
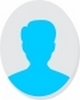
- Open Access
- Authors : Mario Peres , Edumar Ramos Cabral Coelho
- Paper ID : IJERTV10IS110145
- Volume & Issue : Volume 10, Issue 11 (November 2021)
- Published (First Online): 01-12-2021
- ISSN (Online) : 2278-0181
- Publisher Name : IJERT
- License:
This work is licensed under a Creative Commons Attribution 4.0 International License
The Influence of POU Pot-Type Ceramic Candle Filters, with and Without Activated Carbon, in The Abundance of Bacteria in Treated Water
Mario Peres, Edumar Ramos Cabral Coelho Department of Environmental Engineering Federal University of EspÃrito Santo (UFES) Vitória, Brazil
Abstract: In this research the potential growth of fecal indicator bacteria (FIB) and heterotrophic bacteria in water stored for a 24-hour period, following POU treatment using pot- type ceramic candle filters (traditional or with activated carbon) to treat contaminated and chlorinated water sources. Independently of the source, thermotolerant coliform and enterococcus were rarely detected in filtered water, showing that tested POU devices were effective against FIB. In contrast, heterotrophic bacteria count increased during storage in all scenarios investigated, which indicated that some species from this group were able to grow even when chlorinated water was filtered, although higher counts were observed when contaminated water was treated. Moreover, this increase was about 2 log10 higher for ceramic candle filters with activated carbon compared to traditional ones. Although FIB were mostly absent in treated water, the outcomes of this research suggests that ceramic candle filters may be suitable to treat contaminated water but not as appropriate to treat well-disinfected water sources, presenting adequate free chlorine levels, as it can enhance the presence of heterotrophic bacteria, and consequently opportunistic pathogens, especially if activated carbon is used as a filling of ceramic candle filters.
Keywords: Activated Carbon, Bacterial Growth, Ceramic Candle Filter, HWTS, POU Water Treatment.
-
INTRODUCTION
Concerns about drinking water quality has increased the number of consumers that adopt point-of-use (POU) drinking water treatment as a measure to reduce taste and odor issues, normally caused by undesirable chemicals, as well as to remove waterborne microbes where public water treatment is inadequate or inexistent [1]. POU water treatment can include various methods such as the application of heat or UV (e.g., boiling, solar radiation, UV lamps), chemical disinfection (e.g., chlorine), and physical removal by filtration (e.g., slow sand filtration, ceramic filters). Most of these methods have been reported to improve physicochemical and microbial water quality of drinking water [2], [3].
Ceramic candle filters have gained widespread use around the world as a low cost and easy to operate POU water treatment capable of producing safe drinking water [4]. In Brazil, although the introduction of high-tech water purifiers in the last decades contributed to a decline from 57.2% (1990) to 51.3%
(2004) in the proportion of private permanent residences that possessed one of these filters [5], they are still believed to be the preferred method among Brazilians as this proportion was maintained stable from 2004 to 2015, varying in a range from 50.27 to 53.40% [6].
The most common types of ceramic candle filters are pot- type and faucet-type filters (Figure 1). The pot-type ceramic candle filter systems consist of a porous ceramic candle filter attached to an upper reservoir that sits on top of a lower storage reservoir. Source water is poured into the top reservoir, passes through the ceramic candle filter due to gravitational forces and drips slowly into the lower reservoir, in which filtered water is stored [7]. The storage reservoir is normally fitted with a tap and the top one with a lid. In the case of faucet-type filters, a porous ceramic candle filter is fitted in a sealed case, in which water flow through, and that can be installed to a standard faucet [8].
-
Pot-type and faucet-type ceramic candle filter systems.
Source: Stefanis ceramic website.
Ceramic candle filters can be made of various mineral media, including clays and other fine particles. The media are blended, shaped, dried and fired at different temperatures to achieve required pore sizes and filtration properties [9]. Similarly to other filtration methods, the traditional ceramic
Identify applicable sponsor/s here. If no sponsors, delete this text box
candle filters remove particles larger than the size of the pores in the filter material [10]. Moreover, a thin biofilm layer developed on the ceramic candle filters surface has also been observed to increase particle removal [11]. However, as usage time increases, this layer can become very thick causing a decrease in the flow rate. At this point the candles should be replaced or adequately cleaned to restore its functionality [7], [9]. To prevent biofilm formation within the candle itself, some ceramic candle filter producers developed new candles with colloidal silver impregnated on their surface or mixed with the media before firing [9].
One of the main advantages of POU ceramic candle filters is that it does not require energy supply and, therefore, they are an attractive alternative to remote rural communities. In contrast, the major risks to the success of all forms of ceramic filtration are hairline cracks and cross-contamination. Even the presence of only slightly cracked filter can make them unsuitable for reduction of particles and microbes from water [9]. Moreover, even when the filtration process is effective, the quality of stored water can be affected by various other factors, including condition and duration of storage, reservoir characteristics and water source [12].
Typically, well designed ceramic filters present satisfactory removal rates for suspended solids and most bacteria, protozoa and microbial cysts but is ineffective against viruses since their size is too small to be retained by the filter. Previous studies have shown a turbidity reduction ranging from 80 to 99% [13], [14], [15]. With respect to microbial contaminants, reference
[16] observed a reduction of 4.3 and 4.6 log10 for Cryptosporidium parvum and Giardia lamblia, respectively. Moreover, removal rates varying from 2 to 7 log10 for total coliform and E. coli have been observed in previous research [17], [18], [19].On the other hand, ceramic candle filters do not remove chemicals itself. In order to address this, some ceramic candle filters incorporate activated carbon inside the candle, making them capable of absorbing various chemical compounds that presents high risk to human health. According to reference [20], activated carbon can remove some pesticides, herbicides, metallic contaminants, disinfection by-products (DBPs), organic compounds as well as free chlorine and other compounds that causes taste and odor in drinking water.
Although chemical removal was significantly enhanced with the incorporation of activated carbon, the WHO has long- nurtured that POU water treatment based on filters with activated carbon is not appropriate to use in poorly disinfected waters as it can support the excessive growth of bacteria, and consequently, increase the risk of enhancing pathogen concentration [21]. Moreover, the reference [22] in a recent study using molecular techniques, observed that the use of activated carbon block filters promoted significant alteration not only in the bacterial abundance but also in the bacterial diversity of filtered water.
Most drinking water standards require the use of FIB to indicate faecal contamination and heterotrophic bacteria as a surrogate to process control [23]. The Brazilian drinking water regulation requires the absence of E. coli and total coliform in 100 mL of treated water for human consumption. Moreover, in
order to guarantee the integrity of the distribution system,it also requires the analysis of heterotrophic bacteria, which may indicate some irregularity in the distribution system if its concentration reach levels above 500 CFU/mL [24].
It is relevant to mention that most of recent researches using POU ceramic candle filters focused on the faucet-type. Although some studies considering the pot-type gravitational ceramic candle filters have been found in the literature, most of them were performed long time ago. Furthermore, most of these studies were focused on the efficacy of these systems in removing microbes when applied to treat various water sources but studies to investigate the effect of these type of filters on bacterial growth were rarely found in the literature, especially when chlorinated water source was considered.
When pot-type ceramic candle filters are employed, filtered water is stored in the lower reservoir, normally for a period no longer than 24 hours as these systems are commonly refilled every single day. As ceramic candle filters with activated carbon is expected to produce water without remaining free- chlorine, it is believed that bacteria growth in treated water is very likely to happen during storage. This research aimed to investigate the potential growth of thermotolerant coliform, enterococcus and heterotrophic bacteria in drinking water stored for a period of 24 hours, following POU water treatment using pot-type ceramic candle filters, with and without an activated carbon filling, and considering the use of contaminated and chlorinated water sources.
-
-
MATERIAL AND METHODS
-
Experimental design
An experimental study was developed to assess the potential growth of FIB and heterotrophic bacteria in stored water, following a POU drinking water treatment based on pot-type ceramic candle filters fitted with traditional ceramic candles or ceramic candle filters with activated carbon from a very popular brand (not displayed due to ethical reasons). It is important to mention that all tested ceramic filters did not present any trace of silver, which is commonly impregnated in these types of filters nowadays. According to the candles producer, the ceramic candle filters for gravitational systems used in this research presented pores size varying from 5 to 15 m. Moreover, contaminated water and well-disinfected chlorinated water sources were used, resulting in four tested scenarios.
The experiment and all the analyses were performed at the Laboratory of Sanitation (LABSAN) from May to July 2020. Figure 2 presents the schematics of the experimental design, including the two treatments tested, which varied according to the presence of activated carbon (coconut shell) inside the candle (TAC) or not (TT), and considered two water sources (contaminated (A1) and chlorinated (CT > 30) (A2)). All POU water treatments were tested using two replicates, and therefore, eight of these systems were mounted side by side on a bench in the laboratory.
It is important to bear in mind that the experiments were continuously running during the entire study period, with exception to the weekends, and therefore, fresh contaminated and chlorinated water were daily prepared and added to the top reservoir of ceramic candle filters every weekday. However, as
presented later in this section, water quality monitoring was performed weekly. It is also relevant to mention that the ceramic candle filters were not cleaned during the entire experiment period.
-
Preparation of contaminated water
As previously mentioned, two affluents were used in this research (Figure 2): contaminated (A1) and chlorinated (A2). Affluent A1 was produced by adding 25 L of tap water, dechlorinated with sodium thiosulfate (1.8%), and 2.5 mL of sewage into a clean gallon (40 L). This mixture was well homogenized before use. This proportion of sewage (1:10000) was chosen to simulate potentially contaminated waters sources, presenting low turbidity and organic matter content but moderate microbial concentration such as harvested rainwater, groundwater, etc. Fresh sewage was collected three times a week at a wastewater treatment plant located in Vila Velha, ES.
-
Experimental schematics.
Legend: = sampling points; A = affluent; C = control; T = treatment (treatment, followed by replicate identification).
To prepare A2, 25 L of tap water was added into a clean gallon (40 L), but instead of dechlorinating it, bleach (OBA, 2.0-2.5%) was dosed when the level of free chlorine in tap water was lower than 1.5 mg/L. This was performed because preliminary tests indicated that to guarantee the maintenance of a minimum level of one mg/L of free chlorine in A2 (after 30 min of contact time), considering the conditions of this study, tap water should present at least 1.5 mg/L of free chlorine. Finally, 2.5 mL of sewage was added to the gallon and mixed well.
-
-
Sample collection
Although the ceramic candle filters were filled every weekday to better represent the way that householders normally use them, physicochemical and microbiological analyses were monitored weekly for a period of 10 weeks. Samples of contaminated and chlorinated water were collected from the midpoint of the 40 L gallon, at 10-15 cm below the water surface, following vigorous homogenization. Moreover, following a period of 24 hours, samples of treated water were collected from the tap of the storage reservoir of all systems. In total, 10 samples were collected every week and analyzed for
selected physicochemical and microbiological parameters presented later in this section.
It is important to mention that all samples were collected in sterile polypropylene containers and sodium thiosulfate (1.8%) was used to neutralize chlorine when chlorinated water was sampled. Moreover, the tap of all storage reservoirs was carefully disinfected with alcohol 70% and the first 200 mL of treated water from each system was discarded before sampling. All sampling points are shown within circles in Fig. 2.
-
Experimental control
In this study, three controls were selected to investigate the potential bacterial growth in contaminated water (C1) and chlorinated water, following dechlorination (C2) or not (C3), following a period of 24 hours. Sterile containers were filled with contaminated water (C1) or chlorinated water (C2 and C3) and, immediately, stored in the dark for 24 hours. Sodium thiosulfate (1.8%) was added to C2 container to neutralize free chlorine. Microbiological analyses were performed in all controls and C3 was also monitored for free chlorine.
-
Microbiological analyses
All samples and controls were analyzed for microbiological parameters according to the Standard Method for the Examination of Water and Wastewater [25]. The membrane filtration technique using 0.45 m pore size membranes (UNIFIL, Brazil) was used for enumeration of thermotolerant coliform, enterococcus and heterotrophic bacteria. Samples were filtered on membranes that were placed onto m-FC (NEOGEN – Lansing, USA), m-Enterococcus (NEOGEN – Lansing, USA) or m-HPC (NEOGEN – Lansing, USA) agar for thermotolerant coliform, enterococcus and heterotrophic bacteria, respectively. The m-FC plates were incubated at 44.5
°C for 24 hours, whereas the m-Enterococcus and m-HPC
plates were incubated at 35 °C for 48 hours.
The volume of sample for microbiological analysis varied from 1 to 100 mL according to the water quality. Moreover, samples with higher concentration of microorganisms were diluted to a maximum of 100.000 folds whenever it was necessary. All dilutions were performed in sterile capped tubes using phosphate buffer water (PBS) as diluent. Two blanks to guarantee PBS integrity were carried out at the beginning and at the end of each batch of analyses.
-
Physicochemical analyses
Physicochemical analyses were performed according to the Standard Method for the Examination of Water and Wastewater [25]. All water samples were tested for turbidity (2130) uing a portable turbidimeter (HACH 2100P, Loveland, USA), pH (4500-H+) with a pHmeter DIGIMED (DM-22, Brazil) and temperature (2550) at the time of collection, excepted A2, which was analyzed only for pH and free chlorine (4500-Cl/G) using a free chlorine checker (HANNA HI701, Smithfield, USA). Moreover, samples from A2 and C3 as well as from TT and TAC, treating chlorinated water source (A2), were also tested for free chlorine.
-
Statistical Methods
Descriptive and inferential statistical analysis were used to evaluate the outcomes of this research. As the number of
observations (n = 10) was not large enough, the normality of data could not be assumed. Therefore, all the results are presented in terms of their median, followed by the interquartile range between brackets. Moreover, non-parametric tests were applied to evaluate the dataset. Wilcox test and Kruskal Wallis combined with Dunns test were used to investigate differences in the median concentration of evaluated parameters among treatments, controls and affluents. In order to search for correlations in the dataset, the Spearman rank correlation was adopted. Boxplots and line plots were also used to illustrate the outcomes of this research. All analyses were performed using R Version 4.0.0 [26] and RStudio Version 1.3.1056 [27] as well as some packages, including readxl, dplyr, dunn.test, FSA, ggpubr and ggplot2.
-
-
RESULTS
-
Affluent characterization
Temperature and turbidity of chlorinated affluent (A2) were assumed to be similar to contaminated affluent (A1). Affluent A2 presented median pH of 7.4 (7.2 – 7.4) and median level of free chlorine of 1.80 (1.53 – 1.84) mg/L, which decreased to
1.54 (1.25 – 1.59) mg/L after 30 min of contact time, but never presented concentration below 1.19 mg/L. This corresponds to a median CT of approximately 45 min.mg/L, which was very effective against all bacteria investigated in this study. No bacteria growth was observed in any sample from A2 throughout the entire experiment period.
Affluent A1 presented median temperature of 26.0 (25.0 – 26.8) °C and median pH of 7.3 (7.2 – 7.4), which was very similar to the values observed in A2. As planned, the affluent presented low median turbidity (1.02 (0.90 – 1.32) NTU), and relatively high median concentration of thermotolerant coliform (2.7 (2.6 – 2.7) log10 CFU/100 mL) and enterococcus (2.5 (2.5 – 2.6) log10 CFU/100 mL). Moreover, the median concentration of heterotrophic bacteria was 4.7 (4.6 – 4.7) log10 CFU/100 mL, approximately two folds higher than FIB.
-
Experimental controls
No bacteria were observed in 100 mL of sample from C3, which consisted of A2 stored in the dark for 24 hours. The median chlorine concentration in C3 following the 24-hour period was 1.20 (0.99 – 1.31) mg/L. Considering C2, thermotolerant coliform were observed in only one sample at a very low concentration (0.2 log10 CFU/100 mL), whereas enterococcus was never detected in 100 mL of sample. On the other hand, heterotrophic bacteria were always observed in C2, presenting a median concentration of 2.4 (2.3 – 2.6) log10 CFU/100 mL, which was significantly higher compared to A2 (p-value = 1.64E-04).
Compared to A1, control C1 (A1 stored in the dark for 24 hours), presented significantly higher median concentration of thermotolerant coliform (4.1 (4.0 – 4.2) log10 CFU/100 mL, p- value = 1.58E-04) and heterotrophic bacteria (6.7 (6.5 – 6.8) log10 CFU/100 mL, p-value = 1.63E- 04). However, the median count for enterococcus (2.3 (2.3 – 2.4) log10 CFU/100 mL) was observed to decrease slightly following the storage period.
-
Evaluation of pot-type ceramic candle filters
The most relevant results of POU water treatment using pot- type ceramic candle filters, considering contaminated (A1) and chlorinated (A2) water sources are graphically presented in Figure 3. Treatment of A2 using these systems fitted with traditional ceramic candle filter (TT) produced water with a median concentration of 0.30 (0.22 – 0.33) mg/L of free chlorine. It is important to remind that A2 was not dechlorinated before being added to the top reservoir, which resulted in the remaining concentration of free chlorine, following the 24- hour period. On the other hand, as expected, samples from treated water using ceramic candle filters filled with activated carbon (TAC) did not present any level of free chlorine during the testing period. The overall median turbidity reduction, considering all scenarios, was approximately 67%. This resulted in water with median turbidity lower than 0.5 NTU for both ceramic candle filters and both affluents, with no sample presenting values higher than 0.7 NTU.
-
Boxplots presenting the results of POU drinking water treatment using pot-type ceramic candle filters with 2 types of candle filters to treat chlorinated and contaminated water.
Legend: A = A1 for contaminated affluent or A2 for chlorinated affluent, TT = treatment with traditional ceramic candle filters; TAC = treatment with ceramic candle filters filled with activated carbon; CFU = colony forming units; NTU = nephelometric turbidity units.
The microbial quality of produced water presented a higher variation depending on the affluent and type of ceramic candle filters used as well as on the microorganism evaluated. Enterococcus and thermotolerant coliform were not observed in
100 mL of sample from TT and TAC treating A2 throughout the entire experimental period. Treatment TAC, even when applied to treat A1, presented satisfactory removal rates for enterococcus and thermotolerant coliform, which were not found in 100 mL of samples either. However, when TT was used to treat A1, thermotolerant coliform and enterococcus were observed in one and three out of ten observations, but in low concentration (< 0.4 and < 0.1 log10 CFU/100 mL, respectively).
In contrast, independently of the affluent and the treatment applied, treated water always presented higher counts of heterotrophic bacteria compared to the affluent. Higher counts were observed when A1 was used, resulting in a median concentration of 5.3 (5.2 – 5.3) log10 CFU/100 mL in water treated using TT, which was significantly lower than the median concentration observed in water produced by TAC (7.0 (6.3 – 7.1) log10 CFU/100 mL) (p-value = 8.54E-05). Similarly, when A2 was tested, the median count of heterotrophic bacteria observed in treated water by TT (0.6 (0.4 – 0.7) log10 CFU/100 mL) was significantly lower compared to the median count using TAC (3.5 (2.5 – 3.7) log10 CFU/100 mL) (p-value = 1.53E- 04).
In order to compare the growth of heterotrophic bacteria, the absolute value for the difference between the concentration of heterotrophic bacteria in treated water and affluent was calculated. The median increase in the concentration of heterotrophic bacteria observed in water produced by TT was 0.55 (0.50 – 0.60) log10 and 0.55 (0.40 – 0.70) log10 when
treating A1 and A2, respectively. On the other hand, when ceramic candle filters with activated carbon (TAC) were used, the median increment was 2.03 (1.52 – 2.37) log10 when treating A1 and 3.45 (2.55 – 3.70) log10 considering the treatment of A2.
The median increase in the concentration of heterotrophic bacteria in water produced by TT was lower compared to TAC in all experiment repetitions, independently of the affluent used. This increase was significantly lower in water produced by TT treating A2 compared to TAC treating A1 (p-value = 3.25E-04) and TAC treating A2 (p-value = 1.71E-04). Furthermore, the median increase in the count of heterotrophic bacteria was also observed to be significantly lower in TT treating A1 compared to TAC treating A1 (p-value = 1.76E-04) and TAC treating A2 (p-value = 1.67E-04).
-
-
The influence of usage time on pH and heterotrophic bacteria count in water treated by pot-type ceramic candle filters
Most of investigated parameters did not present any correlation with usage time, with exeption to pH and heterotrophic bacteria counts, which presented strong correlations, considering the 10-week experimental period. The pH level of treated water, independently of the treatment applied, was observed to reduce more than one unit in this period (Figure 4). A significant strong negative correlation of approximately 0.86 (p-value = 1.29E-03) was observed between usage time and pH of water produced by TT, whereas a slightly stronger correlation (rho = – 0.98, p-value = 1.50E-
06) was observed between usage time and pH of treated water using TAC.
Considering the difference from the first to the last week of the experimental period, an increase of nearly 2 log10 in the concentration of heterotrophic bacteria was observed in treated water using TAC. On the other hand, TT was able to produce water with much lower variation and similar concentration of heterotrophic bacteria throughout the entire experiment (Figure 5). A significant strong positive correlation (rho = 0.92, p-value
= 1.33E- 04) was observed between usage time and the count of heterotrophic bacteria in water produced by TAC.
-
Temporal variation of pH in the affluents and treated waters, considering the use of ceramic candle filters with and without activated carbon, during 10 weeks of operation.
-
Temporal variation of heterotrophic bacteria counts in the controls (C1
and C2), affluents and treated waters, considering the use of ceramic candle filters with and without activated carbon, during 10 weeks of operation.
It is also interesting to notice that the median concentration of heterotrophic bacteria in water produce by traditional ceramic candle filters (TT) was lower compared to controls. This resulted in a significant difference in the median concentration of heterotrophic bacteria observed between water produced using TT to treat A2 (0.6 (0.4 – 0.7) log10 CFU/100 mL) compared to C2 (2.4 (2.3 – 2.6) log10 CFU/100 mL) (p- value = 1.76E-04) and between water produced by TT treating A1 (5.3 (5.2 – 5.3) log10 CFU/100 mL) compared to C1 (6.7 (6.5
– 6.8) log10 CFU/100 mL) (p-value = 1.63E-04). However, the higher difference in the count of heterotrophic bacteria observed between treated water using TT applied to treat A2 and
C2 was very likely caused by the use of dechlorinated A2 to fill up C2 containers and chlorinated A2 to fill the top reservoir of ceramic candle filters.
In contrast to that, the use of ceramic candle filters filled with activated carbon (TAC) produced water with higher counts of heterotrophic bacteria compared to controls after six and three weeks of operation, considering the treatment of A1 and A2, respectively. However, a significant difference in the median concentration of heterotrophic bacteria was only observed between water produced by TAC treating A2 (3.5 (2.5
– 3.7) log10 CFU/100 mL) and C2 (2.4 (2.3 – 2.6) log10 CFU/100
mL) (p-value = 0.048).
-
-
DISCUSSION
Independently of the water source (A1 or A2), treatments TT and TAC produced water with relatively high pH, ranging from approximately 9.0 to 10.0, during the first weeks of the experimental period, corresponding to an increase of 2 to 3 units. However, at the end of this study, the pH of treated water decreased to approximately 8.0. Although such high values have not been detected in previous studies, similar increase in the pH level have been observed by [28], [29]. Reference [29] observed pH increase of about 2 to 4 units when using traditional ceramic candle filters to treat mineral water and water from the public supply in Recife, Brazil.
Turbidity was significantly reduced in all tested scenarios, although slightly better results were achieved using traditional ceramic candle filters (TT), especially when applied to treat chlorinated water (A2). The observed median reduction of turbidity, considering all scenarios, was 67% (59 71%), which was lower than the reduction rates of 95% and 98-99%, observed by references [13] and [14], respectively.
The reason for that may lie on the fact that the affluent used in this study presented much lower turbidity (approximately 1.0 NTU) compared to the water sources used by [13] (89 NTU) and [14] (30 NTU). Furthermore, although pores size of ceramic candle filters used in the mentioned studies were not displayed, it could be also an important factor affecting the removal of suspended particles, consequently the reduction of turbidity.
Based on an initial concentration of FIB (varying from 2.3 to 2.9 log10 CFU/100 mL) and heterotrophic bacteria (varying from 4.4 to 5.4 log10 CFU/100 mL) in contaminated water, the chlorination process (median CT = 45 min.mg/L) was very effective against all investigated bacteria, resulting in an affluent (A2) free of thermotolerant coliform, enterococcus and heterotrophic bacteria in 100 mL of sample. Following a 24- hour storage period, enterococcus were not recovered from any sample of chlorinated-dechlorinated (C2) and chlorinated (C3) controls, however thermotolerant coliform were once detected in C2. According to reference [30], the chlorination disinfection process is very effective against FIB and most enteric pathogens.
On the other hand, a growth of about 2 to 3 log10 of heterotrophic bacteria were observed in C2. Previous research has shown that some species of heterotrophic bacteria were more resistant to chlorine than FIB [31], and were able to form biofilm and grow within water distribution systems [32]. In this
study, even in the presence of relatively low levels of free chlorine ranging from 0.16 to 0.34 mg/L (TT treating A2), heterotrophic bacteria were able to grow at a rate ranging from
0.2 to 1.2 log10. However, it is important to notice that the observed growth may have been affected by the presence of a less effective form of free chlorine (hypochlorite ion), formed at high pH levels (condition of this study), compared to hypochlorous acid, which is formed at lower pH levels and is well-known to be more effective against microbes [33].
The use of chlorinated or contaminated affluents did not seem to affect the microbial quality of filtered water with respect to thermotolerant coliform and enterococcus. Satisfactory removal rates for thermotolerant coliform and enterococcus have also been observed in previous studies [34], [35]. In contrast, heterotrophic bacteria were detected in all samples of treated water, independently of the affluent used (contaminated or chlorinated). Higher resistant to chlorine- based products compared to FIB may explain the detection of relatively high counts of heterotrophic bacteria in produced water when A2 was used, although the treatment of A1 produced water presenting much higher concentration.
Similarly, the type of ceramic candle filter (traditional or with activated carbon) did not influence the reduction of thermotolerant coliform and enterococcus significantly. However, higher concentration of heterotrophic bacteria was observed in treated water using ceramic candle filters with activated carbon (TAC). The use of activated carbon to treat water has been indeed observed to facilitate and support the growth of bacteria [21], [22]. Furthermore, it is also interesting to note that the concentration of heterotrophic bacteria in water produced by ceramic candle filters with activated carbon increased with time of use, reaching a roughly constant level after five to seven weeks, which was maintained during the final weeks of this study.
It is also important to remember that treated water was analyzed following a 24-hour storage period, and therefore, these results are not strictly related to the removal rates promoted by the ceramic candle filters, but also related to the sedimentation process that may have occurred during the storage period. Moreover, the reference [36] observed that competition for space and resources could lead to selection of specific bacterial population, and therefore, competition could also have favored the growth of heterotrophic bacteria
Analyzing the results of heterotrophic bacteria in more details, the median increase observed in TT treating A1 (0.55 (0.50 – 0.60) log10) and A2 (0.55 (0.40 – 0.70) log10) were
significantly lower than the median increase observed in C1 (2.05 (1.93 – 2.10) log10, p- values = 8.78E-05) and C2 (2.35
(2.30 – 2.60) log10, p-values = 8.54E-05), respectively. On the other hand, the median increase in the concentration of heterotrophic bacteria when TAC was applied to treat A1 (2.03 (1.52 – 2.37) log10) was very close to the median increase observed in C1, whereas when TAC was used to treat A2, this increase (3.45 (2.55 – 3.70) log10) was significantly higher compared to C2 (p-value = 2.40E-02). It is relevant to mention that the lowest count of heterotrophic bacteria was observed in C3 (not detected in 100 mL of sample), showing that an adequate storage of non-filtered but well disinfected water
source, presenting relatively high level of free chlorine (> 0.80 mg/L), can be more adequate than its storage following any POU water treatment tested in this research, independently of the ceramic candle type.
Although the outcomes of this research indicated that the use of ceramic candle filters, traditional or with activated carbon, to treat contaminated and chlorinated water sources were very effective against thermotolerant coliform and enterococcus, the detected increase in the concentration of heterotrophic bacteria, especially when these filters were filled with activated carbon, may be of concern. There is some evidence that heterotrophic bacteria, which include opportunistic pathogens, are potentially harmful to human health [37], [38]. High levels of heterotrophic bacteria, such as the ones observed in this study, is very likely to be associated with higher counts of opportunistic pathogens, and consequently, it may pose excessive microbial risk to users, especially to the ones presenting weakened immune system such as neonates and elderly individuals [39].
On the other hand, the higher concentration of heterotrophic bacteria could be one of the main factors controlling the growth of FIB and other enteric pathogens, which in this case, having higher counts of heterotrophic bacteria could be desirable. In a study to evaluate bacterial responses to applied nutrient amendments, reference [40] observed that competition from heterotrophic bacteria suppressed E. coli growth in stream water. In order to support the development of more effective measures to protect public health, more research is needed to better understand the effect of competition for space and resources in the abundance and diversity of bacterial communities normally present in non-protected drinking water sources (e.g., FIB, pathogens and environmental microorganisms).
The identification of bacteria strains was not the objective of this study, but with the support of colleagues from the Federal University of São Paulo, some selected colonies of bacteria from chlorinated and/or filtered water were isolated and identified using Matrix Assisted Laser Desorption/Ionization – Time of Flight Mass Spectrometry (MALDI-TOF MS). All species of bacteria were identified with a score higher than 2.0, which was the same threshold to represent an accurate species-level identification used by reference [41]. Nearly all identified bacteria were opportunistic pathogens and included: Micrococcus luteus, Klebsiella pneumoniae, Bacillus cereus, Bacillus subtilis, Pseudomonas aeruginosa and Pseudomonas stutzeri. These results agree with the findings of previous researchers, who observed similar species in potable water samples from some rural habitations of Northern Rajsthan, India [42] and from a hospital in Hungary [43].
In order to keep stored water safer, future research should seek for more effective manners to make ceramic candle filters, including the feeding and storage reservoir, less prone to biofilm formation. Moreover, new disinfection solutions that could be applied to treat water stored in reservoirs of pot-type ceramic candle filters at the time of consumption could also contribute to improve effectivity and reliability of these simple low-cost filters, consequently improving public health.
-
CONCLUSION
The use of POU pot-type ceramic candle filters applied to treat contaminated water were very effective in reducing FIB counts and maintaining its quality during a 24-hour period but not as effective in reducing the concentration of heterotrophic bacteria, especially when ceramic candle filters with activated carbon were used. Moreover, although FIB were rarely detected when pot-type ceramic candle filters were applied to treat well- disinfected water, presenting high levels of free chlorine (> 1.5 mg/L), its use does not seem appropriate in such situation. The reduction of free chlorine to very low levels, especially when activated carbon was applied, allowed the growth of heterotrophic bacteria, which may be associated with an increase in the concentration of opportunistic pathogens, resulting in a higher microbial risk posed to users, especially to neonates and elderly individuals.
Therefore, the use of traditional ceramic candle filters would be more indicated to situations, in which microbial removal is the aim of the POU water treatment, whereas ceramic candle filters with activated carbon should be preferably used if POU water treatment targets the removal of chemical, although it may enhance the microbial risk. On the other hand, any of these filters should be used if water source is well-disinfected and presents adequate level of remaining free chlorine to avoid recontamination of drinking water during storage.
ACKNOWLEDGMENT
This research was made possible through the support of the program CAPES/CAPES Nº 10/2018 – PROFIX 2018, funded by CAPES and FAPES .
REFERENCES
-
Cotruvo J. A., Andrew R. & Herman R. 2014 Point of use and point of entry treatment technologies applicable in the home for controlling chemical, microbial, and aesthetic contaminants in drinking water. Comprehensive Water Quality and Purification, 2, 196-211 J. Clerk Maxwell, A Treatise on Electricity and Magnetism, 3rd ed., vol. 2. Oxford: Clarendon, 1892, pp.68-73.
-
Sobsey M.D., Stauber C.E., Casanova L.M., Brown J.M. & Elliott M.A. 2008 Point of use household drinking water filtration: a practical, effective solution for providing sustained access to safe drinking water in the developing world. Environmental Science Technology, 42, 4261- 4267.
-
Peter-Varbanets M., Zurbrügg C., Swartz C. & Pronk, W. 2009 Decentralized systems for potable water and the potential of membrane technology, Water Research, 43, 245-265.
-
Bielefeldt A.R., Kowalski K. & Summers R.S. 2009 Bacterial treatment effectiveness of point- of-use ceramic water filters. Water Research, 43(14), 3559-3565.
-
Bellingieri J.C. 2006 Uma análise da indústria de filtros de água no Brasil (An analysis of the water filter industry in Brazil). Cerâmica Industrial, 11(3), 31-35.
-
Brazilian Institute of Geography and Statistics (IBGE). Pesquisa nacional por amostra de domicÃlios: domicÃlios particulares permanentes, por posse de filtro de água (National household sample survey: private permanent households, by presence of water filter). Brasilia, D.F., Brazil. https://seriesestatisticas.ibge.gov.br/series.aspx?vcodigo=PD275 (accessed 26 February 2020)
-
Centre for Affordable Water and Sanitation Technology (CAWST) 2018 Ceramic candle filter fact sheet. Calgary, Canada. https://www.hwts.info/products-technologies/a48a2cee/cera mic-candle- filter/technical-information (accessed 09 March 2020).
-
Centers for Disease Control and Prevention (CDC) 2020 Choosing home water filters & other water treatment systems. Atlanta, Georgia, United
States. https://www.cdc.gov/media/ releases/2017/p1205-screening-
congenital-heart-disease.html (accessed 03 March 2020).
-
World Health Oganization (WHO) n.d. Physical removal processes: sedimentation and filtration. Geneva, Switzerland. https://www.who.int/water_sanitation_health/dwq/WSH02. 07_ 4.pdf (accessed 25 February 2020) .
-
Centers for Disease Control and Prevention (CDC) 2012a Household water treatment: ceramic filtration. Atlanta, Georgia, United States. https://www.cdc.gov/safewater/ceramic- filtration.html (accessed 03 March 2020).
-
Gusmão P.T.R. 2008 Manual de orientações filtro doméstico (Guidelines – domestic filter). Department of Civil Engineering, Federal University of Pernambuco. Recife, Pernambuco, Brazil.
-
Djaouda M., Nola M., Togouet S.H.Z., Nougang M.E., Djah M. & Njiné
T. 2010 Modifications des propriétés dabondance bactériennes des eaux souterraines en réponse à une longue durée de stockage dans les récipients à domicile à Yaoundé (Cameroun). European Journal of Water Quality, 41(2), 131-143.
-
Mwabi J.K., Adeyemo F.E., Mahlangu T.O., Mamba B.B., Brouckaert B.M., Swartz C.D., Offringa G., Mpenyanamonyatsi L. & Momba
M.N.B. 2011 Household water treatment systems: a solution to the production of safe drinking water by the low-income communities of Southern Africa. Journal Physics and Chemistry of the Earth, 36(14-15), 1120-1128.
-
Perez-Vidal A., Diaz-Gomez J., Castellanos-Rozo J. & Usaquen-Perilla
O.L. 2016 Long- term evaluation of the performance of four point-of-use water. Water research, 98, 176-182 .
-
Alsulaili A., Al-Harbi M. & Elsayed K. 2020 The influence of household filter types on quality of drinking water. Process Safety and Environmental Protection, 143, 204-211.
-
Sagara J. 2000 Study of filtration for point-of-use drinking treatment in Nepal. Masters Thesis, Department of Civil Engineering and Environment, Massachusetts Institute of Technology, Cambridge, Massachusetts, United States.
-
Duke W.F., Nordin R. & Mazumder A. 2006 Comparative analysis of the filtron and biosand water filters, University of Victoria, British Columbia.
-
Van Halem D. 2006 Ceramic silver impregnated pot filters for household drinking water treatment in developing countries. Masters Thesis, Faculty of Civil Engineering, Delft University of Technology, Delft, Netherland.
-
Oyanedel-Craver V.A. & Smith J.A. 2008 Sustainable colloidal silver impregnated ceramic filter for point-of-use water treatment. Environmental Science and Technology, 42, 927-933.
-
Environmental Protection Agency (EPA) 2011 Finalization of guidance on incorporation of water treatment effects on pesticide removal and transformations in drinking water exposure assessments, Washington, D.C., United States. https://www.epa.gov/pesticide-science- and- assessing-pesticide-risks/finalization-guidance-incorporation-water- treatment (accessed 15 March 2020).
-
Sobsey M.D. 2002 Managing water in the home: accelerated health gains
from improved water supply, Geneva, Switzerland.
-
Wu C.C., Ghosh S., Martin K.J., Pinto A.J., Denef V.J., Olson T.M. & Love N.G. 2017 The microbial colonisation of activated carbon block point-of-use (PoU) filters with and without chlorinated phenol disinfection by-products. Environmental Science: Water Research & Technology, 3, 830-843.
-
World Health Organization (WHO) 2001 Water Quality: Guidelines, Standards and Health. Edited by Lorna Fewtrell and Jamie Bartram. Published by IWA Publishing, London, UK.
-
Ministério da Saúde (MS) 2017 Portaria de Consolidação no 5 Consolidação das normas sobre as ações e os serviços de saúde do sistema único de saúde (Consolidation Ordinance no 5 Consolidation of standards on health services and actions of the national unified health system), BrasÃlia, D.F., Brazil.
-
Baird, R., & Bridgewater, L. (2017). Standard methods for the examination of water and wastewater. 23rd edition. Washington, D.C.: American Public Health Association.
-
R Core Team 2020 R: A language and environment for statistical computing. R Foundation for Statistical Computing, Vienna, Austria. https://www.R-project.org/
-
RStudio Team (2020). RStudio: Integrated Development for R. RStudio – PBC, Boston, Massachusetts, United States. http://www.rstudio.com/
-
Su F., Luo M., Zhang F., Li P., Lou K. & Xing X. 2009 Performance of microbiological control by a point-of-use filter system for drinking water purification. Journal of Environmental Sciences, 21, 1237-1246.
-
Tavares R.G., Gusmão A.C.S., Silva R.S.O., Silva G.F.M., Andrade
P.K.B. & Rocha E.A. 2020 Alteração fÃsico-quÃmica da água para consumo humano após uso de filtros domésticos (Physico-chemical modification of water for human consumption after using domestic filters). Scientific Journal of Environmental Sciences and Biotechnology, 6(1), 58- 63.
-
Centers for Disease Control and Prevention (CDC) 2012b Effect of chlorination on inactivating selected pathogen. Atlanta, Georgia, United States. https://www.cdc.gov/safe water/effectiveness-on-pathogens.html (accessed 04 March 2020).
-
Krishna K.C.B., Sathasivan A., Cox P. & Doolan C. 2020 Bacterial community including opportunistic pathogens dynamics in a chlorine tablet-dosed chloraminated service reservoir and reticulation pipes. International Biodeterioration & Biodegradation, 153.
-
Yi J.C., Lee J., Jung H., Park P.K. & Noh S.H. 2018 Reduction of bacterial regrowth in treated water by minimizing water stagnation in the filtrate line of a gravity-driven membrane system. Environmental Engineering Research, 24(1), 17-23.
-
Di Bernardo L. & Dantas, A.D.B. 2006 Métodos e técnicas de tratamento de água (Water treatment methods and techniques). Environmental and Sanitation Engineering, Rio de Janeiro, Brazil.
-
Clasen T. & Menon S. 2007 Microbiological performance of common water treatment devices for household use in India. International Journal of Environmental Health Research, 17(2), 83-93.
-
Peres M.R., Ebdon J., Purnell S. & Taylor H. 2020 Potential microbial transmission pathways in rural communities using multiple alternative water sources in semi-arid Brazil. International Journal of Hygiene and Environmental Health, 224, 1-13.
-
Hibbing M.E., Fuqua C., Parsek M.R. & Peterson S.B. 2010 Bacterial competition: surviving and thriving in the microbial jungle. Nature Reviews: Microbiology, 8(1), 15-25.
-
Edberg S.C. & Allen M.J. 2004 Virulence and risk from drinking water of heterotrophic plate count bacteria in human population groups. International Journal of Food Microbiology, 92, 255-263.
-
Pavlov D., De Wet M.E., Grabow W.O.K. & Ehlers M.M. 2004 Potentially pathogenic features of heterotrophic plate count bacteria isolated from treated and untreated drinking water. International Journal of Food Microbiology, 92, 275287.
-
World Health Organization (WHO) 2017 Guidelines for drinking water quality: 4th edition. Geneva, Switzerland. http://apps.who.int/iris/bitstream/handle/10665/254637/978924154
9950-eng.pdf?sequence=1 (accessed 10 March 2020).
-
Gregory L.F., Karthikeyan R., Aitkenhead-Peterson J.A., Gentry T.J., Wagner K.L. & Harmel R.D. 2017 Nutrient loading impacts on culturable
E. coli and other heterotrophic bacteria fate in simulated stream mesocosms. Water Research, 126, 442-449 .
-
Li Y., Shan M., Zhu Z., Mao X., Yan M., Chen Y., Zhu Q. & Li H. 2019 Application of MALDI- TOF MS to rapid identification of anaerobic bacteria. BMC Infectious Diseases, 19, 941.
-
Suthar S., Singh S. & Chhimpa V. 2009 Bacterial contamination in drinking water: a case study in rural areas of northern Rajasthan, India. Environmental and Monitoring Assessment, 159, 43-50.
-
Felföldi T., Heéger Z., Vargha M. & Márialigeti K. 2010 Detection of potentially pathogenic acteria in the drinking water distribution system of a hospital in Hungary.Clinical Microbiology and Infection, 16, 8992.