
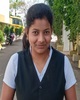
- Open Access
- Authors : Kharat Amol , Chorge Shivani , Kharat Kiran
- Paper ID : IJERTV11IS030121
- Volume & Issue : Volume 11, Issue 03 (March 2022)
- Published (First Online): 02-06-2022
- ISSN (Online) : 2278-0181
- Publisher Name : IJERT
- License:
This work is licensed under a Creative Commons Attribution 4.0 International License
An Overview on Green Synthesis of Nanoparticles for Sustainable Future
Kharat Amol*,Kharat Kiran, Chorge Shivani Government College of Pharmacy, Osmanpura, Aurangabad-431005, Maharashtra,
India
Abstract: Biological approaches seem to be the perfect options for the massive-scale manufacturing of nanoparticles. In recent years, the development of efficient green chemistry methods for the synthesis of metal nanoparticles has become an excellent element for researchers. Researchers studied an ecologically sustainable method of producing nanoparticles. Microorganisms are generally used to produce metal nanoparticles. Nanoparticles obtained from plants are more stable than nanoparticles produced by microorganisms. However, the nanoparticles are not the same form or size as those produced by other species.
This paper discusses green synthesis methodologies for nanoparticles as well as their variety of applications. It gives a collation of effective techniques of synthesis using green routes compared to other techniques, which is excellent confirmation for choosing the optimal methods for the manufacturing of nanoparticles.
Keywords: Biosynthesis, eco-friendly, sustainable, green synthesis, nanoparticles
INTRODUCTION:
Paul Anastas is the Father of Green Chemistry and is widely known for his revolutionary research on the development, manufacture, and application of non-toxic materials for a pollution-free environment. The chemical community must undergo a major shift to achieve sustainable chemistry. Green chemistry principles must be included in part of teaching and practice in chemistry1.
The nanoscale material ranges from one to a hundred nanometers used in a new area of nanoscience. They have the potential to solve problems in branches such as water treatment, catalysis, solar energy conversion, medicine. This growing demand must be fulfilled using “green” technology. The proclivity to recycle waste improves the overall environmental friendliness of the process. As a result, the method is particularly appealing due to its simplicity, as the reaction is completed in 10 minutes and no organic solvent is required at any stage of the process (1). “Green” chemistry and chemical processes are gradually integrating with modern scientific and industrial developments in the global effort to reduce hazardous waste. The implementation of these sustainable processes should adhere to the 12 fundamental principles of green chemistry3.
Following are the Green Chemistry consists of 12 principles that can be applied to almost any aspect of chemistry, along with the synthesis of molecules with the appropriate structure and properties, process catalysis, less polluting reaction conditions, and so on.
- Prevention: The prevention of waste formation.
- Less toxic substance synthesis: Synthetic methods should be designed to use and produce substances that are safe for human health and the environment.
- Before Chemicals: Chemical products should be designed to perform their intended function while remaining as non-toxic as possible.
- Atom economy: Synthetic procedures should be designed to use as few materials as possible in the end result. 5.Safer chemical design is advantage of green synthesis.
- Energy efficiency is possible in this.
- Use renewable feedstock: Where technically feasible, raw materials or feedstock should be renewable rather than depleting.
- Lessen derivatives: If possible, unnecessary derivatization (use of blocking groups, protection/deprotection/deprotection, and temporary modification of physical/chemical/chemical processes) should be reduced or avoided, as such steps require additional reagents and can generate waste.
- Catalysis : Catalysis: Selective catalytic reagents outperform stoichiometric reagents. The use of catalytic reagents allows for greater selectivity, higher yield, and the possibility of previously infeasible reactions.
- Design for degradability: Chemical products should be designed so that when they no longer serve their purpose, they degrade into innocuous degradation products and do not persist in the environment.
- Real-time pollution prevention analysis: Analytical methodologies must be improved to allow for real-time pollution prevention analysis.
2. Inherently safer for accident prevention: Substances and the form of a substance used in a chemical process should be preferred to reduce the possibility of chemical accidents such as releases, explosions, and fires.
APPROCHES OF NANOPARTICLE SYNTHESIS:
At the moment, the top-down and bottom-up approaches to nanoparticle synthesis are the two most popular. By breaking down the bulk material, the top-down approach produces nanoparticles. In contrast, the bottom-up approach creates nanoparticles through chemical reactions between atoms and/or molecules. Green methods have been shown in studies to be more effective for the generation of NPs due
Diagram 2: Approaches of green synthesis.
to lower failure rates, lower to costs, and characterization is made simple. The pH of the solution, temperature, extract concentrations, raw material concentrations, size, and, most importantly, synthesis methods must all be taken into account. Despite the many advantages of organic green synthesis, controlling NP polydispersity is a significant challenge. Optimize the reaction conditions by adjusting the pH, temperature, incubation time, irradiation, salt concentration, and redox state to tackle this issue. pH is a significant consideration in the environmentally friendly synthesis of nanoparticles. In the case of plants, pH changes affect the phytochemical charge, which affects reduction and biding during the synthesis process. Green technology is used to synthesize nanoparticles at temperatures below 100 ° C. Furthermore, particle size and porosity influence the properties of green nanoparticle synthesis. Green synthesised nanoparticles have attracted the attentions of many people due to their inherent characteristics such as speed, eco-friendliness, and cost-effectiveness. 6. The green synthesis of NPs entails three major steps that must be evaluated from the standpoint of green chemistry, namely
- selection of solvent medium,
- selection of solvent medium, and
- selection of solvent medium7.
- Physical synthesis of nanoparticles: Thermolysis, Fusion Blending, Laser Ablation, Implantation of ions, sputtering deposition, electric arc deposition and other physical processes are used to produce nanoparticles8.Microwave-assisted synthesis: Microwave- assisted reactions were carried out in a domestic microwave at atmospheric pressure9.
- Chemical synthesis is among the most widely used methods for producing large quantities of nanoparticles in a short amount of time. The disadvantages are the high toxicity of the chemical synthesis and the high cost of the chemicals used10.10
- Biological synthesis:
SYNTHESIS OF NPS FROM PLANT EXTRACT:
Diagram 3: Work flow of green synthesis of NPs using plant leaves.
Plant extract preparation: The plant’s fresh leaves to be used were collected. The leaves were thoroughly washed and dried. After powdering the dried leaves with a mortar, 20 g of powdered leaves were mixed with 200 mL deionized water and heated at 80°C for 10 minutes with a heater-stirrer. The mixture was filtered and centrifuged after the plant residue and impurities were removed before being stored or future use.
Table 1: Plant source for nanoparticle synthesis
Sr.no. | Plant extract | Part used | Precursors | Shape | Size | Reference |
1 | Sophora japonica | Pods | Na2S2O3 | Spherical | 60nm | (3) |
2 | Azadirachta Indica | Leaves | Ca(NO)3 | Star | 50nm | (4) |
3 | Plantago major | Leaves | AgNO3(5) | Spherical | 12-18nm | (6) |
4 | Citrus limon | Fruits | AgNO3 | Spherical | – | (7) |
5 | Tea | Leaves | Ca(NO3)2.4H2O | Agglomerated | 86-117nm | (8) |
6 | Papaya | Leaves | Ca(NO3)2.4H2O | Agglomerated | 89-180nm | (9) |
7 | Oscimum sanctum | Leaves | Ca(NO3)2 | Needle | – | (10) |
8 | Piper betle L. | Leaves | AgNO3 | NA | 37 | (11) |
9 | Plumeria rubra L. | Leaves | AgNO3 | NA | 32 | (12) |
10 | Piper nigrum L. | Leaves | AgNO3 | spherical | 50 | (13) |
11 | Solanum xanthocarpum L | Leaves | AgNO3 | NA | 10 | (14) |
12 | Sorghum | Leaves | AgNO3 | NA | 10 | (15) |
13 | Swietenia mahogany L. | Leaves | AgNO3 | NA | 10-35 | (16) |
14 | Trianthema decandra L. | Leaves | AgNO3 | NA | 10-50 | (17) |
15 | Tridax procumbens L. | Leaves | CuO2 | NA | NA | (18) |
16 | Zingiber officinale | Rhizome | AgNO3 | spherical | 10 | (19) |
17 | Vitis vinifera grape | Fruits | AgNO | spherical | NA | (20) |
18 | Euphorbia condylocarpa M. | Fruits | PdCl2 | NA | NA | (21) |
19 | Manilkara zapota | Leaves | AgNO3 | spherical | NA | (2) |
This plant contains a variety of bioactive compounds, such as alkaloids, fatty acids, flavonoids, terpenoids, phenolic acid derivatives, vitamins, and others, which contribute to its specific therapeutic effects (6).
b. Synthesis of NPs from microorganisms: nanoparticles are synthesized using bacteria, fungi, algae.
Table 2: Fungi source for nanoparticle synthesis
Sr.no. | Fungi | NP | Location | Shape | Size | Reference |
1 | Shewanella oneidensis | Au | Extracellular | spherical | 12 ± 5 | (22) |
2 | Aspergillus niger | Ag | NA | spherical | 20 | (23) |
3 | Aspergillus oryzae | Ag | NA | NA | 5-50 | (24) |
4 | Fusarium oxysporum | Ag | Extracellular | spherical | 1 | (25) |
5 | Klebsiella pneumoniae | Se | NA | spherical | 100 | (26) |
6 | Phaenerochaete chrysosporium | Ag | Extracellular | NA | 50 | (27) |
7 | Trichoderma asperellum | Ag | Extracellular | NA | 13-18 | (27) |
8 | Fusarium oxysporum | PbCO3 | Extracellular | spherical | 120200 | (28) |
9 | Fusarium semitectum | Ag | NA | NA | NA | (29) |
10 | Phaenerochaete chrysosporium | Ag | NA | NA | NA | (30) |
11 | Aspergilus terreus | Ag | Extracellular | spherical | 20 | (31) |
12 | Yarrowia lipolytica | Au | Extracellular | Triangles | 15 | (32) |
13 | V. luteoalbum | Au | Intracellular | NA | NA | (32) |
14 | Candida utilis | Au | Intracellular | NA | NA | (33) |
The following are the drawbacks of microbial nanoparticle synthesis:
- The requirement for isolation rooms and long-term maintainance in terms of protecting microorganism growth. Controlling the size, shape, and crystallization of nanoparticles poses great challenges.
- Manipulation of reaction parameters such as pH and temperature may interfere with microbial growth and biological induction procedures. When working with bacteria by hand, the user must take extra precautions.
- Biosafety issues should be addressed in microbiological research, large-scale nanoparticle microorganisms, and commercial applications. Nanomaterials have the potential to cause human harm or to spread dangerous bacterial diseases (34).
Table 3: Bacterial source for nanoparticle synthesis
Sr.no. Bacteria NP Location shape Size Reference 1 Escherichia coli Cds NA NA NA (35) 2 Pseudomonas stutzeri Ag Intracellular NA ~200 (36) 3 Lactobacillus strains Ag, Au NA NA 15-40 (37) 4 Corynebacterium Ag NA 5/15 NA Ag NA NA 5-15 (38) 5 Staphylococcus aureus Ag NA NA 150-180 (39) 6 Ueibacillus thermosphaericus Ag NA NA 1-100 (40) 7 Clostridium thermoaceticum CdS Intracellular and extracellular NA NA (41) 8 Rhodopseudomonas capsulata Au Extracellular spherical 10-20 (42) 9 Aspergilus turrets Ag Extracellular spherical 1-20 (31) 10 Bacillus licheniformis Ag Extracellular Spherical 23 (30) 11 Schizosaccharomyces pombe CdS Extracellular Hexagonal lattice 11.5 (43) 12 Schizosaccharomyces pombe and candida glabrata CdS Intracellular Hexagonal lattice 2 (44) 13 pseudomonas sp. Au Extracellular Spherical 10 (42) 14 Brevibacterium casei Au Intracellular Spherical 10-20 (29) 15 Enterobacter sp. Hg Intracellular Spherical 2-5 (45) CHARACTERIZATION OF NPS:
Scanning electron microscopy (SEM), atomic force microscopy (AFM), X-ray diffraction (XRD), fourier transform infrared spectroscopy (FT-IR), transmission electron microscopy (TEM), energy dispersive spectroscopy (EDS), and dynamic light scattering were used to characterise the samples (DLS).
- UV-vis spectrophotometry :
Light frequencies ranging from 200800 nm are commonly used .To characterize NPs, UV-vis absorption measurements at wavelengths ranging from 250 to 400 nm are used.
- XRD:
XRD is a valuable technique for understanding the atomic and crystal structure of nanomaterials. analysing XRD is a useful characterization tool for validating NP formation, evaluating crystal structure and planes, and determining the size of crystalline NPs. The standard JCPDS (Joint Committee on Powder Diffraction Standards) papers were used for NP confirmation.
- FT-IR:
FT-IR is a type of molecular spectroscopy that examines different chemical functional groups in the 400 to 4000 cm-1 range.
- SEM:
A fundamental analysis approach that generates a micro-image as an output using electrons rather of light. The polydispersity and purity of the resultant NPs are shown in SEM microphotographs.
- TEM:
TEM is a significant characterization technique that allows you to evaluate a material’s particle size on a nano-scale and thoroughly examine the crystal structure with high resolution. TEM analyses are carried to estimate the average size of NPs and size distribution. (46) .
APPLICATIONS OF GREEN NANOTECHNOLOGY:
- Green-synthesized nanoparticles have clinical applications. When silver nanoparticles were treated against S. aureus, their antibacterial activity was at its peak.
- Nanoparticles used as drug carriers are highly stable, can incorporate both hydrophobic and hydrophilic substances, and can be delivered via a variety of routes also including oral application and inhalation.
- Applications in healthcare:
- Dental adhesives
- Burn to heal and wound care
- Skin therapy
- Bone cements and reconstructive orthopaedics
- Medical devices and catheters made of plastic
- Targeted drug administration
- Medical imaging, biomarker detection
- Cancer treatment
- breath mask coating intellectual property rights and ultrasonic detection, Implant coating for joint replacement.
- UV-vis spectrophotometry :
- Fungicidal activity: NPs have antifungal and fungicidal activity against Candida albicans, Candida krusei, Candida species, Candida glabrata, Trichophyton mentagrophytes, and Candida tropicali.
- Antibacterial activity: NPs inhibit Gram positive and Gram negative bacteria, as well as Pseudomonas aeruginosa, Bacillus cereus, Escherichia hermanii, Bacillus subtilis, Klebsiella pneumonia, agricultural plant pathogens, and antibiotic-resistant bacteria.
- Antiviral activity: NPs has antiviral activity against human immunodeficiency virus-1, influenza virus (HIV-1), herpes simplex virus, herpes simplex virus, herpes simplex virus, herpes simplex virus, monkeypox virus, hepatitis B, HSV-1, and respiratory syncytial virus.
Table 4: Applications of NPs synthesized by green synthesis
Sr. no. | Name of plant | Application | Reference |
1 | Alianthus altissima | Seed germination study | (47) |
2 | Allium sativum | Antifungal activity | (47) |
3 | Azadirachta indica | Antibacterial activity | (47) |
s4 | Oscimum basilicum | Seed germination study | (48) |
5 | Punica granatum | Foliar spray | (49) |
FUTURE PERSPECTIVE:
Green nanotechnology is a rapidly evolving approach with applications in all aspects of life for the creation of innovative, reliable, and long-term solutions. By efficiently tackling the inherent limitations, this green technology has the potential to assist future generations in all sectors of life. On a daily basis, astonishing in the field of nanoparticle synthesis and their sophisticated applications in the huge venue of science and technology are occurring in this era of nanotechnology.
CONCLUSION:
The use of biological sources such as organisms and plants can aid in the reliable and ecologically sustainable synthesis of nanoparticles. The synthesis of nanoparticles by these natural sources is characterized by processes that take place at near-ambient temperatures and pressures, as well as near-neutral pH . The current review study incorporates current knowledge and develops a database of natural decomposition agents for various nanoparticles using various biological systems. As a result, choosing an appropriate biological element for large-scale, environmentally acceptable commercial production of nanoparticles is crucial. Simple, cost-effective, environmentally friendly, and easily scalable procedures, as well as parameters for controlling the size and shape of the materials, should be established. Recognizing the biological system’s full capacity is crucial.
ACKNOWLEDGEMENT :
I would really like to express my sincere gratitude and appreciation to my professors and supervisors for giving me with the fantastic opportunity to do this wonderful work on the topic of green synthesis of nanoparticles, which also assisted me in doing a lot of research and learning a lot of new things. I am quite grateful to them.
REFERENCE:
[1] Micheletti G, Delpivo C, Baccolini G. A green synthesis of glycoluril derivatives in aqueous solution with recycle of the waste. Green Chem Lett Rev.2013;6(2):1359.
[2] Gour A, Jain NK. Advances in green synthesis of nanoparticles. Artif Cells, Nanomedicine Biotechnol [Internet]. 2019;47(1):84451. Available from: https://doi.org/10.1080/21691401.2019.157878 [3] Depeursinge A, Racoceanu D, Iavindrasana J, Cohen G, Platon A, Poletti P-A, et al. Fusing Visual and Clinical Information for Lung Tissue Classification in HRCT Data. Artif Intell Med. 2010;11:ARTMED1118. [4] Nikam A, Pagar T, Ghotekar S, Pagar K, Pansambal S. A Review on Plant Extract Mediated Green Synthesis of Zirconia Nanoparticles and Their Miscellaneous Applications. J Chem Rev. 2019;1(3):15463. [5] Singh C, Sharma V, Naik PK, Khandelwal V, Singh H. A green biogenic approach for synthesis of gold and silver nanoparticles using zingiber officinale. Dig J Nanomater Biostructures. 2011;6(2):53542. [6] Sukweenadhi J, Irianti K, Avanti C, Kartini K, Jahan E, Yang D. South African Journal of Chemical Engineering Scale-up of green synthesis and characterization of silver nanoparticles using ethanol extract of Plantago major L . leaf and its antibacterial potential. South African J Chem Eng [Internet]. 2021;38(April):18. Available from: https://doi.org/10.1016/j.sajce.2021.06.008 [7] Iravani S. Green synthesis of metal nanoparticles using plants. Green Chem. 2011;13(10):263850. [8] Nadarolu H, Alayli Güngör A, Ince S. Synthesis of Nanoparticles by Green Synthesis Method. Int J Innov Res Rev [Internet]. 2017;1(1):69.Available from: http://www.injirr.com/article/view/4
[9] Jadoun S, Arif R, Jangid NK, Meena RK. Green synthesis of nanoparticles using plant extracts: a review. Environ Chem Lett [Internet].2021;19(1):35574. Available from: https://doi.org/10.1007/s10311-020-01074-x
[10] Naikoo GA, Mustaqeem M, Hassan IU, Awan T, Arshad F, Salim H, et al. Bioinspired and green synthesis of nanoparticles from plant extracts with antiviral and antimicrobial properties: A critical review. J Saudi Chem Soc [Internet]. 2021;25(9):101304. Available from: https://doi.org/10.1016/j.jscs.2021.101304 [11] Mallikarju K, Dillip GR, Narasimha G, Sushma NJ, Prasad Raj BD. Phytofabrication and Characterization of Silver Nanoparticles from Piper betle Broth. Res J Nanosci Nanotechnol. 2012;2(1):1723. [12] Schmidt-Ott A. New approaches to in situ characterization of ultrafine agglomerates. J Aerosol Sci. 1988;19(5). [13] Garg S. Rapid biogenic synthesis of silver nanoparticles using black pepper (Piper nigrum) corn extract Indo-Hungarian project View project Nanoparticle, wound healing, anti-cancer studies View project. 2015;(July). Available from: https://www.researchgate.net/publication/280007266 [14] Amin M, Anwar F, Ramzan M, Ashraf S. Green Synthesis of Silver Nanoparticles through Reduction with Solanum xanthocarpum L . Berry Extract: Characterization , Antimicrobial and Urease Inhibitory Activities against Helicobacter pylori. 2012;992341. [15] Njagi EC, Huang H, Stafford L, Genuino H, Galindo HM, Collins JB, et al. Biosynthesis of iron and silver nanoparticles at room temperature using aqueous sorghum bran extracts. Langmuir. 2011;27(1):26471. [16] Mondal S, Sk I, Basu S, Mandal D. mahogay. 2014;(May). [17] Raghunandan D, Bedre MD, Basavaraja S, Sawle B, Manjunath SY, Venkataraman A. Rapid biosynthesis of irregular shaped gold nanoparticles from macerated aqueous extracellular dried clove buds (Syzygium aromaticum) solution. Colloids Surfaces B Biointerfaces. 2010;79(1):23540. [18] Gopalakrishnan K, Ramesh C, Ragunathan V, Thamilselvan M. Antibacterial activity of Cu2O nanoparticles on e.coli synthesized from tridax procumbens leaf extract and surface coating with polyaniline. Dig J Nanomater Biostructures. 2012;7(2):8339. [19] Ankamwar B. 745120.Pdf. 2010;7(4):13349. [20] Venkateswarlu S, Natesh Kumar B, Prathima B, Anitha K, Jyothi NVV. A novel green synthesis of Fe3O4-Ag core shell recyclable nanoparticles using Vitis vinifera stem extract and its enhanced antibacterial performance. Phys B Condens Matter [Internet]. 2015;457:305. Available from: http://dx.doi.org/10.1016/j.physb.2014.09.007 [21] Nasrollahzadeh M, Mohammad Sajadi S, Rostami-Vartooni A, Khalaj M. Green synthesis of Pd/Fe3O4 nanoparticles using Euphorbia condylocarpaM. bieb root extract and their catalytic applications as magnetically recoverable and stable recyclable catalysts for the phosphine-free Sonogashira and Suzuki coupling reactions. J Mol Catal A Chem. 2015;396:319.
[22] Marshall MJ, Beliaev AS, Dohnalkova AC, Kennedy DW, Shi L, Wang Z, et al. c -Type Cytochrome-Dependent Formation of U ( IV ) Nanoparticles by Shewanella oneidensis. 2006;4(8). [23] Gade AK, Bonde P, Ingle AP, Marcato PD, Durán N, Rai MK. Exploitation of Aspergillus niger for synthesis of silver nanoparticles. J Biobased Mater Bioenergy. 2008;2(3):2437. [24] Binupriya AR, Sathishkumar M, Yun S Il. Myco-crystallization of silver ions to nanosized particles by live and dead cell filtrates of aspergillus oryzae var. viridis and its bactericidal activity toward staphylococcus aureus KCCM 12256. Ind Eng Chem Res. 2010;49(2):8528. [25] Durán N, Marcato PD, De Souza GIH, Alves OL, Esposito E. Antibacterial effect of silver nanoparticles produced by fungal process on textile fabrics and their effluent treatment. J Biomed Nanotechnol. 2007;3(2):2038. [26] Fesharaki PJ, Nazari P, Shakibaie M, Rezaie S, Banoee M, Abdollahi M, et al. Biosynthesis of selenium nanoparticles using Klebsiella pneumoniae and their recovery by a simple sterilization process. Brazilian J Microbiol. 2010;41(2):4616. [27] Mukherjee P, Roy M, Mandal BP, Dey GK, Mukherjee PK, Ghatak J, et al. Green synthesis of highly stabilized nanocrystalline silver particles by a non-pathogenic and agriculturally important fungus T. asperellum. Nanotechnology. 2008;19(7). [28] Ahmad A, Mukherjee P, Mandal D, Senapati S, Khan MI, Kumar R, et al. Enzyme mediated extracellular synthesis of CdS nanoparticles by the fungus, Fusarium oxysporum. J Am Chem Soc. 2002;124(41):121089. [29] Kalishwaralal K, Deepak V, Ram S, Pandian K, Kottaisamy M, Barathmanikanth S, et al. Colloids and Surfaces B: Biointerfaces Biosynthesis of silver and gold nanoparticles using Brevibacterium casei. Colloids Surfaces B Biointerfaces [Internet]. 2010;77(2):25762. Available from: http://dx.doi.org/10.1016/j.colsurfb.2010.02.007 [30] Vigneshwaran N, Kathe AA, Varadarajan P V., Nachane RP, Balasubramanya RH. Biomimetics of silver nanoparticles by white rot fungus, Phaenerochaete chrysosporium. Colloids Surfaces B Biointerfaces. 2006;53(1):559. [31] Li G, He D, Qian Y, Guan B, Gao S, Cui Y, et al. Fungus-mediated green synthesis of silver nanoparticles using aspergillus terreus. Int J Mol Sci.2012;13(1):46676.
[32] Agnihotri M, Joshi S, Ravi A, Zinjarde S, Kulkarni S. Biosynthesis of gold nanoparticles by the tropical marine yeast Yarrowia lipolytica NCIM 3589.Mater Lett [Internet]. 2009;63(15):12314. Available from: http://dx.doi.org/10.1016/j.matlet.2009.02.042
[33] Labrenz M, Druschel GK, Thomsen-ebert T, Gilbert B, Welch SA, Kemner KM, et al. Formation of Sphalerite ( ZnS ) Deposits in Natural Biofilms of Sulfate-Reducing Bacteria. 2000;290(December):17448. [34] Guan Z, Ying S, Ofoegbu PC, Clubb P, Rico C, He F, et al. Green synthesis of nanoparticles: Current developments and limitations. Environ Technol Innov [Internet]. 2022;102336. Available from: https://doi.org/10.1016/j.eti.2022.102336 [35] Parveen K, Banse V, Ledwani L. Green synthesis of nanoparticles: Their advantages and disadvantages. AIP Conf Proc. 2016;1724. [36] Klaus T, Joerger R, Olsson E. microbially fabricated. 1999;96(24):136114. [37] Sintubin L. Lactic acid bacteria as reducing and capping agent for the fast and efficient production of silver nanoparticles. [38] Zhang H, Li Q, Lu Y, Sun D, Lin X, Deng X. Biosorption and bioreduction of diamine silver complex by Corynebacterium. 2005;290(December 2004):28590. [39] Nanda A, Saravanan M. Biosynthesis of silver nanoparticles from Staphylococcus aureus and its antimicrobial activity against MRSA and MRSE.Nanomedicine Nanotechnology, Biol Med [Internet].2009;5(4):4526. Available from: http://dx.doi.org/10.1016/j.nano.2009.01.012
[40] Juibari MM, Abbasalizadeh S, Salehi G, Noruzi M. Intensi fi ed biosynthesis of silver nanoparticles using a native extremophilic Ureibacillus thermosphaericus strain. Mater Lett [Internet]. 2011;65(6):10147. Available from: http://dx.doi.org/10.1016/j.matlet.2010.12.056 [41] Cunningham DP, Lundie LL. Precipitation of Cadmium by Clostridium thernoaceticum. 1993;59(1):714. [42] pe th o r s Biosynthesis of gold nanoparticles using the bacteria. [43] Filipe CDM, Daigger GT, Grady CPL. Stoichiometry and kinetics of acetate uptake under anaerobic conditions by an enriched culture of phosphorus- accumulating organisms at different pHs. Biotechnol Bioeng. 2001;76(1):3243. [44] Rafique M, Sadaf I, Rafique MS, Tahir MB. A review on green synthesis of silver nanoparticles and their applications. Artif Cells, Nanomedicine Biotechnol [Internet]. 2017;45(7):127291. Available from: https://doi.org/10.1080/21691401.2016.1241792 [45] Sinha A, Khare SK. Mercury bioaccumulation and simultaneous nanoparticle synthesis by Enterobacter sp. cells. Bioresour Technol [Internet].2011;102(5):42814. Available from: http://dx.doi.org/10.1016/j.biortech.2010.12.040
[46] Khatami M, Sharifi I, Nobre MAL, Zafarnia N, Aflatoonian MR. Waste-grass-mediated green synthesis of silver nanoparticles and evaluation of their anticancer, antifungal and antibacterial activity. Green Chem Lett Rev. 2018;11(2):12534. [47] Salem NM, Al-banna L, Abdeen A, Society RS, Ibrahim Q. Sulfur Nanoparticles Improves Root and Shoot Growth of Tomato Sulfur Nanoparticles Improves Root and Shoot Growth of Tomato. 2016;(April). [48] Ragab GA, Saad-allah KM. Ecotoxicology and Environmental Safety Green synthesis of sulfur nanoparticles using Ocimum basilicum leaves and its prospective e ff ect on manganese-stressed Helianthus annuus ( L .) seedlings. Ecotoxicol Environ Saf [Internet]. 2020;191(November 2019):110242.Available from: https://doi.org/10.1016/j.ecoenv.2020.110242
[49] Salem NM, Albanna LS, Awwad AM. Green synthesis of sulfur nanoparticles using Punica granatum peels and the effects on the growth of tomato by foliar spray applications. Environ Nanotechnology, Monit Manag [Internet]. 2016;6:837. Available from: http://dx.doi.org/10.1016/j.enmm.2016.06.006