
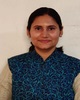
- Open Access
- Authors : Rajshree Charan , Ankit Goad
- Paper ID : IJERTV11IS120152
- Volume & Issue : Volume 11, Issue 12 (December 2022)
- Published (First Online): 05-01-2023
- ISSN (Online) : 2278-0181
- Publisher Name : IJERT
- License:
This work is licensed under a Creative Commons Attribution 4.0 International License
Study on Strength and Behaviour of Concrete Filled Steel Tubular Ractangular Composite Long Columns
Rajshree Charan, Ankit Goad
M.S. University of Baroda
Abstract:- There have been enormous developments in the field of Civil Engineering in the last few decades. One of the spectacular advances made in the field of Civil Engineering is the construction using composite materials.
Steel-concrete composite columns are used extensively in modem buildings; many research works on conventional reinforced concrete column have been carried out in the past. Concrete Filled Steel Tubular (CFST) composite columns however have received limited attention compared to conventional Reinforced Concrete (RC) columns and Steel (S) columns.Concrete filled steel tube columns have been used in bridge piers and multistory buildings. It is now widely accepted that concrete in-filled steel tubes are well suited as compression members in high-rise, long span, heavy loading and seismic resistant structures. Composite structures are more efficient in transferring the load to the foundation. However there arc limitations to its applications mainly due to lack of design guidance.
CFST columns represent a class of structures where the best properties of steel and concrete are used to their maximum advantage. The obvious advantages have led to their widespread use in many countries, and have also attracted many researches to study this structural member. However, a review of the published works has shown widely varying and often contradictory assumptions made by researchers. A design method truly reflecting the strength and behaviour of CFST is not available.
An experimental study is undertaken to monitor the effects of confinement, slenderness, different grades of concrete on CFST composite columns Rectangular Concrete Filled Steel Tubular (RCFST) composite columns. In this study, ultimate strength, load- lateral deflection, load-axial shortening, and stress-strain relationships for CFST column have been developed. Specimens of different types (three in each type and totally eighteen numbers) were cast and tested.
In the present work experimental and analytical investigations are carried out to study the strength and behaviour of CFST composite columns over the entire range of loading in Three phases.
In the first phase, three series of CFST composite columns were cast and tested under different loading conditions by keeping the parameter L/D and M20 & M40 grade of concrete as constant. The study includes application of axial load on the both on steel and concrete. Further tests on CFST Column were carried out to study the confinement effects.
In the second phase, the specimens of CFST columns and RC columns of various slenderness ratios (L/D) were cast and tested. The L/D values selected were 13, 15, 18 Columns of M20 & M40 grade of concrete were used for this stage of investigation.
In the third phase, the study of CFST columns with concretes of different grades was carried out. The grade of concrete was the variable in this study; the grades were M20 and M40. The ratio of L/D was kept constant.
keywords:- Concrete-filled steel tube (CFST) Rectangular column; Slenderness ratio; Axial compressive behavior; Confined concrete; Metal and composite structures; Long Composite Column; Grade Effect.
1.INTRODUCTION
In high-rise structures underground openings are provided for parking purpose in order to overcome land space scarcity. Generally the columns are larger in size and occupy more space. Concrete Filled Steel Tubular (CFST) composite columns represent a class of structure where the best properties of steel and concrete are used to their maximum advantage. When employed under favorable conditions the steel casing confines the core tri-axially and the in-filled concrete inhibits the local buckling of the shell.
Tubular columns have an advantage over the spirally reinforced concrete columns in which the core and the cover behave like two different layers. In the reinforced concrete columns, the cover is inferior to the core and the spiral reinforcement does not come into action until the cover spalls while in the tubular columns the core forms one continuous homogeneous medium. Also in slender tubular columns where buckling will occur before the concrete is confined, the shell will add to the strength while the spiral will not. Tubular columns, when considered as reinforced concrete columns, represent columns with a well dispersed reinforcement. Ties and spirals could be avoided and tubes themselves form the shuttering.
Hence, CFST columns reduce substantially the amount of expensive steel required to support the given load, while the dimensions of the column are smaller than those of a reinforced cement concrete column of the same strength there by increasing the available floor space. CFST columns have become the preferred form for heavy loading conditions, seismic resistant structures subjected to severe flexural load, bridge piers subject to impact from traffic, columns to support large sized storage tanks, decks of railways and columns in multi-storey office buildings.
Until 1950s the steel stanchions were usually encased in low strength concrete primarily to provide fire protection, but the increase in strength afforded by the concrete was not quantified. In modem design of composite columns, the strength and fire resistance of concrete are also taken into account and therefore composite columns provide a more economical structure. Since then, research and application of composite columns have advanced and they have now found wide spread application in the construction industry.
In the mid 1980s several buildings constructed in Seattle in USA became well known for their use of concrete filled steel tubes. Many developed countries like USA, Japan, Germany, Hungary, Singapore, Australia, Canada, Belgium etc., adopt this kind of composite sections to facilitate speedy construction. Because of the increased use of composite columns, many experimental and theoretical works have been carried out to study the strength and behaviour of the CFST columns. Rational methods of designing CFST columns have also been developed.
These columns would be particularly well suited as pre-cast concrete members with close quality control adopting high strength concrete. Main applications are for stocky columns of small slenderness carrying high compressive load as in bridge piers and piles. CFST also possess increased fire resistance.
Figure 1.1 concrete-filled Steel Rectangular or tubular column
-
EXPERIMENTAL ANALYSIS
-
NEED FOR THE PRESENT WORK
The review of literature presented in the previous chapter on composite columns shows that widely varying and often contradictory assumptions were made by researchers. One of the reasons for the large deviations between theoretical and experimental strengths reported by researchers could be due to an enhanced capacity by confining effect of concrete in the composite columns. The earlier research did not give any clear design method that truly reflected the strength and behavior of axially loaded concrete filled steel tubular columns. The present investigation aims to estimate the ultimate strength and study the behavior of composite columns.
-
SCOPE AND OBJECTIVE OF THE INVESTIGATION
Concrete in-filled tubular column is in a different state of stress compared to conventional reinforced concrete column. In the former type, there is a possibility of the concrete in the core being subjected to lateral confining pressre brought about by the surrounding shell while the design of reinforced concrete column is based on uni-axial stress-strain curve of concrete as mentioned in IS 456-2000, that may not be applicable for concrete filled steel tubular column. Any stress-strain curve that is to be used in the design of CFST must realistically reflect the state of stress in concrete in such columns.
The in-filling of a steel tube by concrete produces certain mechanical interactions that are highly beneficial. The in-filled concrete increases the flexural stiffness of the tube, and thus increases its buckling strength under axial compression. The concrete also effectively prevents or delays local buckling of the tube wall. Further more, the lateral confinement provided by the tube improves the strength and ductility (or deformability) of the concrete. It is highly desirable that full advantage be taken of these effects in developing a design method for such structures.
The main objectives of the present work are summarized as follows:
-
To carry out experimental and analytical investigations to determine the confinement effects, slenderness effects and influence of different grades of concrete on strength and behaviour of concrete filled steel tubular (CFST) composite columns over the entire range of loading.
-
To carry out experimental and analytical investigations on strength and behaviour of fully steel encased concrete filled steel tubular (RCFST) composite columns over the entire range of loading.
The axial load is applied on the specimens to know the ultimate strength and behaviour of columns. Also the experimental ultimate load carrying capacity of the CFST columns and the RC columns are compared.
The investigation is also concerned with the determination of the salient points of the curves such as load-lateral deformation, load-axial shortening and The experimental investigation consists of Three phases as follows,
-
Phase I – Study on confinement effects
In this phase the structural behaviour of confined concrete is studied by keeping the parameter L/D and M20 grade of concrete as constant for CFST composite column. Study has been made to observe the effect of confinement in Rectangle CFST columns when the axial load was applied on the concrete core only, both on steel and concrete and on the steel tube only.
-
Phase II – Study on slenderness effects
In the second phase, the specimens of CFST columns and RC columns of various slenderness ratios (L/D) are cast and tested. The L/D values selected are 13,15 and 18. Columns of M20 & M40 grade of concrete are used for this stage of investigation.
-
Phase III – Study on concretes of different grades
Concrete grades are varied and investigated in this phase. The grades used are M20 & M40 for Rectangle CFST.
3.1 MATERIALS (i). Cement
-
-
-
EXPERIMENTAL INVESTIGATION
Ordinary Portland cement 53 conforming to IS: 269-1976 had been used for concreting. The cement used for casting of specimens was fresh, free of lumps and purchased from a single source. It was stored under dry conditions.Standard Consistency 32%.Initial Setting time 75min.
-
Fine aggregate
The most common fine aggregate used in this study was river sand. Uniformly graded sand available near Karur was procured and used. It was clean, free from organic matter, silt and clay.Sieve Analysis-zone2 F.M. for Fine Aggregate=3.3 The Specific gravity of fine aggregate is = 2.98
-
Coarse aggregate
Coarse aggregates are inert materials used for increasing the volume and strength of concrete. Graded, crushed hard blue granite jelly of 10 mm size and confirming to IS 383-1963 available in and around Coimbatore was used. They are free from adherent coatings, coal residues, clay lumps. They were not containing organic or other impurities.
F.M. For Course Aggregate=5.63
(iv).Water
Potable water available in the campus had been used for concreting as well as curing the specimens. The water used for both mixing and curing is free from injurious amount of oils, acids, alkalis, salts organic materials or other substances.
-
Steel tube
Mild steel hot-finished seamless tubes were used. The thickness of the tube was 4.8mm measured by a screw-gauge at four places and the mean value was taken. Using a vernier caliper, the diameter of the tube in three different directions was measured and the mean value was calculated. The steel tubes had an average yield stress of 310 N/mm. All the steel tubes were machined at their ends, and concrete was poured so that a recess of 12mm was available at both the ends. Machined cap plates were placed at both ends to transfer load through this loading piece. The depression of 12mm was ensured that in this condition, even at failure, loading head did not come to bear on concrete. All structural steels used conformed to IS: 4923, IS: 1239
-
Concrete
Concrete strengths are specified in terms of the characteristic average cube strengths, measured at 28 days is M20 and M40 are measured. the properties of different grades of concrete used according to IS: 456-2000 and the corresponding Ec values.Slump for the given sample= 100 mm
-
-
MIXING ,PLACING AND CURING OF CONCRETE
Cement, fine aggregate and coarse aggregate were weighed and batched as per the required quantity, obtained from the mix design An electrically operated concrete mixer was used for mixing the concrete. The materials are mixed in a mechanical mixer of revolving drum type. Minimum mixing time of 90 seconds was maintained. This period has been counted after all the materials are in the drum. The main purpose of mixing is to produce an intimate mixture of cement, water, fine and coarse aggregate of uniform consistency throughout each batch.
Conveying of concrete from the mixer to the mould has been done manually. For composite columns the empty steel pipe was kept ready for placing of concrete. The tubes were filled in a vertical position. Concrete was poured in to the steel tube from top. The tube was placed on a smooth surface before concreting. The concrete was compacted by hammer impact vibration on around CFST column. The compaction by tamping rod is used for adopting high water cement ratio to enable the concrete to flow readily around the reinforcement. The compaction by vibrator was used for all mixes. Such compaction prevents honeycombing, ensures more impermeable and dense concrete, better bond between concrete and reinforcement and a better finish. After filling the tube the concrete was finished smooth at the top.Cubes of size 150 mm x 150 mm x 150 mm was cast as companion specimen for all mixes. After 24 hours, the sides of mould were removed from the specimens. The companion specimens were also removed from the mould. Curing was done by immersing the specimens in the curing tank for 28 days from the date of casting. The companion specimens were also cured under similar conditions and period. The purpose of curing is to prevent the loss of moisture in concrete due to evaporation thereby preventing the development of high temperature gradient within the concrete.
-
CASTING OF SPECIMENS
Rectangular steel tubes are filled with concrete were casted by fixing them at the bottom verticaly then concrete was pored in side the tubes. tamping was done at every fill and vibrations are provided using hammer on over all surface After the curing period, the specimens were taken out from curing tank and dried. The specimen was cleaned. Reference points were marked on the specimen for fixing the compressometer. In the middle height of each specimen four glass pieces were fixed at each quarter perimeter of the specimens. This was done for fixing the LVDT. The DEMEC points for taking strain gauge readings were fixed over the specimen and glass pieces were fixed at deflectometer placing points.
-
Effect of confinement
The experimental investigations consist of 3 stages. In the first stage the structural behaviour of confined concrete was studied by keeping the parameter L/D as constant for steel tubes. CFST column specimens size 96mm x 48mm thickness 4.8 mm and Different length using M20 & M40 grade concrete were used of a total of 9 numbers were cast for each grade with different slenderness ratio to study the confinement effects.
(ii).Slenderness effects
The various slenderness (L/D) ratios used to cast the specimens were 13,15 & 18 Thickness of 4.8mm steel tubes was kept constant for all the specimens. Three specimens for each L/D ratio of CFST were cast. Three specimens of reinforced concrete columns having diameter equal to CFST columns for each L/D ratio were also cast and tested.
The CFST composite columns are found to take more buckling loads due to the confinement of concrete. In CFST composite column the applied load is to be shared by both steel tube and concrete. Maximum buckling usually takes place in the middle regions. Composit colum Cast And Tested. Concrete of grade M20 & M40 was used in this study.
Table 5.1 Steel Tube of Different Grade, LENGTH, Slenderness Ratio FOR RECTANGULAR TUBES
Sr. No.
Size of Tube (mm)
Thickness (mm)
L/D Ratio
Length (mm)
No of Specimen
Gade of Concrete
1
96*48
4.8
13(R1)
624.00
3
M-20
2
96*48
4.8
15(R2)
720.00
3
M-20
3
96*48
4.8
18(R3)
864.00
3
M-20
1
96*48
4.8
13(R1)
624.00
3
M-40
2
96*48
4.8
15(R2)
720.00
3
M-40
3
96*48
4.8
18(R3)
864.00
3
M-40
-
-
EXPERIMENTAL SET-UP :
The column was tested for static, monotonic axial load with the following arrangements, using double-acting hydraulic push-pull jacks. The schematic diagram and complete test set up used for all the specimens are shown in Figs. respectively. The test set-up consists of the following:
-
loading arrangements
-
Instrumentation for measuring deflections
-
The arrangement for taking measurement for strains in steel and concrete
-
Loading Arrangement :
The specimens were instrumented to measure longitudinal strain in steel and concrete and hoop strain in steel. Mild steel pillcts were fixed on the tubes, to measure the longitudinal strain.
The specimens were held vertically between two solid plates to simulate semi rigid end conditions in the real structure. The load cell was placed in between column head and jack. The column head has a special arrangement for the effective load transfer to the column. The column head was changed as per the loading conditions. Using double action push-pull jack of capacity 1000 kN, axial load was applied directly on the load cell and load was measured from load cell meter. Hand operated oil pumps were used for the application of load through jack.
-
Deflection measurement
Lateral deflections were measured at top, bottom one third and also at mid height of the column using Linear Variable Displacement Transducers (LVDT) which indicate the displacement digitally. Longitudinal deflections of the columns were measured using deflectometer with a least count of 0.01 mm. Connecting rod was fixed between the column head and base plate as shown Fig as a special arrangement to measure axial shortening. The deflectometer was attached with the connecting rod and placed above the base plate.
The lateral deflection and axial shortening as well as the deflections were measured along periphery of the casing and at the above mentioned locations for each increment of the loading.
-
Strain measurement
The strains developed on the surface of the steel casing were measured at top, and bottom using DEMEC gauges with a least count 0.02 mm over a gauge length of 100 mm .
In the mid height of the specimen, compressometer was used to determine the strain and deformation characteristics of columns. Due to the pivot, the compression readings are magnified twice and to get the actual deformation of the specimen, the observed readings of the dial gauge are to be divided by two.
-
-
TESTING PROCEDURE
The testing procedures for all the columns were same. The column was placed on the base of the platform and properly centered for loading axially. Before commencing the test, all the defleetometer and strain gauges were checked and the initial measurements at strain measuring points were taken. In each test, initially the column was loaded with small load increment (0.5 kN) and then released to check the effectiveness of the instrument set-up and loading.
The axial load was applied by means of manually operated hydraulic jack and loads were measured for each increment with the help of the proving ring. Then the specimens were subjected to a process of monotonic axial loading up to the maximum
load. For each increment of loading, the following observations were made and recorded up to ultimate load stage.
-
Proving ring readings
-
Defleetometer readings
-
Strain measurement
-
Axial shortening
The buckling and linear strains at all points have more or less uniformly increased up to the formation of first crack. As the load was further increased after formation of first crack, the rate of increasing of buckling was high. The specimens were loaded at constant rate and test was carried out even after the ultimate stage was reached, and the specimens behaviour was observed.
The loading on thecolumn was continued till the final collapse of the specimens. After that, the load increments were controlled depending upon the visible deflection capacity of the column.
(i) Testing of Companion Specimens
The concrete cubes were tested as per IS: 516-1964. Companion specimens viz., cubes of size 150 mm x 150 mm x 150 mm were cast and tested for compressive strength. to obtain the stress strain diagram for concrete. This diagram was used to find modulus of elasticity of concrete.
Fig 7.1 Test set up in plan
Vol. 11 Issue 12, December 2022
Fig 7.2 Test set up in Elevation
-
RESULTS AND DISCUSSIONS
In this chapter, the experimental behavior and mode of failure of columns are discussed, and also the experimental ultimate loads are computed. The columns were tested under axial loading and their behavior at different stages of loading was observed. All the column specimens were loaded up to failure and corresponding deflections and strains were recorded.
Specimens of different types were cast and tested. The test parameters include.
-
Influence of confinement effects
-
Influence of slenderness effects
-
Influence of concretes of different grades
The behavior and type of failure of composite columns for the above mentioned parameters and that of ordinary reinforced concrete columns are discussed below.
-
INFLUENCE OF CONFINEMENT EFFECTS
Axial compressive strain of stel tube was measured using a compressometer at the mid-height of specimen. A special arrangement was used and a dial gauge was attached to measure axial shortening of column.
The structural action of short columns may be described as follows. At the initial stage of loading and before the start of cracking in core concrete, the steel tube has no restraining effect on the concrete core, which carries the load as an ordinary longitudinal reinforcement (loading cases B and C). As the longitudinal strain of core concrete increases, micro cracks develop in concrete. The concrete bears out against the steel tube, which applies a confining reaction to the concrete. A radial pressure develops at the steelconcrete interface setting up hoop tension in the tube. Due to friction at the steel-concrete interface,
Vol. 11 Issue 12, December 2022
longitudinal compression is also induced in the steel tube (loading case A). At this stage and later, in all loading cases, the concrete core is stressed tri-axially and the steel tube bi-axially.
When steel yields, there is a transfer of load from the tube to the core, because the longitudinal stress in steel is decreasing with increasing hoop tensile stress; thus, the strength of concrete core is continuously enhanced. Finally, failure of the column occurs when the resultant compressive force carried by the steel tube and the concrete core reaches the ultimate. The tests showed that the wrinkling and bulging of the steel tube under load in both loading cases A and B. The tests showed that the concrete filled steel tube columns behaved in a ductile manner.
A typical load-axial strain curve of CFST columns shown in Fig.6.1 at the initial stage of CFST loading up to certain portion is practically a straight line with a slight change in slope at point a. At the load indicated by point b, the first slip lines were observed on the surface of the steel tube. After this, the load-strain curve diverged significantly from its initial linearity. At point c where dP/de = 0, the load reaches its maximum value. The point a denotes the load at which cracking in concrete takes place.
Point b denotes the first yield of steel and point c denotes the failure of the column.
Fig 8.1 Solid cylinder and thin shell under different stresses
Table 8.1 Experimental ultimate load for confinement effects
SI.
No.
Description of Specimens
Column ID
Exp. Ultimate Load of Specimen (kN)
Average Ultimate Load (kN)
Observation at Failure
1
CFST of fully loaded
R1-a(13)
67
Small buckling at center
2
R1-b(13)
66
66.33
Small buckling at center
3
R1-c(13)
66
Small buckling at center
80
70
60
50
40
30
20
10
0
1 2 3 4 5 6 7 8 9 10 11 12 13 14 15
R1 Rectangular CFST Column M40 Load
R1 Rectangular CFST Column M40 axial- R1-a
R1 Rectangular CFST Column M40 Axial- R1-b
R1 Rectangular CFST Column M40 Axial- R1-c
ISSN: 2278-0181
Vol. 11 Issue 12, December 2022
-10
70
60
50
40
30
20
10
Fig 8.2 Load vs Axial shortening of CFST for different loading conditions
R1 Rectangular CFST
Column M40 Load
R1 Rectangular CFST
Column M40 Latera- R1-a
R1 Rectangular CFST Column M40 Lateral- R1-b
0
1 2 3 4 5 6 7 8 9 10 11 12 13 14 15
Fig 8.3 Load vs Lateral deformation of CFST for different loading conditions
-10
-
INFLUENCE OF SLENDERNESS EFFECTS
It is observed from the tests that the failure modes of concrete-filled steel tube columns depend on slenderness ratio. When the slenderness ratio is very low, the columns fail due to yielding of steel and crushing of concrete under direct compression. When the slenderness ratio is large, the columns fail by elastic buckling and Eulers formula is applicable.
the deflections increased considerably for L/D ratio of 13 and the specimens failed due to excessive buckling. The load Vs lateral deflection, load Vs Axial shortening, load Vs Axial strain and Stress Vs Strain curves for composite columns with L/D ratios of 13,15 and 18 are shown in Figs.
Table 8.2 Experimental Ultimate Load For Concretes Of Different Slenderness Ratio
Description of Specimens
Column ID
Exp. Ultimate Load of Specimen (kN)
Average Ultimate Load
(kN)
Observation at Failure
CFST for L/D= 13
R1-a
61
64.1667
Small Buckling at center
R1-b
65.5
Small Buckling at center
R1-c
66
Small Buckling at center
CFST for L/D= 15
R2-a
65
65.1667
Medium Buckling at center
R2-b
65
Medium Buckling at center
R2-c
65.5
Medium Buckling at center
CFST for L/D= 18
R3-a
56
59.833
Larg Buckling at center
R3-b
58.5
Larg Buckling at center
R3-c
65
Larg Buckling at center
70
60
50
40
30
20
10
0
Rectangular Column
M20 Load
Rectangular Column M20 Axial-R1 L/D=13
Rectangular Column M20 Axial-R2 L/D=15
Rectangular Column M20 Axial-R3 L/D=18
1 2 3 4 5 6 7 8 9 10 11 12 13 14 15 16 17
Fig 8.4 Load Vs Axial shortening of CFST for different L/D ratio
-
INFLUENCE OF CONCRETES OF DIFFERENT GRADES
Failure of the specimen occurs due to the crushing of the core concrete and the compressive yielding or local buckling of the steel tube. Crushing of concrete occurs at the top or middle or bottom of the specimen. Whenever the grade of concrete was increased, the load carrying capacity of the composite columns also increased. The ultimate load carrying capacities of each type of specimen for different concrete grades are listed in the Table and The load Vs lateral deflection, load Vs axial shortening, load Vs axial strain and stress Vs strain graphs are shown in the Figs.
The ultimate load carrying capacities of each type of specimen, for different concrete grades are listed in the Table.
Also the load Vs deflection and stress Vs strain curves are shown in the Figs..
Table 8.3 Experimental ultimate load for concretes of different grades
SI.
No.
Description of Specimens
Column ID
Exp. Ultimate Load of Specimen (kN)
Average Ultimate Load (kN)
Observation at Failure
1
CFST for M40
R3-a
66
68.33
Larg Buckling at center
2
R3-b
73
3
R3-c
66
1
CFST for M20
R3-a
56
59.83
Larg Buckling at Center as Compare to M40
2
R3-b
58.5
3
R3-c
65
80
70
60
50
40
30
20
10
R3 Rectangular CFST
Column for Different Grade Load
R3 Rectangular CFST Column for Different Grade Axial R3-M40
R3 Rectangular CFST Column for Different Grade Axial R3-M20
0
1 2 3 4 5 6 7 8 9 10 11 12 13 14 15 16 17
Fig 8.5 Load Vs Deflection of CFST for concretes of different grade
-
-
CONCLUSIONS AND SUGGESTIONS FOR FUTURE WORK
-
-
GENERAL
The experimental results are discussed in chapter 6. This chapter presents the effects of the following parameters on concrete filled steel tubular composite columns based on Experimental investigation.
-
Confinement effect
-
Slenderness effect
-
Concretes of different grades
The conclusions arrived about the above parameters are presented in this chapter. Recommendations and scope for future study are also presented.
-
-
CONCLUSIONS
1. Confinement effects
The conclusions drawn based on the experimental investigation carried out to study confinement effects of Rectangal CFST composite columns are discussed below.
-
Fully loaded CFST column carries 2.45 (245%) times the load carried by the composite steel only loaded case.
-
Ultimate load capacity of composite core loaded CFST column is higher than that of the specimen which is loaded through the steel tube and concrete together.
-
The composite core loaded specimen has adequate ductility. The elephant foot buckling is avoided when load is applied through the core.
-
Ultimate load capacity of steel filled with concrete loaded composite column is 1.28 (128%) times that of hollow steel tube loaded column. In such situations concrete can be filled so that local buckling can be avoided.
-
Both steel and concrete loaded column has 1.38 (138%) times the load capacity of plain concrete and hollow steel tube individually loaded cases.
-
For composite column with concrete filled circular sections, the confinement effect of concrete increases the resistance to axial load.
-
Observations of tests on laterally confined concrete are applicable to concrete in the core of CFST, in a qualitative manner. The lateral confinement supplied by the shell of CFST, progressively increases with the load level and is also
continuously applied.
-
Slenderness effects
The following conclusions are arrived from the experimental test results and observations:
-
The buckling failure can be avoided and the load carrying capacity can be increased by lowering the slenderness ratio for CFST and RC columns.
-
The ultimate axial compressive failure load of the CFST composite columns was 363 percent more when compared with the ultimate axial compressive failure load of the conventional RC columns of same size.
-
It was observed from the tests, that the failure mode of the CFST composite column depends on slenderness ratio. When the slenderness ratio is very less, the column fails due to yielding of steel and crushing of concrete under direct compression. When the slenderness ratio is large, the column fails by elastic buckling.
-
The deflection is low, where L/D ratio is 13 The deflection rate considerably increased when L/D ratio is 18 compared with the ratios of 15 respectively.
-
-
Concretes of different grades
The following conclusions are arrived from the experimental investigations:
-
Generally, when the grade of concrete was increased, the load carrying capacity of both the composite columns and ordinary reinforced concrete columns were increased. Comparing the experimental results of composite columns with the ordinary reinforced concrete columns, the load carrying capacity is 300% higher.It is due to the confinement effect of steel shell.
-
The steel confinement prevents the crushing of the concrete. The percentage increase of load carrying capacity of composite columns compared to that of the ordinary reinforced concrete columns.
-
-
General conclusions
-
The strains and deformations of CFST composite columns were found to be more than those of the conventional RC columns of same size. Hence, these composite columns are more ductile than RC columns. Therefore, it is concluded that the CFST composite columns are most suitable in places like where seismic forces are large.
-
The ultimate axial failure load of CFST composite columns were found to be more. Hence, the Euro code 4 underestimates the ultimate axial load carrying capacity of
-
CFST composite columns.
-
-
The material models used for steel tube and in-filled concrete columns predict the failure load, load-lateral deflection, load-axial shortening and stress-strain response up to service load with higher confidence.
-
A study of bi-axially stressed steel shell had shown that shell, when supplying confining pressure on the core, is stressed in the lateral direction and hence cannot sustain its full compliment of longitudinal load. However, taking into account the enhanced strength of the laterally confined core, it was decided that the loss in longitudinal load carrying capacity of shell can be ignored without loss of accuracy. Hence a perfect elastic-plastic relationship for steel is acceptable.
-
Classical approach of lateral strain compatibility does not exist in CFST. In the initial stages of loading (ie. up to 50% of ultimate load) the CFST behaves like a member with two independent constituent materials.
-
Comparing the behaviour of reinforced concrete columns and concrete filled steel tubular columns strain compatibility and complete interaction between steel and concrete are present over the entire range of loading in the former, while, strain compatibility and composite action are absent up to certain load level in the later. Consequently, the core of CFST is laterally confined at advanced stages of loading only and at the earlier stages of loading it is under a uniaxial state of stress.
-
-
SUGGESTIONS FOR FUTURE STUDY
-
The study of confinement effect can be extended to other L/D ratios the behaviour can also be studied for different support conditions.
-
The study can also be extended to filled concrete tubes with very highly ductile materials like FRP jackets, polyester cylinder etc.
-
Experimental investigations can also be carried out on eccentrically loaded composite columns.
-
In the present investigation to study the influence of confinement of concrete M25 grade of concrete alone has been used. The study can also be extended to cover other higher grades of concrete.
-
Similarly the investigation on the effects of slenderness ratios can be carried out for higher concrete strengths.
-
The study can be extended to cover the composite columns using suitable steelconcrete bonding agents and shear connectors.
-
The effect of the thickness of the steel shell on the behaviour of composite columns can be studied by changing the D/t ratios keeping D constant.
-
The study can also be extended to cyclic loading and transverse loading.
8. The length of steel encasement of concrete can be varied and the optimal length of encasement can be determined for practical application.
10.REFERENCES
[1] Ansouria,P., Connections to concrete filled tube columns [2] Bidyut Dutta and Bhattacharyya,S.K, An experimental study on the behaviour of concrete-filled light gauge steel tubular columns, The Indian Concrete Journal.. [3] Furlong,R.W., Concrete filled steel tubes, Journal of Structural Division, ASCE [4] Gardner,N.J., and Jacobson. Structural behaviour of concrete filled steel tubes, ACI, Volume-67, No.7[5] Hong Mei, Panos D.Kiousis, Mohammad R.Ehsani, and Hamid Saadatmanesh, "Confinement effects on high-strength concrete, ACI Structural Journal
[6] I.S.1786-1985, "Indian standard cold twisted steel bars", Bureau of Indian Standards [7] I.S.456-2000, Indian standard code of practice for plain and reinforced concrete", Bureau of Indian Standards [8] I.S.516-1964, "Indian standard methods of tests for strength of concrete", Indian Standards Institutions [9] Kvedaras,D.Mykolaitis and SapaIas,A, "Projects in hollow concrete-filled steel tubular structures", Tubular Structures VII, Farkas & Jarmai (eds)- 1996, Balkema [10] Lillistone,D. and Jolly,C.K., "An innovative form of reinforcement for concrete column using advanced composites", The Structural Engineer,Volume-78
[11] Neogi,P.K., Sen H.K and Chapman J.C., Concrete-filled tubular steel columns under eccentric loading, The Structural Engineer [12] Pierre Rochette and Pierre Labossiere, "Axial testing of rectangular column models confined with composites, Journal of Composites for Construction, Volume-4 [13] Roik,K and Bergmann,R., Design method for composite columns with unsymmetrical cross-sections, Journal of Constructional Steel Research [14] Shams,M and Saadeghvaziri,M.A, "Nonlinear response of concrete-filled steel tubular columns under axial loading, ACI Structural Journal [15] Shanmugam,N.E and Lakshmi,B, "State of the art report on steel-concrete composite columns, Journal of Constructional Steel Research [16] Shakir-Khalil,H and Zeghiche,J., Experimental behaviour of concrete-filled rolled rectangular hollow section columns. The Structural Engineer [17] Shakir-Khalil,H and Mouli,M., Further tests on concrete-filled rectangular hollow section columns, The Structural Engineer [18] Shakir-Khalil,H and Al-Rawdan,A., Composite construction in steel and Concrete [19] Stevens, R.F., Encased stanchions, Structural Engineers London [20] Swamidurai, A and Ramajayam L., Behaviour of concrete in-filled tubular column stiffened with weld-mesh, Journal of Structural Engineering [21] Uy,B.Patil S.B., Concrete-filled high strength steel box columns for tall buildings: behaviour and design. The Structural Design of Tall Buildings [22] Yi Zheng, Tsutomu Usami and Hanbin Ge, "Ductility of thin-walled steel box stub-columns, Journal of Structural Engineering [23] Zhong Shan-Tong and Miao Ruo Yu, "Stress-strain relationship and strength of concrete filled tubes, proceedings of an Engineering Foundation Conference on Composite Construction in Steel and Concrete [24] Guiax and Janss., Confinement of concrete filled tubes, Report CRIF, Brussels [25] Basu,A.K and Sommerville,W., Derivation of formulae for the design of rectangular composite columns, Proc. Institution of Civil Engineers [26] He Ruoquan, "Behaviour of long concrete filled steel columns, proceedings of an Engineering Foundation Conference, New York [27] Prion,H.G.L., Boehme,J., Beam-column behaviour of steel tubes filled with high-strength concrete, Fourth International Colloquium, SSRC. New York [28] Rangan,B.V and Joyce,ML, Strength of eccentrically loaded slender steel tubular columns filled with high strength concrete, ACI Structural Journal [29] O'Brien,A.D., Rangan,B.V., Tests on slender tubular steel columns filled with high-strength concrete, Australian Civil Engineering Transactions