng and F. Z. Peng, SiC based z-source resonant converter with constant frequency and load regulation for EV wireless charger, IEEE Trans. Power Electron., Vol. 32, No. 11, pp. 8813-8822, Nov. 2017. [21] T. Wang, X. Liu, H. Tang, and M. Ali, Modification of the wireless power transfer system with Z-source inverter, IET Electron. Letters, Vol. 53, No. 2, pp. 106-108, Jan. 2017.