
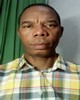
- Open Access
- Authors : Kayode Idowu , Roland Uhunmwangho , Okafor E.C.N
- Paper ID : IJERTV9IS050346
- Volume & Issue : Volume 09, Issue 05 (May 2020)
- Published (First Online): 08-06-2020
- ISSN (Online) : 2278-0181
- Publisher Name : IJERT
- License:
This work is licensed under a Creative Commons Attribution 4.0 International License
The Effect of Distributed Generation on Distribution Network Stability
Kayode Idowu1, Roland Uhunmwangho2, Okafor E.C.N3.
-
Department of Electrical/Electronic Engineering, University of Port Harcourt, Choba P.O. Box 5323, Port Harcourt, Rivers State, Nigeria.
-
Department of Electrical/Electronic Engineering, University of Port Harcourt, Choba P.O. Box 5323, Port Harcourt, Rivers State, Nigeria.
-
Department of Electrical and Electronics Engineering, Federal University of Technology, Owerri, Imo State, Nigeria.
Abstract:- The abysmal performance of power sector in Nigeria requires an urgent attention. A deliberate and effective action must be taken by Power Utilities to provide lasting solutions to this problem that has lasted for decades. In order to meet the electricity demands of consumers, alternative means of generation in addition to the traditional centralized system of generation has been proposed in this paper. Distributed Generation (DG) is an excellent way that can be employed to solve the afore-mentioned problem. The transient stability of Port Harcourt distribution network is studied when DG unit is integrated into it. The distribution system performance with different levels of DG penetration is investigated when a balanced three phase fault is applied to the network. The result shows an improvement in the transient stability of the network. The power angle swings to 50 degree approximately without DG and improved to 38 degree with DG.
Keywords: Distributed generation, transient stability, three-phase short circuit fault.
1. INTRODUCTION
The concern for the greenhouse gas emission and the need to eliminate the unnecessary cost associated with transmission system have opened the window for large-scale deployment of Distributed Generation (DG) in distribution system [11]. Today DG has started to have a significant contribution in electric power system in the developed countries of the world but in Nigeria, the benefit of DG is yet to be tapped. Generation of electricity using DG technology is still at the primary stage despite the abundance availability of renewable energy resources in the country [4] [6].
DG involves integration of small and medium size electric generator in power system network to form alternative source of power generation. Power utilities and consumers can benefit from the utilization of DG, because it offers several technical, environmental and economic benefits. Renewable resources such as wind, solar and small hydro are employed by DG technology to generate electricity. [1] [2].
High penetration level of DG into power distribution network may lead to several problems, regarding system stability, power quality and control. This may also create problems to the control of large central generators and to behaviour of loads in the power system. The addition of DG to distribution network will make power generation from
central generator to reduce which may be a major issue during occurrence of major disturbance.[8][9]
The impact of DG at low percentage level of penetration on the distribution network may not be considered as significant, but as its penetration increases, the impact will not only affect the distribution network but also influences the entire network.[11]
In this paper, the aim is to study how DG affects the transient stability of a distribution network. A section of Port- Harcourt Electricity distribution network was used for this study. ETAP 16.0 is the electrical software that was used to model the network [3]. The capacity of each DG unit employed is 3 MW. DG units were attached to the load buses in the low-voltage area of the network. The study was done by making load demands constant. DG units were connected to different load buses in the network. The response of the network was investigated. The behaviour of the network with and without DG was studied and analysed.
2.0 POWER SYSTEM STRUCTURE NETWORK IN NIGERIA.
The alternating current generation voltage in Nigeria is either 11kV or 16kV. The terminal rated voltage of synchronous generators is 10.5, 11,11.5 and 16 kV with operating frequency of 50Hz. In the transmission network, the grid voltage is 330 kV.[6] The secondary transmission voltage is 132 kV. In the distribution section, we have primary distribution feeder voltage of 33 kV in various injection substations and secondary distribution feeders voltage of 11 kV to various consumers on point load. The tertiary distribution section comprises of 11/0.415 kV transformers that distribute powers to consumers on 4-wire system. The domestic loads are fed on 415V on three phase system and 240V on single phase system.
-
MATERIALS AND METHODS.
-
Study Area.
Port-Harcourt Electricity Distribution (PHED) network is used to carry out simulation study of the transient stability problem. At first, this distribution network did not contain distributed generation. Subsequently Distributed Generation was attached to the network while the load was kept constant. DG units was integrated into the network at different buses in the test distribution network. In order to investigate the impact that Distributed Generation will have
on the network transient stability, a fault was initiated to happen on the 33 kV bus. The response of the system was studied. The penetration level can be calculated using equation 1.
5.0 DESCRIPTION OF TEST NETWORK.
A section of the Port Harcourt electricity distribution network in Port Harcourt metropolis is used for this study. The test system used was not large to reduce the computation time and reduce the number of possible scenarios. The Port
(%) =
(1)
. 100 %.
Harcourt Mains Transmitting station known as Z2 at Oginigba with the associated 33kV feeders and transformers were modelled. The Port Harcourt Mains Transmitting
4.0 TRANSIENT STABILITY INDICATORS.
X
X
X
X
X X X
X X X
132KV BUS
132KV BUS
In determining the impact that will be experienced in the distribution network due to integration of DG units into it, an indicator is used. This indicator is used in reference to oscillations of the rotor speed of the synchronous generator at Afam generating station. Maximum rotor speed deviation is chosen as the indicator.
station known as Z2 is one of the transmitting stations of this company in Port-Harcourt metropolis. It is a 132/33 kV transmitting station consisting of 3X 60MVA, 132/33 kV transformers. There are eleven 33 kV feeders from this transmitting station. Ten feeders supply different customers made up of domestic, commercial and industrial customers while the remaining one is a spare feeder. Figures 1 and 2 show the single line diagrams of the 33 kV bus system of the network. Table 1 shows the characteristics of the feeders.
PH. MAINS T/S (Z2)
PH. MAINS T/S (Z2)
Line 1 from Afam
Line 2 from Afam
Line 1 from Afam
Line 2 from Afam
From T-Amadi Gas Turbine Station.
From T-Amadi Gas Turbine Station.
33 kV Feeders 1 2 3 4 5 6 7 8 9 10 11
33 kV Feeders 1 2 3 4 5 6 7 8 9 10 11
X
X
X
X
X
X
T2A
60MVA
132/33KV
T/F
T2A
60MVA
132/33KV
T/F
T1A
60MVA
132/33KV
T/F
T1A
60MVA
132/33KV
T/F
T3A
60MVA
132/33KV
T/F
T3A
60MVA
132/33KV
T/F
X X X
33KV BUS
<>X X X
33KV BUS
33 kV Feeders
1 T/Amadi (RSPUB) , 17.1 MW, 8.19 km.
-
Spare.
-
Rainbow 33KV Feeder 13.7 MW, 12.515Km.
-
Oyigbo 33 KV Line, 11.5 MW.
-
RF LINE 2, 33 KV Feeder , 16MW, 5.1Km
-
Rumuodomaya feeder 22.1 MW, 55.42Km.
-
Abuloma 33kv fEEDER, 9.5 MW, 11.825 Km
-
Woji 33KV Feeder, 7.6mw, 9.Km
-
Rumuola Feeder 3, 15.8 MW, 18.2 Km
-
Akani Feeder.
-
Airport Feeder 33KV Feeder 21MW, 45.3Km.
33 kV Feeders
1 T/Amadi (RSPUB) , 17.1 MW, 8.19 km.
-
Spare.
-
Rainbow 33KV Feeder 13.7 MW, 12.515Km.
-
Oyigbo 33 KV Line, 11.5 MW.
-
RF LINE 2, 33 KV Feeder , 16MW, 5.1Km
-
Rumuodomaya feeder 22.1 MW, 55.42Km.
-
Abuloma 33kv fEEDER, 9.5 MW, 11.825 Km
-
Woji 33KV Feeder, 7.6mw, 9.Km
-
Rumuola Feeder 3, 15.8 MW, 18.2 Km
-
Akani Feeder.
-
Airport Feeder 33KV Feeder 21MW, 45.3Km.
Source: Transmission Company of Nigeria. .[11]
Figure 1: Port Harcourt Main Zone 2 Transmission Station, Oginigba.
AKANI(FDR 2), (14.5MW), 0.22KM
AKANI(FDR 2), (14.5MW), 0.22KM
FISHRY. 1×1.0MVA
33/0.415KV, T/F.
FISHRY. 1×1.0MVA
33/0.415KV, T/F.
AIR LIQUID. 1×4.5MVA
33/11KV T/F.
AIR LIQUID. 1×4.5MVA
33/11KV T/F.
RIVOC INJ. 1×7.5MVA
33/11KV T/F.
RIVOC INJ. 1×7.5MVA
33/11KV T/F.
STALION 1 1×2.5MVA
33/11KV T/F.
STALION 1 1×2.5MVA
33/11KV T/F.
T2
T2
T1
T1
GALBA. 1×1.5MVA
33/11KV T/F.
GT( RI V OC)
GALBA. 1×1.5MVA
33/11KV T/F.
GT( RI V OC)
OIL & INDUSTRIES 1.5MVA
33/0.415KV
S/S
OIL & INDUSTRIES 1.5MVA
33/0.415KV
S/S
TRANS AMADI. 2x15MVA
33/11KV INJ.S/S.
TRANS AMADI. 2x15MVA
33/11KV INJ.S/S.
FORMER
PHCN ZONAL OFFICE. 1x500KVA
33/0.415KV T/F.
FORMER
PHCN ZONAL OFFICE. 1x500KVA
33/0.415KV T/F.
TRANS AMADI GARDENS. 1×2.5MVA
33/11KV T/F.
TRANS AMADI GARDENS. 1×2.5MVA
33/11KV T/F.
D
D
From Feeder 1
T/AMADI (RSPUB), (17.1MW), 8.19KM
FIRST ALUM. 1×7.5MVA
33/11KV T/F.
ELF NIG. 2×7.5MVA
33/11KV
T/Fs.
FIRST ALUM. 1×7.5MVA
33/11KV T/F.
ELF NIG. 2×7.5MVA
33/11KV
T/Fs.
BEKEMS PROPERTY 2.5MVA
33/0.415KV
S/S
BEKEMS PROPERTY 2.5MVA
33/0.415KV
S/S
FEMI E
WATER WORKS
RI V OC NDA BROS
FEMI E
WATER WORKS
RI V OC NDA BROS
Source: Transmission Company of Nigeria. .[11]
Figure 2: Single Line Diagram of Trans-Amadi 33 kV Feeder.
6.0 TRANSMISSION LINES PARAMETER.
Table 1: Port-Harcourt Distribution Parameters. (33kV Feeder)
S/n |
33kV Feeder Line |
Line Load (MW) |
Length (KM) |
Size (mm2) |
1 |
Refinery Line 2 |
17.6 |
5.1 |
150 mm2 |
2 |
Rumuola Feeder3 |
15.8 |
18.2 |
150 mm2 |
3 |
Rumuodomaya Feeder |
23 |
55.42 |
150 mm2 |
4 |
Airport Feeder |
20.9 |
45.3 |
150 mm2 |
5 |
Oyigbo |
9.3 |
19 |
150 mm2 |
6 |
Woji |
7.6 |
9 |
150 mm2 |
7 |
Abuloma |
9.5 |
11.82 |
150 mm2 |
8 |
Trans-Amadi Feeder |
16.7 |
8.19 |
150 mm2 |
9 |
Akani Feeder2 |
13.6 |
0.22 |
150 mm2 |
10 |
Rainbow Feeder |
13.7 |
12 |
150 mm2 |
7.0 SIMULATION PROCEDURE
In this study, three 33 kV feeders supplied from one 60 MVA transformer in Port-Harcourt Zone 2 transmission station of the network was modelled as a case study.The three feeders are Trans-Amadi, Refinery 2 and Rainbow feeders. The size, length and load demand on the feeders are shown in table 1. A three-phase fault was applied to the 132 kV main bus and the network simulations were carried out both with and without DG units. The fault was made to happen at 0.5 secs and cleared at 0.6 secs.
8.0 ANALYSIS OF RESULT
Figure 3 shows the rotor angle variation due to the three- phase fault without the addition of DG in the system. Figure 4 shows the angular variation with the attachment of DG to the network load buses. Figure 6 shows the ETAP modelling and transient stability result of the network. Table 2 shows the transient stability result.
Table 2: Transient Stability Result
Time. |
Angle |
Time. |
Angle |
Time. |
Angle |
|||||
Secs. |
Without DG. |
With DG. |
Secs. |
Without DG. |
With DG |
Secs. |
Without DG. |
With DG |
||
0.0000 |
-3.33 |
-3.33 |
1.7210 |
-34.66 |
-24.61 |
3.4810 |
1.24 |
-2.09 |
||
0.0400 |
-3.33 |
-3.33 |
1.7610 |
-31.24 |
-18.47 |
3.5210 |
-2.49 |
-6.57 |
||
0.0800 |
-3.33 |
-3.33 |
1.8010 |
-24.34 |
-9.85 |
3.5610 |
-6.32 |
-10.48 |
||
0.1200 |
-3.33 |
-3.33 |
1.8410 |
-15.21 |
0.03 |
3.6010 |
-9.66 |
-13.33 |
||
0.1600 |
-3.33 |
-3.33 |
1.8810 |
-5.20 |
9.86 |
3.6410 |
-12.05 |
-14.78 |
||
0.2000 |
-3.33 |
-3.33 |
1.9210 |
4.37 |
18.36 |
3.6810 |
-13.15 |
-14.69 |
||
0.2400 |
-3.33 |
-3.33 |
1.9610 |
12.36 |
24.23 |
3.7210 |
-12.87 |
-13.16 |
||
0.2800 |
-3.33 |
-3.33 |
2.0010 |
17.78 |
26.5 |
3.7610 |
-11.30 |
-10.49 |
||
0.3200 |
-3.33 |
-3.33 |
2.0410 |
19.97 |
24.44 |
3.8010 |
-8.74 |
-7.15 |
||
0.3600 |
-3.33 |
-3.33 |
2.0810 |
18.72 |
18.22 |
3.8410 |
-5.61 |
-3.68 |
||
0.4000 |
-3.33 |
-3.33 |
2.1210 |
14.38 |
9.06 |
3.8810 |
-2.42 |
-0.62 |
||
0.4400 |
-3.33 |
-3.33 |
2.1610 |
7.70 |
-1.56 |
3.9210 |
0.36 |
1.6 |
||
0.4800 |
-3.33 |
-3.33 |
2.2010 |
-0.27 |
-12.22 |
3.9610 |
2.34 |
2.71 |
||
0.5010 |
-3.33 |
-3.33 |
2.2410 |
-8.45 |
-21.49 |
4.0010 |
3.26 |
2.64 |
||
0.5410 |
-3.69 |
-3.68 |
2.2810 |
-15.77 |
-28.15 |
4.0410 |
3.01 |
1.45 |
||
0.5810 |
-4.69 |
-4.64 |
2.3210 |
-21.28 |
-31.23 |
4.0810 |
1.70 |
-0.6 |
||
0.6210 |
-6.29 |
-6.14 |
2.3610 |
-24.24 |
-30.31 |
4.1210 |
-0.43 |
-3.16 |
The rotor angle maximum deviation of synchronous generator has been chosen as an indicator to judge the nature of the transient stability.[8]. The output plot of the system without DG is shown in figure 3 while the one with DG addition is shown in figure 4. Looking at the two profiles, when the fault occurred, the generator power angle swing to 50 degree approximately without DG but to about 38 degree when DG was added to the network. The first swing in the two plots show that the addition of DG units makes the
magnitude of the maximum rotor angle deviation to decrease. This is a proof that DG units are able to bring significant improvement to system stability. We can also explain that increase in the percentage level of DG units in distribution network provides means to successfully deal with larger disturbances. Integration of DG into a distribution network provide means to reduce maximum rotor angle deviation during a severe disturbance and thereby maintain synchronism in the system.
40.00
Generator Rotor Angle with no DG
40.00
Generator Rotor Angle with no DG
20.00
0.00
-20.00
-40.00
-60.00
20.00
0.00
-20.00
-40.00
-60.00
0.00
0.28
0.54
0.82
1.08
1.36
1.64
1.92
2.20
2.48
2.76
3.04
3.32
3.60
3.88
4.16
4.44
4.72
5.00
5.28
5.56
5.84
6.12
6.40
6.68
6.96
7.24
7.52
7.80
8.08
8.36
8.64
8.92
9.20
9.48
9.76
0.00
0.28
0.54
0.82
1.08
1.36
1.64
1.92
2.20
2.48
2.76
3.04
3.32
3.60
3.88
4.16
4.44
4.72
5.00
5.28
5.56
5.84
6.12
6.40
6.68
6.96
7.24
7.52
7.80
8.08
8.36
8.64
8.92
9.20
9.48
9.76
Figure 3: Rotor Angle variation with no DG
Generator Rotor Angle with DG connected.
40
20
0
-20
-40
-60
Generator Rotor Angle with DG connected.
40
20
0
-20
-40
-60
0.00
0.28
0.54
0.82
1.08
1.36
1.64
1.92
2.20
2.48
2.76
3.04
3.32
3.60
3.88
4.16
4.44
4.72
5.00
5.28
5.56
5.84
6.12
6.40
6.68
6.96
7.24
7.52
7.80
8.08
8.36
8.64
8.92
9.20
9.48
9.76
0.00
0.28
0.54
0.82
1.08
1.36
1.64
1.92
2.20
2.48
2.76
3.04
3.32
3.60
3.88
4.16
4.44
4.72
5.00
5.28
5.56
5.84
6.12
6.40
6.68
6.96
7.24
7.52
7.80
8.08
8.36
8.64
8.92
9.20
9.48
9.76
Figure 4: Rotor Angle variation with DG
Generator Rotor Angle with and without DG
Generator Rotor Angle with and without DG
40
30
20
10
0
-10
-20
-30
-40
-50
-60
40
30
20
10
0
-10
-20
-30
-40
-50
-60
Without DG
With DG
Without DG
With DG
(sec.)
0.2
0.48
0.741
1.001
1.281
1.561
1.841
2.121
2.401
2.681
2.961
3.241
3.521
3.801
4.081
4.361
4.641
4.921
5.201
5.481
5.761
6.041
6.321
6.601
6.881
7.161
7.441
7.721
8.001
8.281
8.561
8.841
9.121
9.401
9.681
9.961
(sec.)
0.2
0.48
0.741
1.001
1.281
1.561
1.841
2.121
2.401
2.681
2.961
3.241
3.521
3.801
4.081
4.361
4.641
4.921
5.201
5.481
5.761
6.041
6.321
6.601
6.881
7.161
7.441
7.721
8.001
8.281
8.561
8.841
9.121
9.401
9.681
9.961
Figure 5: Rotor Angle variation with DG and without DG superimposed.
Figure 6: ETAP Model of Distribution Network.
9.0 CONCLUSION
The 33 k V distribution network of Port Harcourt Electricity Distribution Network was used as a case study. In the base case, no DG was attached to the network and simulation was done at constant load. The percentage level of DG was increased from 10% to 40%. The study shows that the influence of DG on power system stability depends on the percentage level of DG penetration. At low percentage level
of DG penetration, the stability of the network was the same with the base case but with high percentage level of penetration the stability improved.
REFERENCES
-
Ackermann, T, Anderson G, and Soder, L (2001), Distributed generation: a definition. Electric Power System Research, vol.57 195-204.
-
Angelo, L, Gianluca, F, Fred, S and Stathis, D. (2007) Distributed Power Generation in Europe: technical issues for further integration.
-
Operation Technology Inc, (2001) Electrical Transient Analyzer Program (ETAP).
-
Hachimenum, N, A (2017): Distributed Generation in Nigeria`s Post-Privatized Power
SectorChallengesandProspects.Availableonlinehttps://www.rese archgate.net/publication
-
Idowu, K; Ogbonaya, I; and Eseosa, O (2017). Voltage Stability Analysis of Nigeria Power System Using Distributed Generation. Applied Research Journal.
-
Okereafor, F C, Idoniboyeobu D, C and Bala T (2017): Analysis of 33/11 kV Injection Substation for Improved Performance with Distributed Generation Units.American Journal of Engineering Research (AJER), Volume6, Issue-9, pp-301-316.
-
Operation Technology Inc, (2001) Electrical Transient Analyzer Program (ETAP)
-
Sharma, D and Bartels, R (1998) Distributed electricity generation in competitive energy markets: a case study in Australia. The energy Journal Special issue Clevland, Ohio USA.
-
Slootweg, J and Kling, W (2002) Impacts of distributed generation on power system transient stability. Power Engineering Society Summer Meeting, 2002 IEEE, Vol 2, 21-25.
-
Transmission Company of Nigeria, Single Line diagram of Port Harcourt. Unpublished 2019.
-
Valerijs K, and Ackermann T. (2003). Interaction between the Distributed Generation and Distribution Network: Operation, Control, and Stability Aspects. In CIRED 17th International Conference on Electricity Distribution, Brcelona.