
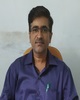
- Open Access
- Authors : Sekar Tamilperuvalathan , Haritha Kaliyaperumal Rabindranathan , Rajeswari Balakrishnan , Prabakaran Jayaraman, Surendran Ramakrishnan
- Paper ID : IJERTV12IS040148
- Volume & Issue : Volume 12, Issue 04 (April 2023)
- Published (First Online): 25-04-2023
- ISSN (Online) : 2278-0181
- Publisher Name : IJERT
- License:
This work is licensed under a Creative Commons Attribution 4.0 International License
A Critical Review of the impacts of 3D Printing Technologies on Dental Medicine
Sekar Tamilperuvalathan1*, Haritha Kaliyaperumal Rabindranathan2, Rajeswari Balakrishnan3, Prabakaran Jayaraman4, Surendran Ramakrishnan5
1* Corresponding author and Faculty of Mechanical Engineering Department, Government College of Technology, Coimbatore, India.
2- P.G Scholar of Manufacturing Engineering, Department of Mechanical Engineering, Government College of Technology, Coimbatore, India.
3 & 5 Faculty Members of Mechanical Engineering Department, Government College of Technology, Coimbatore, India. 4 Faculty of Mechanical Engineering, Alagappachettiar Government College of Engineering and Technology, Karaikudi, India.
Abstract:- In the recent past, 3D printing technologies have widely been utilized in diverse fields to address the challenges wherever involved in conventional manufacturing technologies. There are various types of 3D printing technologies like Stereolithography (SLA), fused deposition modelling (FDM), selective laser sintering (SLS), inkjet printing (IJP), and powder binder printing (PBP) that are used to fabricate the different 3D models to cater to the needs of dental applications, in particular human implant products. The creation of complicated structures using 3D printing has been made possible in a variety of dental applications like prosthodontics, orthodontics, maxillofacial surgery, oral implantology, endodontics, periodontology, and surgical devices. 3D printers have a significant contribution to make in producing components with the least price, the shortest lead time, and the least material consumption without compromising the required properties, especially for dentistry applications. The filaments of polymeric materials, namely acrylonitrile butadiene styrene (ABS), poly-lactic acid (PLA), polycarbonate (PC), polyamide (PA), thermoplastic polyurethane (TPU), and polyethylene terephthalate glycol (PETG), have been used in dentistry applications. Hence, this paper attempts to explore the opportunities and existing available facilities of 3D printing technologies in dentistry applications. This review paper also emphasises the necessity of integrating these activities of 3D printing, post-processing time, and cost. Finally, the paper explains the research gap, especially the usage of shape memory alloys and polymers in dentistry applications using 4D printing technology. Most of the problems existing in dentistry should be addressed by the recent technologically advanced 3D printing technologies, and the living society will be getting effectively benefited; however, a few researchers presented their review works over the 3D printing technology in dentistry applications, and hence this work develops the awareness among the researchers to fulfil the needs of dentistry.
Keywords: 3D Printing technologies, Dentistry, SLA, FDM, SLS, influencing parameters, ABS, PLA, PC, Clinical applications
-
INTRODUCTION
In 1986, the first three-dimensional (3D) printing technology was applied to diverse fields for the development of many different manufacturing technologies. Later, the technology of patented Stereolithography (SLA) led to the development of a 3D printing system, and Scott Crump received a patent for fused deposition modelling (FDM) in 1990. In the recent past, 3D printing of biopolymers has been extensively utilised in the medical domain for reasonably addressing the issues raised while using metal-based implants and dentistry-relevant replacements [1]. Additive manufacturing describes the principles that are presently used in various fields, from the aircraft industry to medical-related industries, thus enabling the fast production of intricate products for the development of applicable solution in self-growing robots [2]. There are various types of additive manufacturing technologies, like Stereolithography (SLA), fused deposition modelling (FDM), selective laser sintering (SLS), inkjet printing (IJP), and powder binder printing (PBP), that are used to fabricate the different 3D and 4D models for the daily usage of human implant products [3-9] as shown in Fig.1. Various kinds of materials are used in 3D printers, like polymers, composites, ceramics, and metals and their alloys, to fabricate layer upon layer [10-11]. The creation of complicated structures using 3D printing has been made possible in a variety of dental applications like prosthodontics, orthodontics, maxillofacial surgery, oral implantology, endodontics, periodontology, and surgical devices by utilising the demand for flexibility and better mechanical resistance, and abrasion resistance properties of newly developed materials. The recent development of 3D-printed products is becoming a new follow-up to "Dimensional Variables Printing," which is termed 4-D Printing [12]. Programmable bio-smart materials will change their orientation depending on the surrounding environment [13].
Figure 1 Present 3D and 4D Printing techniques in the Dentistry field
Applications of these 3D printed platforms would enable the readers to appreciate the progress achieved in the developing dentistry field. Computer-Aided Design and Manufacturing (CAD/CAM) technologies led to major improvements in dentistry applications. These technologies in helping produce reliable restorations with accurate dimensions, reducing time and labour costs when compared to traditional methods. It is more economical than that subtractive manufacturing technique in terms of hardware investment and overall production costs.
The thickness, orientation of the printed layer, degree of polymerization, and post-curing process are to be considered the major influencing factors for determining the mechanical and physical properties of the 3D-printed components. 3D printers have a significant contribution to make in producing components with the least price, lead time, and material consumption without compromising the required properties. The filaments of polymeric materials, namely acrylonitrile butadiene styrene (ABS), poly-lactic acid (PLA), thermoplastic polymers, nylon, and polyethylene terephthalate glycol (PETG), have been used in dentistry applications [14-16]. The main purpose of this review is to describe and explore the 3D printing technologies and their characteristics while using various types of polymers, biopolymers, metal-based alloys, and ceramic materials in the field of dentistry. Also, the possibility of using polymeric materials in the 4D printing of maxillofacial surgery-related products and the fabrication of prosthodontics, orthodontics, and maxillofacial oral surgery products has been explored [17].
-
Search Methods
-
-
METHODS AND MATERIALS
A detailed review was conducted, as of now, using 'PubMed' to identify the 3D printing technologies and their relevant materials for making prostheses in dentistry. The keywords used in the above 'PubMed search are: "Dental materials from metals to polymers and 3D printing techniques like SLA, IJP, SLS, FDM, and PBF for dentistry applications". The bibliographies of the obtained references and review articles are used to identify the relevant articles. Subsequently, these articles were screened to extract the qualitative and quantitative results for further analysis.
-
3D Design and Printing
Before initiating the 3D printing process, it needs the 3D design of the required products, so commercially available CAD software like CREO, SOLIDWORKS, CATIA, AutoCAD, etc. are used to develop the model with a high degree of geometric accuracy as per therequired design [18]. Developed models have been saved in '.stl' file format and further sliced using slicing software like Cura, 3D Printer OS, IdeaMaker, KISSlicer, Octoprint, Repetier-Host, AstroPrint, Slic3r, Simplify3D, and IceSL. Among the above, some of the software may be open-source and free. These are compatible with most desktop 3D printers and can work with most files in the most common 3D formats, such as.STL,.OBJ,.X3D, and.3MF. The sliced data are exported to the 3D printing software for checking the parameters and whether the processing capabilities are within the selected 3D printer. Supports could be set based on the density index, size of the specimen, and any allowances required.
Further, check the platform alignment for ensuring the printing accuracy of the objects and orientations on the build platform at different projection angles of 0°, 30°, 45°, and 90°. Secondly, the optimal printing orientation was determined, and compared with the pre-defined filament, powder, liquid, or resin parameters. A commercially available 3D printed materials database will be provided for the required appearance of the 3D products, like white, black, grey, or transparent, as well as their physical, mechanical, and electrical properties.
-
3D Printing Accuracy
The accuracy of the 3D printing was determined by comparing the dimensions of the model to the dimensions set in the printed bars. The measurements are made using a digital Vernier calliper (± 0.01 mm) for the length, width, and thickness of the 3D printed samples. At least three samples are measured for each condition tested; three measurements were taken from the
different samples along with the printed bar, where a single measurement was made for each sample. Finally, calculate the average percentage of errors by specifying a value for each dimension and normalising the printing error to obtain high printing accuracy [18].
-
3D Printing Techniques
The working principles of 3D printing technologies in dentistry applications can be classified into several categories that depend upon the printing methods, material, and its characteristics, merits, and limitations, as selected and listed in Table 1.
Table 1: Impacts of 3D printing methods and materials in dentistry application
3D printing methods
Material characteristics
Materials Used
Merits
Limitations
Ref
SLA
Light curable resins
Epoxy-based materials and Methacrylate monomer
[19- 22]
SLS
Powders
Polymers Ceramics Metals
[23- 25]
FDM
Thermoplastic polymers, Metal alloys with low temperature
and Composites
Wire or Filament form of raw material
[26- 31]
Powder binders printer
Raw materials are available in powders
Metals, Ceramics, Plastics
[32- 37]
Photo polymer jetting
Light curable resins
Bio-compatible photo polymers
resolution (16 microns per layer).
[38- 39]
Digital light projection
Light curable resins
Resins
[40] -
Better product resolution and Less working time.
-
Post-processing is required, Lack of surface.
-
Smoothness and Achieved limited mechanical strength.
-
No external protective environment is needed.
-
No thermal stresses are distributed.
-
The powder itself creates the supports.
-
Excellent mechanical properties.
-
Rapid method.
-
The surface is porous and rough
-
Release. harmful gases
-
Materials waste is high.
-
The raw material powder is high in cost.
-
Post-processing is very exhausting.
-
Filaments of various colours are readily available in a commercial.
-
Cost-effective.
-
Fast production.
-
Low maintenance.
-
Thickness (0.1mm).
-
Gases are not toxic.
-
Discontinuous extrusion may cause a defect.
-
Depth and cuts need a support structure.
-
Delamination at low temperature.
-
Thermal distortion.
-
Safe material
-
Less working time.
-
Low cost
-
Low resolution
-
Low strength
-
Not to be soaked/heat sterilized.
-
Less processing time.
-
A thin layer with high
-
Excellent surface finish.
-
No need for post- processing.
-
High cost and irritant to the body.
-
Excellent surface finish and accuracy.
-
High complex and intricate products are made.
-
Less time.
-
Limited material selection.
-
Skin sensitization.
-
VAT Polymerization-based printing
The first additive manufacturing (AM) technology with SLA was invented by Chuck Hull in 1986, and was applied in medicine as a surgical model for all plastic implant surgery in 1994 [41]. The SLA process uses a laser and computer-aided scanning to build the product's components layer by layer using photo polymerized vat resin. The vat photo polymerization printing technique involves a light of a specific wavelength directed towards photo-curable resin to cure layer by layer at the required time. This rapid manufacturing method employs a light source that influences polymerization to create mounted structures made of highly cross-linked polymers [42]. This printing process consists of the following three major steps: ultra violet (UV) exposure, model-built platform movement, and photosensitive liquid resin refilling.
The first layer of vat resin will be traced by a laser scanner; once the layer has been produced and cured, the build platform will descend downward due to gravity, and this procedure will be repeated. The contact between the resin and oxygen will undergo a process of polymerization when the UV source avoids oxygen interaction. A laser is placed at the bottom to reduce the severity of the occurrence for the operators, and subsequently, a vat of resin will move down to the bottom platform. The supports are generated by CURA software, which will resist the depths and cuts in the models after the final products are created and the supports are manually removed.
(a) SLA printing process
(b) Crown printed along with support structure using SLA
A post-processing process is required to remove the excess resin and support structures before proceeding with the curing process. Hence, the process is quite costlier for mass production of bigger products using the 3D printed implant with a better surface finish obtained for the final products. A laser beam is directed to cure a resin layer, and the platform moves down depending on the thickness of the initial layer generated. The platform lifts the cured layer to allow a fresh layer of resin to flow beneath the cured layer, depending on the laser time provided by its thickness. The printed objects are exposed to UV light for a post-processing process to obtain stability and flexibility and enhance the mechanical properties of the printed samples. A 3D- designed model is used for the fabrication in the SLA method to complete the digitalized form of items by using CAD/CAM software. The structured model layers are fused by a laser scanner of the VA-resin to ultraviolet light, which influences the free radical polymerization of monomers [43]. After the first layer is polymerized, the resin build platform will move down with a computerised scan of thickness, then it will build the adjacent layer of the 3D object as shown in Fig. 2 (a and b). To produce 3D-printed models or items using photo polymerization, the combination of SLA and liquid interaction will be crucial. There are several methods used to manage the variables, such as UV intensity, scanning speed, the number of resin monomers, photo- initiators, and modelling kinetics, to get the desired final product [44].
Figure 2. SLA Printing of Tooth Crown
Nowadays, SLA is used mainly for the fabrication of dental restorations such as denture bases, crowns, palatals, surgical guides, cast models, and prototypes.The merits and demerits of 3D printing by the SLA method in the field of dentistry and maxillofacial surgery are discussed in Table 1. It includes the design flexibility and symmetry of geometry in terms of high dimensional accuracy of dental medical devices. High exactitude in the measurements was retrieved from the patients scanning data using cone beam computed tomography (CBCT), enabling the production of various devices with different technologies [44]. The cytotoxicity of the printed devices may release a discharge of residual un reacted resin monomer, which affects the human body. It provides a better analysis of the methods and guidance to produce dental restoration products in the alternative approaches for the dental diagnosis, treatment, and production of prosthetic devices in oral cavities [45]. It is understood that a limited choice of resins can be used in photo polymerization for making dental products. The introduction of Nanoparticle in the polymer matrix improves the mechanical properties and provides some degree of flexibility [46].
-
Powder-based printing
The various types of powder-based printing methods, namely selective laser sintering (SLS), electron beam melting (EBM), direct metal laser sintering (DMLS), and selective laser melting (SLM), have been promptly utilised in dentistry applications [47].
Figure 3. Selective Laser Sintering process
The SLS is a technique with high-energy electron beams, directed by electromagnetic coils under vacuum conditions, which are used to fuse the powdered raw materials into the bonded particles as shown in Fig.3 [48]. The laser forms a solid layer out of the powder; after that, the platform is lowered to the bottom to make space for the laser to sinter another powdered particle to get the next layer; thus, the process is repeated for the creation of 3D structured objects [48]. This process does not require any additional support during the printing process because the support will be provided by the surrounding powdered particles itself; hence, the post-processing cost will be minimised. Various 3D prototypes have been fabricated for analysing the characteristics during machining, drilling, cutting studies, etc., as presented in Figs. 45 [48]. The main advantages of SLS methods of manufacturing are the usage of antiseptic materials with high mechanical properties, low cost in high volume production, support-free processing, and low post-processing costs. Even though this process may cause severe health-related problems by
causing the inhalation of raw material powders, which leads to severe health hazards, it also demands a high initial investment, i.e., high equipment and setting costs [49-51].
Figure 4. Removable partial Denture framework fabricated using SLS
Figure 5. Partial Denture fabricated by Sintered framework fixed in the Oral cavity
Another challenge is, that the powder gets mushy if the operating temperature exceeds the design. Hence, stringent temperature monitoring and control determine the quality of the 3D printed product. This method of manufacturing does not require structural support for printing, so the processing time is quite longer than that of other AM processes [52 -54]. Metal- based, ceramic-based, and polymer-based are the three varieties of SLS printers that are commercially available.
The first type exploits fine metal powders, but the second type needs micro-grained thermoplastic polymers as raw materials. The metal-based SLS printer uses a metal powder with different grades as required, such as SS-alloys (316, 304L, 309, 174), Titanium alloy (6AI-4V), Cobalt-Chromium, etc. for functional applications. The process requires a vacuum condition to avoid the contamination of oxygen with metal powders that may cause distortion of the final products. But, the polymer-based SLS printer does not require vacuum conditions. The fabrication of products using the ceramic powder-based SLS printer requires a significant amount of vulnerable time to obtain the densification of the products. The different kinds of polymers, metals, and glass compounds are used as binders with ceramic powder. So, the functionality of binders melts the powder bed surface and forms a glassy appearance around the ceramic particles to bond them together. For preventing the oxidation contamination, an inert atmosphere must be maintained inside the fabrication environment. The polymers in the presence of high temperatures lead to firing in the furnace, and inorganic materials remain the same at that temperature [55]. The ceramic-based SLS requires the penetration of stable compression that enhances the mechanical accomplishment of the ceramic materials products by maximising the final compactness [56]. In this process, maintaining the relationship between the laser scanner and the mechanism of melting the powers to solidify is highly difficult, and it needs thermal and residual stress analyses.
The DMLS is similar to the SLS, but the utilisation of metal alloys is higher in the rate of production. Similarly, SLM and EBM use a high-energy electron beam to melt a raw material before printing the final product. Due to the incomplete melting of the powders, the end product has a porous internal structure and a rough surface, which is its principal drawback. The melting process consolidates the powders to create parts with high density and improved mechanical properties [57]. The laser absorption and flow behaviour of the structure in the form of a liquid phase determine the final printed product with the desired properties; hence, it has limited available material for meeting the basic requirements. The major advantage of the melting process is that it produces dense parts, thus eliminating post-processing steps such as thermal treatments or infiltration that are required for the (SLS or DMLS) printed final products [58]. Fused Deposited Modelling (FDM) or Fused Filament Fabrication (FFF) is the most prominent 3D printing method used in the dentistry field [59]. It is a secondary form of 3D printing technique where the cost is quite less than other AM processes [60]. The FDM has an extruder, a printing nozzle, and links. The filament, which is made of thermoplastic materials, can be passed from the extruder to the printer's nozzle, which has a heating chamber. The filament can be melted and transformed into a designated layer with the required thickness, and the deposition of a layer on the table can be started as per the design as shown in Fig. 6 [61].
-
Extrusion-based printing
(a) FDM Process (b) Crown made by FDM
Figure 6. Fused deposition Modeling Process
Every single layer of the products is bonded and forms the final product with greater in homogeneity [62]. In this process, the melting point of the 3D printing material plays an important key role in determining the end quality of the 3D printed product. The inputs come from the desktop computer or laptop, which is directly connected with the 3D printer, so the printing nozzle can move in X, Y, and Z-axis directions accordingly [63]. The material, i.e., filament, is extruded in the form of a molten state from a preheated nozzle and deposited in the platform. The provided tme gap allows us to solidify the material and finally obtain the 3D product by layer-by-layer deposition [64]. Various polymer-based materials like poly-lactic acid (PLA), poly-carbonate (PC), polyamide (PA), acrylonitrile-butadiene-styrene (ABS), and thermoplastic polyurethane (TPU) have widely been used in 3D (FDM) printing industries. Low-melting metal alloys are also utilised in this technique for making 3D-printed components [59, 65]. PLA is biodegradable and thus suitable for dental prosthodontics [66].
The medical-grade, high mechanical-strength, and biocompatible tissue scaffolds in dentistry are constructed using the FDM method by combining polycarpic acid with 3CaPO4 (tri-calcium phosphate) [67]. Direct Ink Writing (DIW) is widely used in recent days to fulfil the demands in the dentistry field [68]. DIW involves either a pneumatic or mechanical piston-based dispensing system to extrude the material with the suspension of materials through a nozzle or syringe. DIW is the most common technique for bio-printing cell suspensions, extracellular matrix-based solutions, and cell-laden hydrogels. A complex structure needs a support structure, so the same material, which has a water-soluble characteristic, can act as a support material, resulting in less cleaning time without damaging the external appearance. The accuracy of the 3D-printed objects is based on the speed of the extruder, the nature of the material flow, the size of the nozzle, the thickness of the layer, and the metallurgical properties of the materials. One of the primary advantages of FDM printers, also known as "home" 3D printers, is their low cost.
The polymers, if reinforced with Nanoparticle, result in improved functionalities. The mechanical properties of the printed objects are influenced by various parameters, namely raster angle, infill density, print orientation, stacking sequence, extrusion temperature, layer thickness, solidification rate, the melting point of filament, nozzle travel speed, and bed temperature. The FDM technique can be used to print the temporary crowns and bridges in the prosthodontic field, as presented in Fig. 6 (b).
-
Powder binder printer
Binder jet 3D printing, also known as powder bed and inkjet printing or drop-on-powder printing, is a rapid prototyping technology used to create objects described by digital data. In this process, the liquid adhesive droplets are ejected from the inkjet head, releasing droplets that infiltrate a layer of powder on the build platform. The levelling of the layer is formed with the fusion of powder and inkjet, then the powdered bed will lower the platform and the next step takes place to complete the process. As shown in Fig.7, the levelling roller will roll off the surface of the formed layer, and the powder will be taken to another build layer, and the process will be repeated to obtain a subsequent layer. In the field of dentistry, it is used to fabricate intricate parts for working cases and prototypes. The printed objects are delicate in nature and lack accuracy because of low-cost technology, and powder materials are very difficult to handle, so this technique does not require any sterilisation for the printed cast materials [69-70]. The laser light is focused on the metal layer to convert it from the metal state into a plasma state. This technique uses a liquid binding agent called an adhesive, which is jetted from the printer head to bind the different powder particles like ceramics, polymers, and metals. Infiltrating a layer of powder forms a layer-by-layer new product, and it will impinge on liquid and powder to bind particles. The individual layer of the designed model is built up by sprinkling fine powders over the platform. The model is supported by an un-penetrated powder structure that is left over on the platform surface, so it doesnt require the support materials. The post-processing is done with the help of cyanoacrylate or epoxy resin, which will help to improve the mechanical properties.
-
Powder binding printing (b) Denture model by powder binding
Figure 7. Powder Binding Process
-
-
Photopolymer jetting
The photopolymer jetting technique is a combination of the high-powered printing head and photo polymerized polymer vat resin. The UV-sensitive polymer is ejected to the platform surface through the printing head and cured beneath the descending platform as shown in Fig.8. If a supporting structure is fragile, it can be easily removed from the final objects in the photopolymer jetting process [71]. This process is capable of producing silicone-based material with a high resolution of 16 microns [72]. These printed products are used in the dentistry field as tooth crowns or anatomical study models. The primary benefits of this method are quick processing and low-cost, high-quality production of complicated structures with smooth surfaces [73]. A 3D jet printer operates independently in both directions and has several printing heads to cover the working platform width.
Figure 8. Photopolymer Jetting Process
A UV light source is used to harden the resin layer, which is ejected from the printer head. The main drawbacks of this printing technique are: difficult to remove the material completely due to the rigid support material ejected from the nozzle, which causes skin irritation.
-
Digital light projection (DLP)
-
The DLP is a photo curing method that utilizes the same basic principles as the SLA process. The 3D model is printed layer by layer using liquid photosensitive resins, with the subsequent layers being built up on top of the preceding layers. The complete building layers are cured by the projected light source from the DLP 3D printer within the clear resin tank across the platform. After each layer has been hardened, the entire part is assembled by moving a build platform based on the layer thickness using a computerized projector screen. A digital micro-mirror is placed beyond a laser projector to reflect the light and form different configurations for individual layers [74].
As seen in Fig.9, SLA employs a UV laser beam as a light source, whereas DLP uses UV light from the digital lens's projection source. The UV light source is static, and it cures each layer of the resin at the same time so that the curing is accurate and the quality is better in SLA compared to DLP. The intensity of the light source in the DLP 3D printer is versatile.
Figure 9. Digital Light Projection Process (DLP)
The effect of light on the resin can be controlled by the operator, and it allows for the rapid printing of larger parts, whereas SLA printing produces more accurate parts with intricate functions [74].
-
MATERIALS USED IN 3D DENTISTRY APPLICATIONS
-
Polymers based Materials
-
Vinyl polymers
Vinyl polymer is the most commonly used polymer in oral and facial surgery components because of its properties, biocompatibility, and degradation characteristics. To accommodate a variety of material qualities and manage the molecular weight of the vinyl polymer in chain functional groups, synthesising, a controlled radical polymerization (CRP), has been applied. Vinyl polymers are utilised in the dental 3D printing technologies like as SLS and photo polymerization (SLA) [75-77].
-
Poly Methyl Methacrylate (PMMA)
PMMA is generated from the vinyl polymer through the process of polymerization, and this polymer is most frequently used to fabricate the dental product via 3D printing technology. It is created by anionic polymerization using Iso-butyronitrile or n-butyl lithium as the initiators, starting with methyl methacrylate monomer. Because of its ease of manufacture, flexibility, low cost, lightweight, stability in the oral cavity, and enhanced esthetical features, PMMA is usedfor printing denture base goods. It does, however, have poor surface characteristics and mechanical qualities. However, it has poor surface properties and low mehanical properties. So reinforcement of hard particles with matrix materials results in improved properties.
-
Styrene polymers
Acetate-butadiene-styrene (ABS) and polystyrene (PS) are the two main forms of styrene polymers used in making dental components with ABS. Through FRP and the use of benzoyl peroxide as an activator, styrene monomers are converted into polystyrene, an aromatic hydrocarbon. It has a structurally amorphous structure, high flexibility and transparency with a smooth surface, high impact resistance, and better mechanical properties [78-79]. Different initiators like t-butyl or cumene (OH)4 or sodium formaldehyde, sulfoxylate dehydrate, ammonium persulfate, and Na(SO)2 redox are usually preferred to improve the biomechanical properties.
-
Polyesters
Polyester is a class of thermoplastic polymers with ester groups in the chain that serve as a functional set for polymerization [80, 81]. They are mechanically tough, amorphous, and transparent, and they polymerize via poly-condensation, i.e., the removal of water molecules. The other forms of polyester, namely polycarbonate (PC), polycaprolactone (PCL), and polylactic acid (PLA), are also used in dentistry applications. The PC is polymerized by a condensation reaction between bisphenol and carbonyl chloride or diphenyl carbonate. It is used to manufacture dental products like orthodontic brackets, denture bases, and provisional crowns by FDM. However, the PC combined with bisphenol releases a harmful substance unlike other compositions [82]. The PCL is one of high mechanical strength, low melting point (63°C), biodegradable, hydrophobic nature, and biocompatible polymers; hence, its influence in dentistry is unavoidable. It is employed in bone tissue regeneration, such as alveolar bone augmentation, and is produced from lactic acid via poly-condensation using ring-opening polymerization [83]. Due to its great biocompatibility, PLA is used in the FDM printer to manufacture drill guides for surgical insertion, temporary restorations, and crown preparations. [84].
-
Polyether-Ether Ketone
Polyether-Ether Ketone (PEEK) was developed by the Du Pont Company in 1979. It belongs to the group of thermoplastics that require extensive post-processing and lack precision, with a melting temperature of 343°C. The structural changes in this polymer result in improved biomechanical and biological properties. It exhibits a different melt index and molecular weight if you introduce Nanoparticles such as SiO2, and Al2O3 to the matrix. The modified bioactive surfaces of PEEK materials are generally used in implantology for facial, and cranial reconstructions and dental prostheses. The PEEK has a glass transition temperature of 143°C, and a maximum level of 48% crystallisation. It has high tensile, bending, and impact strengths. It is dimensionally stable under a heated environment, has good sliding and wear behaviours, has high resistance to chemicals, radiation, and hydrolysis except for sulfuric acid, and has high in-vitro biocompatibility, i.e., is non-cytotoxic. Carbon fibre-reinforced PEEK (CFR-PEEK) has a high modulus of elasticity and is also used in dentistry applications.
-
Polyphenylene Sulfone (PPSU)
The manufacturing of dental products using thermoplastic polyphenylene Sulfone (PPSU) polymer is widely available in the market, and this can effectively be utilised in 3D printing techniques for medical applications. However, now this material has come into the limelight in the dentistry domain despite its poor mechanical properties. The PPSU polymer is one of the amorphous materials (solid-state), and its crystallinity structure has a significant impact on material properties. The amorphous phase of this polymer has good optical properties and the consistency of printed parts, which helps in dental restorations. It has higher flexural strength and wear resistance properties when compared to PEEK, which emphasises its use in the dental field for both removable and fixed dental prostheses.
-
Polyamides (PA)
Since 1936, PA has been produced industrially. There is a wide range of PA that is recognised, and adjustments are possible. It is therefore appropriate for a wide range of technical applications. High strength, rigidity, and hardness are the defining characteristics of PA. It exhibits dimensional stability when heated and has excellent electrical insulating qualities. One can observe a high damping capacity, a high resistance to fuels, lubricants, and solvents, as well as a high resistance to wear. PAs can be biocompatible. The work piece's moisture content affects the mechanical properties of the material. Its structure determines its colour: aromatic PAs are transparent like water, whereas aliphatic PAs are semi-crystalline or opaque. The monomer of PA 12 -Aminoundecanoic acid is well known that functional, filling, and reinforcing additives are used. The melting point is lower, and the material is softer. The degree of crystallinity of a material is inversely correlated with the rate at which it cools during processing; the higher the degree of crystallinity, the better the material's mechanical properties and resistance to abrasion.
-
Natural polymers/bio-polymers
Aliphatic polyesters made of polycondensed lactic acid or polyglycolic acid are known as polylactide (also known as polylactic acid, PLA). Due to PLA's melting range of 160 to 220°C, heat table printing plates are not necessary during the processing. Biopolymers are another name for PLA (degradable polymers made from renewable raw materials). The thermoplastic PLA has a semi-crystalline structure and is highly resistant to moisture, alcohol, and lubricants. The modification determines whether it is rigid or flexible. It might look transparent, but it can be coloured, and its surface is glossy.
-
Polyvinyl-alcohol
Polyvinyl alcohol (PVA) is a polymeric material usually utilised as a supporting filament in FDM 3D printers to form complex structures. This polymer is widely used in the biomedical field because of its good physical properties, biocompatibility, and water solubility. Instead of multiple patient visits, intraoral optical impressions and a CAD/CAM milling system are used to trace out and fabricate dental restorations during a single patient visit. Fig.10 represents a new concept for the fabrication of an indirect crown using an FDM 3D printer with PVA filament and a chairside to fabricate a dental model for crown preparation with PVA [85]. A study was conducted to compare the accuracy of the models and crowns with digital as well as conventional prosthetic methods [8586].
However, because PVA is a water-soluble polymer, the fabricated crown was immersed in water for 10 minutes to completely dissolve the PVA model. The crown should be removed immediately with a strong stream of water, then examined to evaluate the accuracy of the PVA model and the fabricated restoration.
Figure 10. Fabrication of crown using PVA by FDM printer
-
Recent developments in the field of dental polymers
-
-
Due to the rising demand, new and improved material developments in the field of polymer AM are currently being made. Biocompatibility and the ability to use materials not just momentarily in oral settings appear to be of utmost significance in material development. There is a dearth of suitable dental polymers, particularly for the manufacture of dental prostheses, that can last for an extended period of time in the mouth (at the moment, no material is suitable for intraoral use for more than 12 months). There are currently very few additively manufactured polymers that have been authorised for temporary dental applications. Based on their chemical makeup, they can be divided into two groups: dimethacrylates and urethane
dimethacrylate; monomethacrylates or acrylic resin (polymerization induced by light). The manufacturers frequently do not provide the specifications of he provisional AM polymers. Therefore, a comparison between these materials and traditional dental application materials is necessary.
Additionally, potential materials must be capable of being processed using AM technology while also integrating the physical characteristics needed for the finished product. Mechanical qualities like flexural strength, hardness, impact strength, and colour stability are crucial for the prolonged use of temporary dental restorations. Rimell and Marquis have provided an illustration of the advancement of materials. They created an ultra-high molecular-weight polyethylene for the selective laser sintering production of dental workpieces. The material did, however, exhibit significant porosity and shrinkage. In order to successfully use other starting materials (higher-density powders), they came to the conclusion that the creation of composites made of biocompatible supramolecular polymers (PCL) for use in regenerative medicine was necessary. They note that the best printing results in terms of resolution were obtained by replacing the initial polymer with a terminal benzyl group. The test series was finished with additional modifications using hybridization with silicate particles.
All polymers created were cytotoxic and biocompatible according to ISO 10993-5 and 10993-12 standards. For this reason, conventional dental polymers are copolymerized with positively charged terminal alkyl chain monomers, which are essential for the antibacterial effect. The printed objects' mechanical characteristics were remarkably similar to those of products made without the addition of antimicrobial monomers. Instead of an antimicrobial agent being released, the antimicrobial effect is based on the contact inhibition of the bacteria. In their investigation of the 3DP, Steinhaus et al. used a liquid binder made of 2-hydroxyethyl methacrylate (HEMA) and phenyl ethylene (styrene). It has been demonstrated that the concentration of styrene has a significant impact on the polymerization reaction. It lessens thermal stress and makes it possible for the material to reduce stress. The polymerization process is significantly influenced by the binder's age. The development of photo initiators, along with the advancement of polymers, accelerates the polymerization process and improves its speed, which in turn enhances the material properties of finished dental products.
-
Metal-based Materials
-
Stainless Steel Alloys
The 302 stainless steel (SS) alloy, initially developed with similar properties to vanadium steel, was implanted in the human body. The SS alloys like 316L, 316, etc. that have advanced properties of corrosion resistance, wear resistance, and biocompatibility have been widely used [87, 88]. A protective surface film of Cr2O3 takes responsibility for introducing the corrosion and wear resistance of the fabricated biomedical components that are to be implanted inside the human body environment. The presence of Ni (1720%) helps to stabilise the austenitic phase at room temperature, whereas Cr and Ni in higher concentrations develop allergic reactions in patients, making this type of stainless steel unsuitable for permanent implants in the human body [89]. Stress, corrosion, cracking, and pitting corrosion may have taken place if the implant metal used did not possess sound mechanical qualities. Deep pits form on the metal surface after breakage, weakening the spots more than an oxide surface layer that propagates through the bulk material [90].
-
Titanium and its alloys
Commercially pure titanium (CP-Ti) and Ti-6Al-4V are recommended for the manufacture of orthopaedic implants due to their high corrosion resistance, biocompatibility, strength, and lightweight [91]. Natural bone has a lower Young's modulus (1030 Gpa) than a thin passive oxide coating on the surface, which makes it unsuitable for orthopaedic application since natural bone generates stress shielding and bone resorption, which causes implant loosening [92]. Surface hardening and surface coating are required for Ti-6Al-4V alloy; otherwise, the presence of vanadium or aluminium results in lower wear resistance and greater destructiveness [93]. Titanium alloys with lower moduli of elasticity, such as Ti-12Mo-6Zr-2Fe, Ti- 15Mo5Zr-3Al, Ti-15Sn-4Nb-2Ta-0.2Pd, and To-13Nb-13Zr, are embrittlement in nature and are easily susceptible to oxygen diffusion, resulting in oxygen dissolution.
-
Cobalt-based alloys
-
Cobalt-based alloys are used to manufacture medical implants because they have excellent corrosion resistance, wear resistance, biocompatibility, and low impact resistance. The first cobalt-chromium-based alloys were used in the biomedical field in 1930, i.e., manufactured and tested to fix implant products that have better refinement structures for the human body [94]. Four types of Co-Cr-based alloys are commonly used to fabricate the bio components, namely cast CoCrMo alloy (F75), wrought CoCrWNi alloy (F90), wrought CoNiCrMo alloy (F562), and wrought Co-Ni-Cr-Mo-W-Fe alloy (F563) [95]. These bio-compatible materials have high corrosion and wear resistance abilities with higher tensile and yield strengths. Additionally, these alloys inclusion of oxide-forming elements like Cr, Mo, and Co ought to be thermodynamically conducive to the development of a thin passive oxide coating when exposed to bodily fluids.
It is better suited for human implants due to the formation of a protective oxide layer over the surface, which is composed of Cr2O3 [96]. Cobalt is an unstable face-cantered cubic system with very low stacking fault energy. These alloys will exhibit their changes in the crystal structure from an unstable face-cantered cubic (FCC) to a hexagonal close-packed (HCP) crystal structure at high temperatures. The kinetic energy stabilises from FCC to HCP throughout the slow cooling process at ambient temperature. So, it will create momentous improvements that will lead to high yield strength and similarly reduced fatigue damage under cyclic loading.
-
Ceramic Based Materials
-
Zirconia
A pure version of zirconia (Z-System) became commercially accessible in 2004, and since then zirconia has been used in the development of dental implants as a metal-free ceramic [97]. For treating osteoblast adhesion and bone-implant Osseo integration, zirconia-based implants are highly used because of their biocompatibility with the soft oral tissues. A bio-inert surface will reduce the contaminants on the surface and become a bioactive surface to promote surface hydrophilicity in nature. A variety of techniques, such as oxygen plasma surface treatment, acid etching, UV irradiation, and hydrogen peroxide treatment, will have a stronger affinity for human body conditions. The Yttrium-stabilised zirconia (3Y-TZP) powder mixed with polymethylmethacrylate (PMMA) creates a homogenous hybrid sol, i.e., 'ink'. To get an improved material fixation in dental areas, the production of this 'ink' can be utilised for making dental accessories by using photo-polymerized techniques.
-
Alumina
-
Alumina (Al2O3) is one of the ceramic materials that have been used in dental applications. It exhibits different forms namely , , , , , , and . Among them, the most thermodynamically stable form is alpha-(Al2O3) [98]. It has good wear resistance and is biocompatible, but it is less compact and has less flexural strength compared with zirconia. Zirconia blended on an alumina matrix can be infiltrated to create a mineral composite that offers excellent toughness and fatigue resistance and controls the grain size, porosity, number of sinter cycles, sintering temperature, time, high temperature, and low temperature cooling rates with the addition of stabilisers like zirconium oxide, magnesium oxide, or chromium oxide [99100]. Alumina with a submicron grain size for dental applications has better mechanical and optical prperties. Various reviews of the literature were collected to learn about studies on polycrystalline alumina ceramics that provide a grain (1 m), a porosity of 0.7
%, and a similar degree of translucency to commercial translucency porcelain [101].
-
Crown and Bridge Dentures
-
-
CLINICAL APPLICATIONS
The crown structures are transformational changes of phase in fixed dentures, but they should fulfil the mechanical, biological, and esthetical requirements [102]. It has several functions, like protecting periodontal tissues, reducing the displacement of teeth, and maintaining prosthodontic function. It is effective for many purposes, including maintaining the prosthodontic function, preventing tooth displacement, and safeguarding periodontal tissues. The basis of dentures can be created utilising resin-based 3D printing techniques like SLA or DLP when there are restrictions on the machining equipment and material qualities for prosthodontics and orthodontics [103]. As shown in Fig.11, 3D printing uses fewer materials without any material loss than milling does. Additionally, a range of materials can be employed to produce favourable products at the same time. A good fit of a crown and denture needs good mechanical stability, durability, and the health of surrounding soft tissues [104]. Sometimes it may have some unfavourable issues like bacterial and fungus accumulation, less leakage of adhesive, colour changes in the tooth, a lack of aesthetics, sensitivity, dental caries, and orthodontic disease. The temporary crowns are fabricated using 3D printing, and excellent edges, internal fit, and accuracy are obtained by applying CAD/CAM and the interior of the temporary crown to the occlusal area [105].
-
Fabricated by 3D printing (b) Fabricated by subtractive manufacturing
Figure 11. Difference in surface smoothness of crown fabrication
-
COMPLETE DENTURE BASE
The 3D printing technology involves the CAD data and rapid creation of a digital model for the fabrication of a complete denture base without the need for any substituted products like moulds, cutting tools, and tooling fixtures, as shown in Fig.12. Subtraction technology combined with traditional heat-curing and self-curing methods can also be used to fabricate complete dental-related orthodontics and prosthodontics areas [105]. Tooth loss can occur as a result of trauma, fracture, periodontal disease, or caries.
There are several ways to replace lost teeth, including with whole or partial detachable prostheses, bridges supported by neighbouring teeth, and crowns supported by implants. Traditionally, an alginate or polyvinylsiloxane imprint was made
before a prosthetic device was built. The next step is the creation of a model for intricate laboratory work by a qualified dental technician. The production of dental prosthesis has been dramatically altered by the introduction of CAD/CAM technology.
The construction plan is divided into three phases. The first phase entails taking virtual impressions with intraoral and extra oral scanners and recording the patient's occlusion. The PMMA component is used in the compression moulding technique for making dentures, which has the advantages of being faster and less error-prone.
Figure 12. Acrylic resin-based complete denture with acrylic teeth
The design and development of complete dentures based on various manufactured product applications is still being researched to better understand the physical circumstances.
-
REMOVABLE PARTIAL DENTURE FRAMEWORKS
To create a partial prosthesis frame, removable partial denture (RPD) frames are processed using CAD/CAM analyses employing an intraoral or extra oral scanner to scan impressions using CBCT and turn the work into a digital file of a standard tessellation file (STL). Further, the STL files are directly transferred to CAD software for the analysis of parameters in designing aspects, through which the process parameters are calculated, and the input generates customised structures as shown in Fig.13 [106107].
Figure 13. CAD/CAM process of constructing a partial Prosthesis framework
In comparison to contemporary digital approaches, a waxing procedure like an investment pattern or refractory cast dental deformity applications may result in impoverished cast products. The denture base and prosthetic teeth can provide contact pressure and lessen bone resorption, thanks to the utilisation of 3D printing technologies in rapid product development.
-
OCCLUSAL SPLINTS
By modifying the occlusal distance between the upper and lower dental jaws inside the oral cavity, the occlusal splint, an intraoral device that can change its therapeutic qualities, is also used to treat transient mandibular joint diseases, as shown in Fig.14. In the conventional method, metal joints are framed using different imprint trays to obtain tooth structure and create a manufactured model to produce splints. Inter-occlusal wax dentition for the upper and lower jaws is created using working models, and alginate impressions are used to cast the dental teeth to mark them. This approach is expensive and produces mistakes in the creation of castings or impressions [108].
-
CUSTOM TRAYS
Figure 14. Occlusal splints/ aligner
Using the custom trays, an implant imprint is taken to create prostheses for precise edentulous impressions with good support, stability, and restored function to safeguard the health of oral tissue. For taking precise impressions, impression trays must be flexible and securely fixed in the mouth. It should have a uniform thickness and enough space for imprinting ingredients. The fabrication method for the custom trays depicted in Fig.15 uses CAD software and 3D printers along with a straightforward application for design analysis. The 3D printers perform multiple tasks to improve their efficiency of workflow with simplified denture supports to take an accurate impression to complete the edentulous patients [109]. The custom trays must meet the requirement of bonding strength between the trays and imprinting material with the human body [110]. An important indicator is the precision of custom trays. The space set aside for the impression materials is precisely controlled with only a small amount of deviation when using custom 3D printing trays, improving accuracy and reproducibility. In comparison to traditional hand-made custom trays, custom trays offer a considerable improvement in accuracy with a suitable range of extensions and a more homogeneous 3D area of the impression material.
Figure 15. Custom tray for a complete-arch open impression by FDM
The printing procedure should be completed in a shorter amount of time to produce correct impressions since the impression materials will prevent distortion during casting [111]. Custom trays, which are becoming more and more popular, can be created using 3D printing thanks to their high precision, quick processing time, ease of programming, and personalization. Almost all printing work in the creation of custom trays can be done by equipment with the aid of CAD software and 3D printers. The versatility of three-dimensional printers allows for increased productivity. Therefore, for patients who are completely edentulous, 3D printing technology can streamline the process of creating direct implant-supported dentures.
-
CROWNS AND BRIDGES
The creation of dental bridges and crowns is one of the most alluring uses of 3D printing in dentistry. Dental implants can be made functional and tooth-like using crowns, or they can be used to protect the underlying tooth structure. Crowns are frequently used to repair lost teeth by serving as an anchor (also known as a tooth abutment). In recent years, 3D printing technology has been used to create crowns and bridges. Additionally, using SLA printing technology, inexpensive 3D printers have been utilised to create more accurate temporary crown and bridge restoration.
-
ORTHODONTIC APPLIANCES AND ORTHOTICS
Exciting possibilities exist for creating orthodontic appliances that fit the teeth, like Invisalign aligners. Because they are clear and can be taken out for eating, drinking, and cleaning your teeth, aligners are a preferred alternative to braces for the treatment of moderate malocclusion. Using an intraoral scanner, the orthodontist creates a digital impression of the patient's upper or lower arch for the 3D manufacturing of clear aligners. The model for thermoforming the aligner is then printed using Stereolithography, often known as FDM. While maintaining the quality of the aligners, 3D printing shortens the production process. Malocclusion and temporomandibular joint issues are treated with orthotics. Additionally, 3D printers and intraoral scanners can be used to build these products. Silica and hydroxyapatite, two Nano fillers utilised to strengthen these appliances, are not able to attach to the resin during SLA. In response, methacrylate functionality was added to the surface of HAp to enhance the adherence of the Nano filler to the polymer matrix. Additionally, the Nano fillers have been functionalized with quaternary ammonium salts to add antibacterial and antifungal characteristics. As a consequence, the orthotics had better mechanical and antibacterial qualities.
-
ORAL IMPLANTOLOGY
Utilizing 3D printing technology in oral implantology aims to transform the field from one that relies solely on experience to one that is precise and digital. They can greatly reduce the technical difficulty and risk, streamline the medical
treatment process, and boost dentists effectiveness. This module primarily introduces applications in oral implantology, such as creating surgical guides and printing personalised trays in three dimensions.
-
ORAL AND MAXILLOFACIAL SURGERY
Due to advancements in 3D scanning technologies, including intraoral and extra oral scanning, cone beam computed tomography (CBCT), and other CAD/CAM technologies, the area of maxillofacial surgery has experienced significant progress in 3D printing. The numerous unique advantages of three dimensional printing technology in surgery can help enhance the symmetry and functional results of craniomaxillofacial plastic surgery treatments. Jacobs and Lin have provided a concise summary of applications in the craniomaxillofacial region, including surgical guides, occlusal splints, and implants. The use of 3D printing technology in RPD manufacture enables the denture base to offer more consistent contact pressure and thus reduce the danger of long-term bone resorption.
Recent research has shown that SLM can provide an RPD framework that is suitable for clinical use. When cast or milled RPD frames were put up against SLM Co-Cr alloy frames, it was found that the former was deemed to have higher mechanical qualities and organizational capacities.
-
SURGICAL GUIDES
Surgical guides can dramatically lower operation mistakes, enhance the accuracy and speed of clinical procedures, and boost treatment efficacy. The implant prosthodontic technique should be understood by patients to increase predictability for dentists. The surgical guiding systems comprise both dynamic and static systems. Dynamic guides transport digital surgical plans to the operating room via mechanical or optical technologies and display the process in real time on a screen. Static surgical guidelines, known as SLA guides, are 3D-printed in the lab using holes made in the jaw models. Because it doesn't move while the patient is having surgery, the static surgical guide differs from the dynamic surgical guide.
The process for creating a static surgical guide has changed significantly. Conventional surgical guides are built using panoramic x-ray images. However, due to the expansion, distortion, and lack of clarity in the radiation picture, the surgical guides cannot be generated, making the preoperative plan erroneous and untrustworthy. After the treatment planning is complete using the preoperative planning software, SLA may create the surgical guides. The drill guides properly communicate this information to the patient via the simulated plan and establish a link between the planned and real operations by displaying the implant's insertion location, angles, and depth. Due to the advantages of a less complicated approach and cheaper investment costs, static methods are commonly chosen as the preferred method to perform direct surgery. The SLA standards, in comparison to dynamic recommendations and traditional guides, have many advantages.
-
-
-
4D PRINTING IN DENTISTRY AND MAXILLOFACIAL SURGERY: CHALLENGES AND FUTURE PERSPECTIVES A 4D printing technology can give 3D-printed things responsive and active features. Different stimuli, including light,
can cause it to modify its structure. Fortunately, by theoretically modelling the structural changes with simulations and analytical computations, the structural changes are very predictable. In other words, we are aware of the effects that any external stimulus will have on the substance. These materials have significant promise for the dental field. Nickel-titanium arch wires, one of the most frequently used materials in orthodontics, have both advantages and disadvantages. Shape-memory polymers may be used to get around some of the drawbacks of these shape-memory arch wires.
This material is more suitable for both the patient and the practitioner due to its superior aesthetics, an improved elastic modulus, improved mechanical and chemical stability, and the capacity of the arch wire to self-adjust during treatment. Endodontics is another industry that could profit from 4D printing. One of the most typical issues that arises during root canal treatment is instrument separation. Pontic fitting may be less than ideal when bridge pontics are permanently seated in prosthodontics while the surrounding hard and soft tissues alter. Given their capacity for self-adjustment, shape-memory polymers may be useful in these circumstances as they help prevent further difficulties. Due to bone resorption brought on by tooth extraction and denture stresses exerted on the bony ridge, full or partial removable dentures are usually difficult for patients. Relining dentures is, therefore, a routine process. Dentures created via 4D printing may be better able to adapt to shifting hard and soft tissue profiles if shape-memory polymers are used. The most often-used materials in implantology for creating oral implants are titanium alloys. Despite the various benefits of titanium alloys, reports of surface deterioration and hypersensitivity have been made. Instead of using titanium alloys, biocompatible shape-memory polymers may improve some implant attributes but not implant strength. For instance, SMPs are more tunable, have remarkable elastic deformation capability, are cost and time effective, have low density (leading to lower weights), are simple to produce, have more programming freedom, and are superior at biocompatibility and biodegradability.
Shape-memory polymers are advantageous because they completely fill the root canals, preventing the development of biofilm, reinfections, and periradicular illnesses. They exhibit the typical expansion and contraction of teeth in order to close the contact space and minimise microleakage. Shape-memory polymers provide biocompatible breakdown products as opposed to metals, which produce metal ions that can result in chronic disorders. Shape-memory polymers, at least in this use in dentistry, show remarkable stability and much improved efficacy due to qualities far outweighing other types of materials.
-
CONCLUSIONS
This paper has extensively collected the data of the 3D printing of dentistry products and presented it in a systematic way for the readers to understand it without ambiguity and identify the new pavement for carrying the real and society-needed
research activities in the dentstry domain. The details of 3D printing technologies that have been adopted to fabricate the dental components, the various materials of metal, polymer, and ceramic that are associated with them, and the various clinical applications are presented.
Before developing the dentistry models by CAD, it is essential to understand the major influencing parameters and their impacts on 3D printed accuracy, strengths, required mechanical, chemical, and biocompatible properties of materials, post- processing, ageing activities, capital investment involved, and operational cost. So, this paper elaborately reviewed the 'required essential knowledge in the applications of 3D printing and its materials in dentistry.
The clinical uses of 3D printing in dentistry were discussed in the concluding section of this review study. These uses included functional prototypes, applications for prosthodontics, oral and maxillofacial surgery, and oral implantology. Additionally covered in the research is the commercially available 3D printing of restorative dental materials, which offer sufficient mechanical qualities to accurately fabricate the components of intra-oral applications of temporary restorations. The review article ultimately highlighted the following difficulties when using 3D printing in the dental field:
-
To effectively apply the existing or develop a new meta-heuristic bio-optimization algorithm for finding the optimum parameters to obtain the 3D-printed product with the required sound quality as per demands in the dentistry domain.
-
A complete analysis software is required to analyze the dimensional tolerances of the components involved in the dental assembly.
-
It is essential to develop a completely automated post-processing system for 3D printing technologies to reduce the lead time and be ready for the challenges involved when considering the newer materials revolution.
-
The integration of a CAD file, .stl file, 3D printer, 3D scanner, computed tomography, cone beam computed tomography, etc. results in a reduction of lead time, less labour cost, low rejection, and high accuracy of 3D-printed products with sound properties [112].
-
Provide adequate training modules with proper training for the personnel who are involved in the 3D-printed activities to strengthen the rate of return mechanism.
-
Limited availability of materials for 3D printing technologies significantly affects innovations.
-
The 4D printing technology using shape-memory alloys and polymers/Nanoparticle mixed together should lead the dentistry domain to a new horizon.
ACKNOWLEDGMENTS
The authors greatly acknowledge the support rendered by the P.G. Manufacturing Engineering Department of Mechanical Engineering, Government College of Technology (GCT), Coimbatore, India, and the respectful soul of Sri G.D. Naidu for having given the land and building at GCT without any expectations to provide a good education to the children from the socially disadvantaged society.
There is no conflict of interest.
Funding Interest
CONFLICT OF INTEREST
The authors declare that no funds, grants, or other support were received during the preparation of this manuscript.
Availability of Data:
The authors have promptly cited the referred papers in the references, and all data are readily available and ready to share if demand arises.
Contribution of Authors
Sekar Tamilperuvalathan1 Conceiving idea, collection of literature, writing, editing
and analysis
Haritha Kaliyaperumal Rabindranathan2 Collection of literature work and written
the review paper Rajeswari Balakrishnan3 Editing and reviewing the paper Prabakaran Jayaraman4- Reviewing and analysing the content Surendran Ramakrishnan5 Consolidation of journal paper
REFERENCES:
[1] C.H. Groth, N.D. Kravitz, P.E. Jones, J.W. Graham, W.R. Redmond, Three-dimensional printing technology, J. Clin. Orthod. 48 (2014) 475-485. [2] Ali Sadeghi, Emanuela Del Dottore, Alessio Mondini, Barbara Mazzolai, Passive morphological adaptation for obstacle avoidance in a self-growing robot produced by additive manufacturing, Soft Robot. 7 (2020) 85-94. [3] A. Wad, S.J. Trenfield, J. Gaisford, A.W. Basit, 3D printed medicines: A new branch of digital healthcare, Int. J. Pharm. 548 (2018) 586-596. [4] J.W. Stansbury, M.J. Idacavage, 3D printing with polymers: Challenges among expanding options and opportunities. Dent. Mater. 32 (2016) 5464. [5] D.Y. Kim, J.H. Kim, H.Y. Kim, W.C. Kim, Comparison and evaluation of marginal and internal gaps in cobalt-chromium alloy copings fabricatedusing subtractive and additive manufacturing, J. Prosthodont. Research. 62 (2018) 5664.
[6] D. Chytas, M. Piagkou, E.O. Johnson, Can three-dimensional visualization technologies be more effective than cadavers for dental anatomy education? Anat. Sci. Educ. 13 (2020) 664665. [7] J.C. Ruiz-Morales, A. Tarancón, J. Canales-Vázquez, J. Méndez-Ramos, L. Hernández-Afonso, P. Acosta-Mora, J.R.M. Rueda, R. Fernández- González, Three dimensional printing of components and functional devices for energy and environmental applications, Energy Environ. Sci. 10 (2017) 846859. [8] C.K. Chua, Rapid Prototyping: Principles and Applications, third ed., World Scientific Publishing Co, Singapore, 2010. [9] X. Li, B. Xie, J. Jin, Y. Chai, Y. Chen, 3D Printing Temporary Crown and Bridge by Temperature Controlled Mask Image Projection Stereolithography, Procedia. Manuf. 26 (2018) 10231033. [10] Vojislav Petrovic, Juan Vicente Haro Gonzalez, Olga Jordá Ferrando, Javier Delgado Gordillo, Jose Ramón Blasco Puchades & Luis Portolés Griñan, Additive layered manufacturing: sectors of industrial application shown through case studies, Int. J. Prod. Res. 49 (2011) 10611079. [11] T.D. Ngo, A. Kashani, G. Imbalzano, K.T.Q. Nguyen, D. Hui, Additive manufacturing (3D printing): A review of materials, methods, applications and challenges, Compos. Part B Eng. (2018) 172196. [12] W. Zhou, Z. Qiao, E.N. Zare, J. Huang, X. Zheng, X. Sun, M. Shao, H. Wang, X. Wang, D. Chen, J. Zheng, S. Fang, Y.M. Li, X. Zhang, L. Yang, P. Makvandi, A. Wu, 4D-printed dynamic materials in biomedical applications: chemistry, challenges, and their future perspectives in the clinical sector, J. Med. Chem. 63 (2020) 8003-8024. [13] E.O. Olakanmi, R.F. Cochrane, K.W. Dalgarno, A review on selective laser sintering/melting (SLS/SLM) of aluminium alloy powders: processing, microstructure, and properties, Prog. Mater. Sci. 74 (2015) 401477. [14] T.S. Jang, H.D. Jung, M.H. Pan, W.T. Han, S. Chen, J. Song, 3D printing of hydrogel composite systems: recent advances in technology for tissue engineering, Int. J. Bioprint. 4 (2018) 126131. [15] D.H. Ballard, A.P. Trace, S. Ali, T. Hodgdon, M.E. Zygmont, C.M. DeBenedectis, S.E. Smith, M.L. Richardson, M.J. Patel, S.J. Decker, Clinical applications of 3D printing: primer for radiologists, Acad. Radiol. 25 (2018) 5265. [16] W. Yang, W.S. Choi, Y.Y. Leung, J.P. Curtin, R. Du, C. Zhang, X. Chen, Y. Su, Three-dimensional printing of patient-specific surgical plates in head and neck reconstruction: a prospective pilot study, Oral. Oncol. 78 (2018) 3136. [17] Danial Khorsandi, Amir Fahimipour, 3D and 4D printing in dentistry and maxillofacial surgery: Printing techniques, materials, and applications, Acta.Biomaterialia. 122 (2021) 26-49.
[18] Anthony Tahayeri, MaryCatherine Morgan, Ana P Fugolin, Despoina Bompolaki, Avathamsa Athirasala, Carmem S Pfeifer, Jack L Ferracane, Luiz E Bertassoni, 3D printed versus conventionally cured provisional crown and bridge dental materials, Dent. Mater. 34 (2018) 192-200. [19] M. Dehurtevent, L. Robberecht, J.C. Hornez, A. Thuault, E. Deveaux, P. Béhin, Stereolithography: A new method for processing dental ceramics by additive computer-aided manufacturing, Dent. Mater. 33 (2017) 477485. [20] P. Makvandi, C.Esposito Corcione, F. Paladini, A.L. Gallo, F. Montagna, R. Jamaledin, M. Pollini, A. Maffezzoli, Antimicrobial modified hydroxyapatite composite dental bite by stereolithography, Polym. Adv. Technol. 29 (2018) 364371. [21] A.E. Ersoy, I. Turkyilmaz, O. Ozan, E.A. McGlumphy, Reliability of implant placement with stereolithographic surgical guides generated from computed tomography: Clinical data from 94 implants, J. Periodontol. 79 (2008) 13391345. [22] Y. Sun, H. Luebbers, J.O. Agbaje, S. Schepers, C. Politis, S. Van Slycke, L. Vrielinck, Accuracy of dental implant placement using CBCT-derived mucosa-supported stereolithographic template, Clin. Implant. Dent. Relat. Res. 17 (2015) 862870. [23] J.P. Kruth, B. Vandenbroucke, J. Van Vaerenbergh, I. Naert, Digital manufacturing of biocompatible metal frameworks for complex dental prostheses by means of SLS/SLM, Proceedings of the 2nd International Conference on Advanced Research in Virtual and Rapid Prototyping Leiria, Portugal (2005) 139-145. [24] J. Liu, B. Zhang, C. Yan, Y. Shi, The effect of processing parameters on characteristics of selective laser sintering dental glass-ceramic powder, Rapid Prototyp. J. 16 (2010) 138145. [25] L. Wu, H. Zhu, X. Gai, Wang, Y. Evaluation of the mechanical properties and porcelain bond strength of cobalt-chromium dental alloy fabricated by selective laser melting. J. Prosthet. Dent. 111 (2014) 5155. [26] N.K. Tolochko, V.V. Savich, T. Laoui, L. Froyen, G. Onofrio, E. Signorelli, V.I. Titov, Dental root implants produced by the combined selective laser sintering/melting of titanium powders, Proc. Inst. Mech. Eng. Part L J. Mater. Des. Appl. 216 (2002) 267270. [27] P.M. Gronet, G.A. Waskewicz, C. Richardson, Preformed acrylic cranial implants using fused deposition modeling: a clinical report, J. Prosthet. Dent.90 (2003) 429433.
[28] R.E. Rebong, K.T. Stewart, A. Utreja, A.A. Ghoneima, Accuracy of three-dimensional dental resin models created by fused deposition modeling, stereolithography, and Polyjet prototype technologies: a comparative study, Angle Orthod 88 (2018) 363369. [29] J. Brandt, H.C. Lauer, T. Peter, S. Brandt, Digital process for an implant-supported fixed dental prosthesis: a clinical report, J. Prosthet. Dent. 114 (2015) 469473. [30] V.N.V.M.R. Daule, Rapid prototyping and its application in dentistry, J. Dent. Allied Sci. 2 (2013) 5761. [31] A. Kessler, R. Hickel, M. Reymus, 3D printing in dentistry – state of the art, Oper. Dent. 45 (2020) 3040. [32] A. Hazeveld, J.J.R. Huddleston Slater, Y. Ren, Accuracy and reproducibility of dental replica models reconstructed by different rapid prototyping techniques, Am. J. Orthod. Dentofac. Orthop. 145 (2014) 108115. [33] H.N. Mai, K.B. Lee, D.H. Lee, Fit of interim crowns fabricated using photopolymer-jetting 3D printing, J. Prosthet. Dent. 118 (2017) 208215. [34] S. Prasad, N.A. Kader, G. Sujatha, T. Raj, S. Patil, 3D printing in dentistry, 2018. [35] P.W. Poeschl, N. Schmidt, G. Guevara-Rojas, R. Seemann, R. Ewers, H.T. Zipko, K. Schicho, Comparison of cone-beam and conventional multislice computed tomography for image-guided dental implant planning, Clin. Oral Investig. (2013) 317324. [36] A. Dawood, B.M. Marti, V. Sauret-Jackson, A. Darwood, 3D printing in dentistry, Br. Dent. J. (2015) 521529. [37] R. Jain, B.S. Supriya, K. Gupta, Recent trends of 3-D printing in dentistry-a review, Ann. Prosthodont. Rest. Dent. (2016) 101104. [38] L. Ferrage, G. Bertrand, P. Lenormand, D. Grossin, B. Ben-Nissan, A review of the additive manufacturing (3DP) of bio ceramics: alumina, zirconia (PSZ) and hydroxyapatite, J. Aust. Ceram. Soc. (2017) 1120. [39] DH Lee, HN Mai, JC Yang, TY Kwon, The effect of 4,4- bis(N,N-diethylamino) benzophenone on the degree of conversion in liquid photopolymer for dental 3D printing. J Adv Prosthodont (2015) 386-391. [40] B.E. Kelly, I. Bhattacharya, H. Heidari, M. Shusteff, C.M. Spadaccini, H.K. Taylor, Volumetric additive manufacturing via tomographic reconstruction, Science (2019) 10751079. [41] Guk Bae Kim, Sangwook Lee, Haekang Kim, Dong Hyun Yang, Young-Hak Kim, Yoon Soo Kyung, Choung-Soo Kim, Se Hoon Choi, Bum Joon Kim, Hojin Ha, Sun U. Kwon and Namkug Kim, Three-dimensional printing: basic principles and applications in medicine and radiology Korean J. Radiol. 17 (2016) 18297. [42] Azari A and Nikzad S, The evolution of rapid prototyping in dentistry: a review, Rapid Prototyp. J. 15 (2009) 21625. [43] A. Sakly, S. Kenzari, D. Bonina, S. Corbel, V. Fournée, A novel quasicrystal-resin composite for stereolithography, Mater. Des. 56 (2014) 280285. [44] R. Noorani, 3D printing: technology, applications, selection, CRC Press, 2017. [45] P. Aly and C. Mohsen, Comparison of the accuracy of three-dimensional printed casts, digital, and conventional casts: an in vitro study, Eur. J. Dent.14 (2020) 189.
[46] J. Bustillos, D. Montero-Zambrano, A. Loganathan, B. Boesl, A. Agarwal, Stereolithography-based 3D printed photosensitive polymer/boron nitride nanoplatelets composites, Polym. Compos. 40 (2019) 379388. [47] S.F.S.Shirazi et al, A review on powder-based additive manufacturing for tissue engineering: selective laser sintering and inkjet 3D printing Sci.Technol. Adv. Mater. 16 (2015) article. 033502
[48] O. Alageel, M.N. Abdallah, A. Alsheghri, J. Song, E. Caron, F. Tamimi, Removable partial denture alloys processed by laser-sintering technique, J. Biomed. Mater. Res. – Part B Appl. Biomater. 106 (2018) 11741185. [49] T.Koutsoukis, S.Zinelis, G.Eliades, K.Al-Wazzan, M.A.Rifaiy, Y.S.A.Jabbari, Selective laser melting technique of CoCr dental alloys: A review of structure and properties and comparative analysis with other available techniques. J. Prosthodont. 24 (2015), 303312. [50] M.V.Mergulhao, M.D.M.Neves, Characteristics of biometallic alloy to additive manufacturing using selective laser melting technology. J. Biomater.Nanobiotechnol. (2018) 8999.
[51] Kajima, Y.; Takaichi, A.; Nakamoto, T.; Kimura, T.; Yogo, Y.; Ashida, M.; Doi, H.; Nomura, N.; Tarahashi, H.; Hanawa, T.; et al. Fatigue strength of Co-Cr-Mo alloy clasps prepared by selective laser metling. J. Mech. Behav. Biomed. 59 (2016), 446458. [52] Y. Okazaki, A. Ishino, Microstructures and mechanical properties of laser-sintered commercially pure Ti and Ti-6Al-4V alloy for dental applications, Materials (Basel). 13 (2020) 609. [53] M. Figliuzzi, F. Mangano, C. Mangano, A novel root analogue dental implant using CT scan and CAD/CAM: selective laser melting technology, Int.J. Oral Maxillofac. Surg. 41 (2012) 858862.
[54] M.A. Alhnan, T.C. Okwuosa, M. Sadia, K.W. Wan, W. Ahmed, B. Arafat, Emergence of 3D printed dosage forms: opportunities and challenges, Pharm. Res. 33 (2016) 18171832. [55] Z. Chen, Z. Li, J. Li, C. Liu, C. Lao, Y. Fu, C. Liu, Y. Li, P. Wang, Y. He, 3D printing of ceramics: A review, J. Eur. Ceram. Soc. 39 (2019) 661687. [56] M. Wohlert, D. Bourell, Rapid prototyping of Mg/SiC composites by a combined SLS and pressureless infiltration process, Int. Solid Free. Fabr.Symp. (1996) 79-88.
[57] M.V. Mergulhao, C.E. Podesta M.D.M. Neves, Mechanical properties and microstructural characterization of cobalt-chromium (CoCr) obtained by casting and selective laser melting (SLM). Mater. Sci. Forum. 899 (2017) 534539. [58] C.Y.Yap, C.K.Chua, Z.L.Dong, Z,H.Liu, D.Q. Zhang, L.E.Loh and S.L.Sing, Review of selective laser melting: materials and applications Appl.Phys. Rev. 2 (2015) article. 041101.
[59] A. A. Bakr, R. Atik, S. Ozerinç, Effect of fused deposition modeling process parameters on the mechanical properties of recycled polyethylene terephthalate parts, Journal of Applied Polymer Science. 138 (2020) article. 49701. [60] P. Jain, A.M. Kuthe, Feasibility study of manufacturing using rapid prototyping: FDM approach, Procedia Eng. (2013) 411. [61] A. Arnesano, S. Kunjalukkal Padmnabhan, A. Notarangelo, F. Montagna, A. Licciulli, Fused deposition modeling shaping of glass infiltrated alumina for dental restoration, Ceram. Int. 46 (2020) 22062212. [62] U. K. uz Zaman, E. Boesch, A. Siadat, M. Rivette, and A. A. Baqai, Impact of fused deposition modeling (FDM) process parameters on strength of built parts using Taguchis design of experiments, The Int.J.Adv.Manuf. Tech. 101 (2018) 12151226. [63] Z. Lu, O.I. Ayeni, X. Yang, H.-Y. Park, Y.-G. Jung, J. Zhang, Microstructure and phase analysis of 3D-printed components using bronze metal filament, J. Mater. Eng. Perform. (2020) 17. [64] S. C. Ligon, R. Liska, J. Stampfl, M. Gurr, and R. Mulhaupt, Polymers for 3D printing and customized additive manufacturing, Chem. Rev. 117(15) (2017) 1021210290. [65] E. Cuan-Urquizo, E. Barocio, V. Tejada-Ortigoza, R.B. Pipes, C.A. Rodriguez, A. Roman-Flores, Characterization of the mechanical properties of FFF structures and materials: A review on the experimental, computational and theoretical approaches, Materials (Basel) 12 (2019) 895. [66] A. Barazanchi, K. C. Li, B. Al-Amleh, K. Lyons, and J. N. Waddell, Additive technology: update on current materials and applications in dentistry, J.Prosthodontics. 26(2) (2017) 156163. [67] Z. Yefang, D. W. Hutmacher, S. L. Varawan, and L. T. Meng, Comparison of human alveolar osteoblasts cultured on polymer-ceramic composite scaffolds and tissue culture plates, Int.J.Oral.Maxillofacial Surg.36 (2) (2007)137145. [68] J.A.Lewis, Direct ink writing of 3D functional materials, Adv. Funct. Mater. 16 (2006) 21932204. [69] A. Mostafaei, E.L. Stevens, J.J. Ference, D.E. Schmidt, M. Chmielus, Binder jet printing of partial denture metal, Mater. Sci. Technol. (2017) 289 291. [70] Liska R, Schuster M, Infuhr R, et al: Photopolymers for rapid prototyping. J Coat. Tech.Res.(2007) 505510 [71] H. Lipson, M. Kurman, Fabricated: The new world of 3D printing, John Wiley and Sons, 2013. [72] R. Pandey, Photopolymers in 3D printing applications, Photopolymers 3D Printing Applications, Degree Thesis. (2014). [73] Y. Lu, G. Mapili, G. Suhali, S. Chen, K. Roy, A digital micro-mirror device based system for the microfabrication of complex, spatially patterned tissue engineering scaffolds, J. Biomed. Mater. Res. Part A. 77A (2006) 396405. [74] Y. He, Y. Wu, J. Fu, Q. Gao, J. Qiu, Developments of 3D Printing Microfluidics and Applications in Chemistry and Biology: a Review, Electro analysis 28 (2016) 16581678. [75] H.B. Yamak, Emulsion polymerization: effects of polymerization variables on the properties of vinyl acetate based emulsion polymers, Polym. Sci., Intech Open, 2013. [76] A. Echte, Rubber-toughened styrene polymers: A review, ACS Publications, 1989. [77] C. Yu, J. Schimelman, P. Wang, K.L. Miller, X. Ma, S. You, J. Guan, B. Sun, W. Zhu, S. Chen, Photopolymerizable biomaterials and light-based 3D printing strategies for biomedical applications, Chem. Rev. (2020). [78] J. Jockusch, M. Özcan, Additive manufacturing of dental polymers: an overview on processes, materials and applications, Dent. Mater. J. (2020) 345- 354. [79] N. Sathishkumar, A.S.M. Udayakumar, B. Vincent, V.A. Kumar, Study and analysis of 3D printed FDM components by non-destructive testing techniques, Int. J. Res. Rev. 7 (2020) 217-222. [80] Z.A.A. Halim, M.A.M. Yajid, M.H. Idris, H. Hamdan, Dispersion of polymeric coated-silica aerogel particmakvanles in unsaturated polyester composites: effects on thermal-mechanical properties, J. Dispers. Sci. Technol. 39 (2018) 10931101. [81] S.D. Jensen, Thermoplastic/thermoset dental restorative prosthetics, U.S. Patent Application No 12/396,438, (2009). [82] M. imková, A. Tichý, M. Duková, P. Bradna, Dental composites – a lowdose source of bisphenol A? Physiol. Res. 69 (2020) S295S304. [83] S.A. Park, H.-J. Lee, K.-S. Kim, S.J. Lee, J.-T. Lee, S.-Y. Kim, N.-H. Chang, S.-Y. Park, In vivo evaluation of 3D-printed poly caprolactone scaffold implantation combined with -TCP powder for alveolar bone augmentation in a beagle defect model, Materials (Basel) 11 (2018) 238. [84] E.A.M. Hassan, S.E. Elarabi, Y. Wei, M. Yu, Biodegradable poly (lactic acid)/poly (butylene succinate) fibers with high elongation for health care products, Text. Res. J. 88 (2018) 17351744. [85] Su TS, Sun J. Comparison of marginal and internal fit of 3-unit ceramic fixed dental prostheses made with either a conventional or digital impression.J Prosthet Dent. 116 (3) (2016)3627.
[86] Neves FD, Prado CJ, Prudente MS, Carneiro TA, Zancopé K, Davi LR, et al. Micro computed tomography evaluation of marginal fit of lithium disilicate crowns fabricated by using chairside CAd/CAM systems or the heat-pressing technique. J Prosthet Dent.112 (5) (2014)11341140. [87] G. Struzikiewicz, W. Zebala, A. Matras, M. Machno, . Slusarczyk, S. Hichert, F. Laufer, Turning research of additive laser molten stainless steel 316L obtained by 3D printing, Materials (Basel) 12 (2019) 182. [88] M.Z. Ibrahim, A.A.D. Sarhan, T.Y. Kuo, F. Yusof, M. Hamdi, Characterization and hardness enhancement of amorphous Fe-based metallic glass laser cladded on nickel-free stainless steel for biomedical implant application, Mater. Chem. Phys. 235 (2019) article. 121745. [89] G. Singh, A. Saini, Developments in Metallic Biomaterials and Surface Coatings for Various Biomedical Applications, Adv. Mater. Process Confer. (2020) 197206. [90] M. Talha, C.K. Behera, O.P. Sinha, A review on nickel-free nitrogen containing austenitic stainless steels for biomedical applications, Mater. Sci.Eng. C. 33 (2013) 35633575.
[91] X. Chen, K. Shah, S. Dong, L. Peterson, E.C. La Plante, G. Sant, Elucidating the corrosion-related degradation mechanisms of a Ti-6Al-4V dental implant, Dent. Mater. 36 (2020) 431441. [92] J.W Nicholson, Titanium alloys for dental implants: a review, Prosthesis 2 (2020) 100116. [93] C. Veiga, J.P. Davim, A.J.R. Loureiro, Properties and applications of titanium alloys: a brief review, Rev. Adv. Mater. Sci. 32 (2012) 133148. [94] M. Lowther, S. Louth, A. Davey, A. Hussain, P. Ginestra, L. Carter, N. Eisenstein, L. Grover, S. Cox, Clinical, industrial, and research perspectives on powder bed fusion additively manufactured metal implants, Add. Manuf. 28 (2019) 565584. [95] J. Zuchuat, A. Cura, A. Manzano, O. Decco, CoCrMo alloy as biomaterial for bone reconstruction in oral and maxillofacial surgery: a scoping review, J. Oral Res. 9 (2020) 336349. [96] N.H. Mat-Baharin, M. Razali, S. Mohd-Said, J. Syarif, A. Muchtar, Influence of alloying elements on cellular response and in-vitro corrosion behavior of titanium-molybdenum-chromium alloys for implant materials, J. Prosthet. Dent. (2020) 490-497. [97] J. Han, J. Zhao, Z Shen, Zirconia ceramics in metal-free implant dentistry, Adv. Appl. Ceram. 116 (3) (2017) 138150. [98] G.P. Jayaswal, S.P. Dange, A.N. Khalikar, Bioceramic in dental implants: A review, J. Indian Prosthodont. Soc. 10 (2010) 812. [99] A. Shenoy, N. Shenoy, Dental ceramics: an update, J.con.dent, 13 (4) (2010) 195-203. [100] T.A. Khabas, L.V Maletina, K.S. Kamyshnaya, Influence of nanopowders and pore-forming additives on sintering of alumma-zircorna ceramics, IOP Conference Series: Materials Science and Engineering, IOP Publishing (2014). [101] Y. Zhang, Y. Yang, W. Li, Y. Ren, Effect of Al2O3 addition on the flexural strength and light-transmission properties of bone china, Int. J. Appl.Ceram. Technol. 12 (2015) 875884.
[102] D. M. Simoneti, T. Pereira-Cenci, and M. B. F. Dos Santos, Comparison of material properties and biofilm formation in interim single crowns obtained by 3D printing and conventional methods, J. Prosthet. Dent. 127(1) (2022) 168-172. [103] J. Y. Park, D. Jeong, J. J. Lee, S. Y. Bae, J. H. Kim, and W. C. Kim, In vitro assessment of the marginal and internal fits of interim implant restorations fabricated with different method, J. Prosthet. Dent. 116(4) (2016) 536542. [104] W. Wang, H. Yu, Y. Liu, X. Jiang, and B. Gao, Trueness analysis of zirconia crowns fabricated with 3-dimensional printing, The J.Pros. Dent 121(2) (2019) 285-291. [105] M. A. De Bruyne, R. J. De Moor, and F. M. Raes, Necrosis of the gingiva caused by calcium hydroxide: a case report, Int. Endod. J 33(1) (2000) 67 71. [106] I. Tregerman, W. Renne, A. Kelland, and D. Wilson, Evaluation of removable partial denture frameworks fabricated using 3 different techniques, J. Prosthet. Dent. 122(4) (2019) 390-395. [107] I. E. Harb, E. A. Abdel-Khalek, and S. A. Hegazy, CAD/- CAM constructed poly(etheretherketone) (PEEK) framework of Kennedy Class I removable partial denture: a clinical report, J. Prosthet. Dent. 128(2) (2019) 595-598. [108] M. Salmi, K. S. Paloheimo, J. Tuomi, T. Ingman, and A. Makitie, A digital process for additive manufacturing of occlusal splints: a clinical pilot study, J.Royal Soc.Int.(10) (2013) article no.20130203. [109] W. Piedra Cascon and M. Revilla-Leon, Digital workflow for the design and additively manufacture of a splinted framework and custom tray for the impression of multiple implants: a dental technique, J. Prosthet. Dent. 120(6) (2018) 805-811. [110] S. J. Smith, J. F. McCord, and T. V. Macfarlane, Factors that affect the adhesion of two irreversible hydrocolloid materials to two custom tray materials, J. Prosthet. Dent. 88(4) (2002) 423-430. [111] J. Burns, Accuracy of open tray implant impressions: an in vitro comparison of stock versus custom trays, J. Prosthet. Dent. 89(3) (2003) 250-255. [112] D. Anssari Moin, W. Derksen, H. Waars, B. Hassan, and D. Wismeijer, Computer-assisted template-guided custom designed 3D-printed implantplacement with custom designed 3D-printed surgical tooling: An In-vitro proof of a novel concept, Clin.Oral Imp. Res. 28 (5) (2017) 582585.