
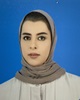
- Open Access
- Authors : Manal Ali, Hamada Esmaiel
- Paper ID : IJERTV11IS010184
- Volume & Issue : Volume 11, Issue 01 (January 2022)
- Published (First Online): 09-02-2022
- ISSN (Online) : 2278-0181
- Publisher Name : IJERT
- License:
This work is licensed under a Creative Commons Attribution 4.0 International License
Symmetric PAM-DMT for Spectrum and Energy Efficient Lifi Systems
Manal Ali
Electrical Engineering Department, Faculty of Engineering, Aswan University,
Aswan 81542, Egypt
Hamada Esmaiel
Electrical Engineering Department, Faculty of Engineering, Aswan University, Aswan 81542, Egypt
Department of Information and Communication, School of Informatics, Xiamen University, Xiamen 316005, China
Abstract A novel digital modulation technique based on orthogonal frequency division multiplexing (OFDM) is proposed for light fidelity (LiFi). The proposed technique is applicable to all OFDM-based intensity modulated and direct detected (IMM/DD) systems. DC biased optical OFDM (DCO-OFDM) is widely used in high-speed LiFi systems. However, the high peak- to-average-power-ratio (PAPR) of OFDM signals requires a high DC bias, which makes it energy inefficient for mobile communications. The proposed technique builds on the principles of pulse amplitude modulation (PAM-DMT) but improves the design by doubling the spectral efficiency and reducing the computational complexity. The proposed scheme offers an energy-efficient alternative to unipolar OFDM schemes at high spectra efficiencies since the gain of the scheme increases with the increased modulation size. For example, a gain of 2.75 dB is achieved over PAM-DMT at a spectral efficiency of 1 bits/s/Hz, whereas a significant 20 dB gain is achieved when our proposed technique is compared with PAM-DMT at a spectral efficiency of 5 bits/s/Hz. This is ideal for uplink communication in LiFi since the mobile terminal battery capacity is limited, thanks to the power-hungry mobile applications.
Keywords Light fidelity (LiFi), Optical orthogonal frequency division multiplexing (OFDM), pulse amplitude modulation discrete multitone (PAM-DMT)
-
INTRODUCTION
The demand for high-speed wireless access has increased significantly. Global mobile data traffic is estimated to grow by a factor of 4.5 to reach 226 Exabyte per month in 2026 [1]. The growth of mobile data traffic is driven by the improved mobile devices capabilities and data-intensive contents [1]. The radio frequency (RF) spectrum is limited and unable to sustainably support this exponential increase in traffic demand and wireless access. Alternative frequency bands are undeniably required to satisfy the worldwide demand for wireless communication. It is widely acknowledged that the optical domain is a promising candidate to support this highly-demanded indoor traffic.
Visible light communication
(VLC) is aiming at reusing the existing lighting infrastructure to enable an energy-efficient telecommunication links that do not require any licensing [2]. Light fidelity (LiFi) takes the concept of peer-to-peer visible light links into the next level by enabling atto-cellular networks that support handover and mobility [3-4].
LiFi modulation techniques are primarily based on intensity modulation and direct detection (IM/DD). This is mainly since LiFi is expected to be enabled using off-the-shelf light emitting diode (LED) and photodiode (PD), since LiFi is assumed to
reuse the available inexpensive lighting infrastructure. Therefore, advanced coherent modulation techniques cannot be realized due to the lack of coherence in the light output of an LED. Off the shelf LED can produce incoherent light, therefore, the information can be encoded in the light intensity. Pulsed modulation techniques such as pulse-amplitude modulation (PAM), binary phase shift keying (BPSK), and pulse width modulation (PWM) can implemented by simply feeding the baseband signal into the LED. However, the major problem of these modulation techniques is the increased inter- symbol interference (ISI) when the symbol rate increases. This is mainly driven by the low pass filter (LPF) characteristic of the conventional LED and the frequency selectivity that could arise from the non-line-of-sight (LoS) optical wireless communication (OWC) channels.
Given the fact that off-the-shelf LED have a low modulation bandwidth (between 3-20 MHz) [5]. It is of paramount importance that LiFi modulation techniques can support high spectral efficiencies. Orthogonal frequency division multiplexing (OFDM) is one of the main suitable candidates to satisfy the requirement of spectral efficiency due to its support of bit and power loading algorithms that can adapt the OWC channel utilization to the possible capacity [6]. Conventional RF domain OFDM techniques can be adopted in the optical domain. However, the optical signal output of a conventional LED can only be real-valued and unipolar. Therefore, the modulation waveform needs to be both real- valued and positive. Hermitian symmetry is usually used in conventional direct current (DC) to enable a real-valued output of the OFDM modulator. Whereas a DC bias is usually added to shift the negative samples of the waveform to become positive. However, the addition of the DC bias results in an additional power dissipation that is not directly used in information transmission. Several different modulation techniques have been proposed to address the problem of spectrum efficiency in conventional DC-biased optical OFDM (DCO-OFDM).
Unipolar OFDM techniques attempt to use the properties of Fourier transformation to adjust the output of the OFDM modulator. For example, pulse-amplitude modulation discrete multitone modulation (PAM-DMT) allows for the time domain waveform to be unipolar by loading symbols on the imaginary component of the sub-carriers [7]. This allows the signal to be clipped at zero levels with a fixed power penalty of 3 dB. Therefore, it can enable data transmission over LED in LiFi. However, PAM-DMT uses 1-D modulation schemes such as
Fig. 1. The block diagram of the transmitter of SPAM-DMT. IFFT denotes IFFT; P/S denotes a parallel to serial conversion.
PAM, whereas DCO-OFDM uses 2-D schemes such as QAM. Therefore, it can achieve the same spectral efficiency of DCO- OFDM. However, it requires a higher signal-to-noise ratio (SNR) as the bit error ratio (BER) performance of M-ary quadrature amplitude modulation (M-QAM) is equivalent to the BER performance of M2-QAM [8]. these unipolar modulation techniques have a disadvantage that prevents their use when high-spectral efficiencies are required. Asymmetrically clipped optical OFDM (ACO-OFDM) restricts the data loading into the odd-indexed sub-carriers to obtain an asymmetry property in the time-domain output waveform [9]. ACO-OFDM uses only half of the sub-carriers, therefore the spectral efficiency of ACO-OFDM is half of that in DCO- OFDM.
In this paper, we propose a modification for PAM-DMT that allows it to cut the waveform size in half, and therefore, allows for higher spectral efficiency. The proposal is based on building asymmetry in the time-domain waveform so that only half of the OFDM frame is required to be transmitted. This is achieved in the frequency domain by loading the data into the real component of the sub-carriers. Therefore, our proposed modulation technique can transmit twice the information of that in PAM-DMT which improves the spectral efficiency of unipolar OFDM modulation techniques for LiFi systems. The paper starts with investigating time-domain symmetry properties that can enable improvements in the energy and spectral efficiencies. A new technique is proposed based on creating a time-domain symmetry. Afterwards, we investigated the DC-bias requirements of the newly proposed technique. This is achieved by performing Monte-Carlo simulation study on all the possible biasing points. The optimal DC bias points are then selected and used in a differet Monte-Carlo simulation study to investigate the BER performance of the proposed technique and compare it to DCO-OFDM and PAM- DMT.
This paper is organized as follows, Section 2 briefly introduce the PAM-DMT modulation techniques, Section 3 discuss the proposed modulation technique symmetrical pulse- amplitude modulation discrete multitone modulation (SPAM- DMT) and explains its block diagram and basic properties. Section 4 presents the simulation results and discusses the main findings, and finally, Section 5 concludes the paper.
-
PAM-DMT
The generation of PAM-DMT starts with a conventional OFDM modulator, as shown in Fig.1 and Fig.2. However, the modulation format of PAM is chosen instead of QAM. This is
because PAM-DMT loads data on the imaginary component of the frequency sub-carriers X[k]=jB[k], where B[k] is the M- PAM symbol. An inverse fast Fourier transform (IFFT) is applied on the frequency domain data symbols and the result is then called the time-domain PAM-DMT waveform. A Hermitian symmetry is applied on the input PAM frequency domain symbols to restrict the timed-domain output to be real- valued. This is done as follows:
(1)
where k=1, 2, …, NFFT-1, X[0]=0, and X[NFFT/2]=0. Similar to other OFDM techniques, the last NCP symbols are appended at the start of each PAM-DMT frame to ensure that single-tap equalizer can be used to equalize the communication channel. The time-domain PAM-DMT waveform can be given as follows:
(2)
Based on Hermitian symmetry, this can be give as
follows:
(3)
The antisymmetry of PAM-DMT can be shown in (1), as follows xPAM[n]=-xPAM[N-n], as shown in Fig.3(a). The positive samples from the first half of waveform are repeated as negative samples in a reverse order at the second half of the waveform, whereas the negative samples from the first half of waveform are repeated as positive samples in a reverse order at the second half. This means that the clipping distortion has a Hermitian symmetry and therefore, falls only on the real- valued sub-carriers. Therefore, clipping the time-domain at zero-level does not distort the data symbols on the imaginary component of the sub-carriers. However, a 3 dB SNR penalty affects the demodulator due to clipping the time-domain PAM- DMT signal at zero-level. The spectral efficiency of PAM- DMT modulation scheme can be given as follows:
(4)
This is exactly similar to the spectral efficiency of DCO- OFDM. However, the BER performance of M-PAM-DMT is equivalent to the BER performance of M2-QAM DCO-OFDM. Therefore, the energy efficiency advantage of PAM-DMT quickly disappears as the constellation size increase.
Fig. 2. The block diagram of the receiver of SPAM-DMT. CP denotes cyclic prefix; S/P denotes a serial to parallel conversion. FFT denotes.
Fig. 3. (a) Time-domain frame of a PAM-DMT modulator showing antisymmetry property. (b) Time domain frame of a SPAM-DMT modulator showing symmetry property
Therefore, a solution is needed to achieve energy efficiency at high spectral efficiencies. The concept of SPAM-DMT is like the concept of PAM-DMT. However, instead of loading the QAM symbols on the imaginary components of the subcarriers, the data symbols are loaded on the real components of the sub-carriers, X[k]=A[k], where A[k] is the M-PAM symbol. As a result, the time-domain waveform would have a Hermitian symmetry that is different from the Hermitian symmetry applied on the incoming frequency- domain data symbols. This is essential because both the time- domain and frequency-domain signals are real-valued.
The generation of a time-domain SPAM-DMT waveform starts with a PAM modulator as shown in Fig.1. The data are loaded on the real-valued sub-carriers X[k]=A[k], where A[k] is the M-PAM symbol, and then an IFFT operation is applied on the incoming data symbols. The time-domain SPAM-DMT waveform can be given as follows:
(5)
Based on Hermitian symmetry, this can be given as follows:
(6)
Therefore, based on (2) it can be observed that xSPAM[n]=xSPAM[N-n] as shown in Fig.3(b). The positive samples from the first half of waveform are repeated as positive samples in a reverse order at the second half of the waveform,
Fig. 4. The BER performance of SPAM-DMT as a function of SNR and DC bias for 4-PAM-DMT
whereas the negative samples from the first half of waveform are repeated as negative samples in a reverse order at the second half. This means that the signal is repeated halfway, and it is only sufficient to send half of the time-domain frame to retrieve the data symbols at the receiver, shown in Fig.2. The last N/2 are removed, and the whole operation is repeated twice before a cyclic prefix is added at the start of the waveform. However, the time-domain waveform cannot be clipped at zero-level, as the clipping distortion would distort the data- carrying symbols and impair the successful data recovery. Therefore, a DC bias is required as it is the only method to convert the bipolar SPAM-DMT waveform into a unipolar, similar to DCO-OFDM. However, the spectral efficiency of SPAM-DMT is twice of that in DCO-OFDM, as given below:
(7)
Therefore, it is possible to use a lower constellation size to achieve the same spectral efficiency of DCO-OFDM. For example, 2-SPAM-DMT can achieve the same spectral efficiency as 4-QAM DCO-OFDM. This is expected to allow for a better BER performance of SPAM-DMT as will be shown later in Section III.
The receiver starts by removing the cyclic prefix and then duplicating half of the received time-domain frame by creating a time-domain Hermitian symmetric frame. An FFT is applied on the frame and equalization is achieved using a single-tab frequency domain equalizer. The selection of the DC bias for each of the constellation sizes is performed by trying all the possible DC bias points and comparing the required SNR at BER values below 1×10-4. The threshold of the BER was chosen so that it allows for forward error correction (FEC) to work. Fig.4 and Fig.5 shows the results for the DC bias selection for 4-SPAM-DMT and 16-SPAM-DMT, respectively. An optimal DC bias point for 4-SPAM-DMT and 16-SPAM-
Fig. 5. The BER performance of SPAM-DMT as a function of SNR and DC bias for 16-PAM-DMT
DMT were selected at 7.8 dB and 11.2 dB, respectively. The optimal DC bias points for the rest of the constellation sizes are shown in Table.I.
TABLE I. THE DC BIAS POINTS FOR SPAM-DMT
Modulation order
Spectral efficiency [bits/s/Hz]
Bias
[dB]2-PAM
1
5.3
4-PAM
2
7.8
8-PAM
3
9.4
16-PAM
4
11.2
32-PAM
5
12
-
NUMERICAL RESULTS
A numerical study was conducted to examine the performance of the proposed modulation techniques compared with DCO-OFDM and PAM-DMT at the same spectral efficiency. The comparison is based on an additive white Gaussian noise (AWGN) channel with zero-level and upper clipping to model an ideal optical front-end design of a LiFi channel. A LPF is assumed to model the frequency profile of the LED similar to many studies [10].
The performance of 2-SPAM-DMT is compared with the performance of 4-PAM-DMT and 4-QAM DCO-OFDM at spectral efficiency of 1 bits/s/Hz in Fig.6. It is shown that 2- SPAM-DMT outperform both 4-PAM-DMT and 4-QAM DCO-OFDM by 2.75 dB and 3 dB at a BER of 10-4 respectively. It was shown in Fig.7 that the SNR performance of SPAM-DMT outperforms that of PAM-DMT and DCO- OFDM by 20.3 dB and 3.6 dB, respectively. This is a huge gain in energy efficiency that could be claimed against all unipolar OFDM modulation techniques that are similar to PAM-DMT, such as DCO-OFDM and Flip OFDM [11]. The SNR gain achieved by the proposed technique against DCO- OFDM and PAM-DMT is presented in Table II for the rest of the spectral efficiency values.
Fig. 6. BER performance comparison of SPAM-DMT, PAM-DMT and DCO-OFDM at a spectral efficiency of 1 bits/s/Hz as a function of the SNR.
Fig. 7. BER performance comparison of SPAM-DMT, PAM-DMT and DCO-OFDM at a spectral efficiency of 5 bits/s/Hz as a function of the SNR.
TABLE II. THE GAIN OF THE PROPOSED SPAM-DMT IN COMPARISON WITH DCO-OFDM AND PAM-DMT
Spectral efficiency
Gain [dB]
DCO-OFDM
PAM-DMT
1
3
2.75
2
2.85
5.7
3
3
10.5
4
2.75
15.1
5
3.6
20.3
In addition to the energy efficiency gains that SPAM-DMT can offer against DCO-OFDM and PAM-DMT, there are additional gains from a computational complexity point of view. Given the fact that SPAM-DMT uses a 1-D modulation format which allows for a simplified IFFT and FFT operations. Furthermore, it is also possible to make use of the fact that only half of the OFDM frame is required to be transmitted, the IFFT computational complexity at the transmitter can be given as O(N/4×log2(N/2)). Using the same logic, the computational
complexity at the receiver can be given as O(N/4×log2(N/2)). This is because half of the frame is required at the output of the FFT operation and because 1-D modulation format is being used PAM. These are compared with O(N×log2(N)). and O(N/2×log2(N)). for the transmitter and receiver of DCO- OFDM modulation scheme.
-
CONCLUSIONS
A novel modulation technique is proposed in this paper to improve the spectral and energy efficiency of LiFi modulation techniques. The proposed technique introduces a different type of time-domain symmetry to PAM-DMT which allows for an increase in the spectral efficiency by a factor of 2, given that one half of the signal is only required to be sent. Although, the proposed technique still requires a DC bias to be transmitted through LEDs, the value of the DC bias is much lower to that required for DCO-OFDM. Moreover, SPAM-DMT offers significant gains when compared with unipolar OFDM modulation techniques such as PAM-DMT. A 20 dB gain is achieved at a spectral efficiency of 5 bits/s/Hz when compared with PAM-DMT, and a 3.6 dB gain when compared with DCO-OFDM at the same spectral efficiency. Therefore, the proposed technique requires lower SNR to operate at the same data-rate as DCO-OFDM and PAM-DMT, which allows for a reduced power requirements and increased range of LiFi systems. In addition, the proposed scheme offers a computationally efficient solution that outperforms other conventional modulation techniques such as DCO-OFDM and PAM-DMT. SPAM-DMT can be used for the uplink in LiFi where a computationally efficient solution is required so that the battery of the mobile terminal is preserved, and it can be used for long distance LiFi links where the received optical power is limited.
REFERENCES
-
Cerwall, Patrik and others, "Ericsson Mobility Report June 2020," Ericsson. com, 2020.
-
Yeh, Chien-Hung and Liu, Yen-Liang and Chow, Chi-Wai, "Real-time white-light phosphor-LED visible light communication (VLC) with compact size," Optics express, vol.21, no. 22, pp. 26192-26197, 2013.
-
Haas, Harald and Yin, Liang and Wang, Yunlu and Chen, Cheng, "What is LiFi?," Journal of Lightwave Technology, vol.34, no. 6, pp. 1533- 1544, 2016.
-
Azza Alamir, Hamada Esmaiel, Hany S Hussein, "Efficient Optical MIMOOFDM Channel Estimation Based on Correntropy Compressive Sensing," Wireless Personal Communications, vol. 15, no. 3, pp.1955– 1971, 2020.
-
Pei, Yanrong and Zhu, Shaoxin and Yang, Hua and Zhao, Lixia and Yi, Xiaoyan and Wang, J Junxi and Li, Jinmin and others, "LED modulation characteristics in a visible-light communication system," Optics and Photonics Journal, vol.3, no. 2B, pp. 139–142, 2013.
-
Abdallah, Walid and Boudriga, Noureddine, "LED modulation characteristics in a visible-light communication system," in IEEE 18th International Conference on Transparent Optical Networks, pp. 1–6, 2016.
-
Lee, Sian C. Jeffrey and Randel, Sebastian and Breyer, Florian and Koonen, Antonius M. J., "PAM-DMT for Intensity-Modulated and Direct-Detection Optical Communication Systems," IEEE Photonics Technology Letters, vol. 21, no.23, pp. 1749–1751, 2009.
-
Islim, Mohamed Sufyan and Haas, Harald, "LED modulation characteristics in a visible-light communication system," ZTE communications, vol. 14, no.2, pp. 29–406, 2019.
-
Dissanayake, Sarangi Devasmitha and Armstrong, Jean, "Comparison of ACO-OFDM, DCO-OFDM and ADO-OFDM in IM/DD Systems," Journal of Lightwave Technology, vol. 31, no.7, pp. 1063–1072, 2013.
-
Linnartz, Jean-Paul MG and Deng, Xiong and Alexeev, Anton and Mardanikorani, Shokoufeh, "Wireless communication over an LED channel," IEEE Communications Magazine, vol. 58, no.12, pp. 77–82, 2020.
-
Fernando, Nirmal and Hong, Yi and Viterbo, Emanuele, "Flip-OFDM for optical wireless communications," in IEEE Information Theory Workshop, pp. 5–9, 2011.