
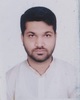
- Open Access
- Authors : Uzair Khan , Dr. Arif Khan
- Paper ID : IJERTV9IS120200
- Volume & Issue : Volume 09, Issue 12 (December 2020)
- Published (First Online): 30-12-2020
- ISSN (Online) : 2278-0181
- Publisher Name : IJERT
- License:
This work is licensed under a Creative Commons Attribution 4.0 International License
Safe Water Technologies
Uzair Khan*
Environmental Engineering Department; Nuva College of Engineering & Technology Nagpur, India
Dr. Arif Khan**
Environmental Engineering Department ; Nuva College of Engineering & Technology Nagpur, India
Abstract- Water everywhere is increasingly being contaminated from numerous sources and this, especially in drinking waters is a major concern, due to the associated health hazards. The most common contaminants being suspended matter, mud, silt, algae, bacteria and other pathogens. Besides large bulk processing systems for industry, Safe Water in India also has special focus on small and medium water supply needs. Solutions are offered for treatment of Municipal Waters, Surface Waters, Open and Tube Well Waters including source waters from streams, rivers, ponds and lakes for drinking water for Residences, Housing Colonies, Resorts, Villages, and Small Towns. Today, more than 1.1 billion people lack access to safe drinking water and 2.6 billion people have no access to basic sanitation. In most industrialized countries, access to safe drinking water is nearly universal, and is often taken for granted. This access has come as the result of massive public expenditures, though now more and more water supply agencies are being privatized. In contrast, access is typically lower in developing countries, where economies are weaker, and infrastructure is not as developed. The situation is the worst for the poorest people in developing countries, who often have no access to piped water supply. These families typically spend one fifth of their income on pure water. In this paper I want to emphasize some Safe Water Treatment Technologies which is used in various house hold and Industries viz. Ultraviolet Water Treatment Systems, Specialty Potable and Non-Potable Water Treatment Systems , Commercial Water Treatment Equipment and Components etc are some of the Technologies to be covered in this paper .
Keywords- Water, Treatment, Hazard, Surface Water, Municipal Water, Technology
-
INTRODUCTION
Water is one of the earth's most precious resources. Though 70% of Earth's surface is water a major 97.5% of this is salt water and only 2.5% is freshwater. Moreover, less than 1% out of this 2.5% amount of freshwater is accessible (the majority is frozen in ice caps or as soil moisture) [Globalchange]. With growing population this amount of water is becoming insufficient. In 2000, 0.5 billion people in the world were chronically short of fresh water out of a total population of six billion [water.org]. As per some estimates, half of the population in the world may have to live without access to their minimum requirement for water by the year 2050. Moreover, India and other developing countries are the worst affected by fresh water crisis mainly because of comparatively lack of better planning to manage their respective fresh water reserves both on the surface and in the aquifers. With a population of above 120 crores, fresh water shortage in India is becoming more and more acute. The per capita water availability in India during the period 1951 2001 has declined from 5177 litres to 1820
litres per year and it would further decrease to 1140 litres by 2050".[economic times]
Three main sources of water can be considered as substitutes for contaminated water: groundwater, rainwater, and surface water. Much has been written about these sources, so this chapter offers only a brief review, with key references.· Groundwater is largely free from harmful bacteria and fecal contamination, though a poorly designed or constructed well can become contaminated from surface water. To prevent this, wells should be grouted around the borehole, and finished at the surface with a concrete platform, with good drainage away from the well. Most commonly, groundwater is reached through boreholes, drilled either by hand or machine. When drilling in arsenic- affected areas, precautions should be taken to make sure that safe aquifers do not become contaminated. If
a borehole must penetrate a contaminated aquifer to reach safe water below, the borehole should be grouted after drilling is completed. In some areas, groundwater can have naturally occurring water quality problems aside from arsenic, such as high levels of iron, manganese, nitrate, chloride, or fluoride. Before promoting new sources of groundwater, the chemical quality should be tested in laboratories. · Rainwater is free from arsenic, and if properly collected, can provide a safe drinking water source. Bacterial contamination is a concern, but this can be minimized by collecting rain from a roof (galvanized metal makes a good collection surface). Tree branches should not overhang the roof, and the roof should periodically be cleaned. Water can be collected through gutters, and piped into a storage tank. Tanks can be built of many materials, but ferrocement (cement with wire reinforcement) is strong and inexpensive, and also can keep the water pH near neutral. When rain first begins to fall, especially at the end of a dry season, roof water should be allowed to run off for 10-15 minutes before collection, to clean the roof. Close to urban areas, and when metal roofs are used, collected rainwater can contain unsafe levels of lead and zinc, and possibly other metals. Typically, collected rainwater contains low levels of bacteria (fecal and total coliform counts average 5-15 and 25-75 per 100 ml, respectively). Water quality testing should be done to ensure that collected water meets relevant standards. In some cases, rainwater may be the safest source of drinking water available, even if low levels of bacteria are present. Often, rainfall is seasonal, and large storage tanks would be required to bridge the dry season. While water can be safely stored for long periods, the large tanks may be too expensive. In such cases, small storage tanks provide an inexpensive and convenient water source during the rainy season, and other sources should be found for the dry
season. · Surface water requires more treatment than groundwater or rainwater, since it usually has very high bacterial contamination. In order to ensure that treatment is always effective, important to include multiple barriers to contamination. The most effective treatment appropriate at the rural, community level, is slow sand filtration, followed by a safety dose of chlorine. In slow sand filtration, surface water passes through prefilters, and is then filtered through 80-100 cm of sand. A bio-layer develops near the surface of the sand, which can effectively destroy most pathogens. Operation of the slow sand filter may be improved through pretreatment with bank infiltration, sedimentation or roughing filtration. The use of roughing filters, in particular, permits effective treatment of water containing higher levels of turbidity, color, and pathogens. This multi-stage filtration is a robust and reliable treatment method in rural communities, and for small and medium size municipalities. Slow sand filtration will not efficiently remove arsenic or agricultural chemicals such as pesticides. It is important to test the water quality of the unfiltered water to make sure that arsenic and pesticides are not present. Likewise, for bacteria, the cleaner the source water, the cleaner the treated water will be. Ponds and other surface water sources used for slow sand filtration should be protected: latrines should not be located near the water, and people and animals should not bathe nearby. Slow sand filters must be regularly cleaned, and the top few centimeters of sand in the filter should be scraped off. After cleanin, the filter will need several days to ripen, and treat water effectively. During this ripening period, filtered water should be disinfected before drinking.
-
Rainwater:
Rainwater collection can provide a safe, arsenic-free source of drinking water if weather conditions are appropriate. When properly collected and stored, rainwater can keep a fairly constant water quality for months. In areas with low population density and hard rocks surfaces (rock outcrops or cement), rainwater can be collected from the ground surface by constructing micro dams to channel surface runoff into underground storage tanks. In more densely populated areas, rooftop collection is more common. Corrugated iron roofs are ideally suited for this purpose, though terra cotta or wood tiles, or concrete roofs are also acceptable. Thatched roofs are not appropriate for rainwater collection, as the collected water is high in organic matter. These roofs, however, can be made suitable with the simple use of inexpensive plastic sheeting. Roofs should be cleaned thoroughly at the beginning of every rainy season, regular maintenance is also crucial. Water is collected from the roof surface in gutters, which are connected to a storage tank with a downpipe. In order to minimize the amount of contamination and organic matter in collected water, a fine mesh netting or coarse sand filter should be placed between the downpipe and the storage tank. This will also help to keep insects and small animals out of the tank, and has been shown to improve water quality, and reduce the risk of mosquito breeding. When rain falls after a dry period, water should not be collected
for the first ten to fifteen minutes, in order to clean the collection surface. This first flush can be achieved through use of a bypass valve, or through a variety of simple designs.
A rainwater storage tank should be completely covered, and have a tap or pump for withdrawing water, to prevent contamination from users. If water is stored in open containers, or users dip cups or pitchers in a tank to retrieve water, the stored water can easily become contaminated with fecal pathogens. The amount of storage required will depend on local rainfall patterns, especially on the extent of dry seasons. If rainfall is scarce or absent for several months, large tanks will be required, or alternate sources of drinking water used during the dry season. In rainy periods, small, inexpensive tanks are able to provide adequate storage for most household needs.
-
Groundwater quality:
Where available and of good quality, groundwater is usually the most acceptable source for drinking water supply. Due to the natural filtering of aquifer materials, and long underground retention times, groundwater typically has very little pathogenic contamination, and requires little or no disinfection. In contrast, surface water is often heavily polluted with fecal material as a result of poor sanitation and hygiene practices. Surface water is also more susceptible to chemical contamination from industrial or agricultural runoff, such as heavy metals, pesticides, or nitrate. However, groundwater quality should not be taken for granted. In very shallow aquifers, bacterial contamination is possible, and even likely if the wellhead is poorly protected. In addition to arsenic, other inorganic constituents in groundwater can cause health or aesthetic problem s, notably iron, manganese, nitrate, chloride, and fluoride.
Other contaminants listed in the WHO Guideline Values should be tested for if their presence is suspected, either from human activity or from naturally occurring sources (WHO, 1993). If some existing groundwater supplies are found to be arsenic-free, these will usually be the most preferred water source, at least in the short term. The simple act of testing all of a communitys wells for arsenic is valuable in that it will identify safe, as well as unsafe, wells. People can be encouraged to share safe water resources, though if safe wells are scarce or absent, this may not be feasible. If safe wells are privately owned, the owners may be reluctant to allow others access to the wells, out of concern for privacy or a fear that increased use will result in increased maintenance and replacement costs. In some cultures, women are the main water collectors, but also have limited social mobility, and privacy concerns can make sharing of a household well uncomfortable or unacceptable. Arsenic-free wells might become contaminated over time through a third mechanism: arsenic could initially be present in a stable, solid form in aquifer sediments. If the geochemistry of the pore water, especially the pH and redox potential, should change, arsenic could
become mobilized, and make its way into the abstracted groundwater.
-
Surface Water:
Surface water presents more of a challenge than groundwater or rainwater in that it is usually heavily contaminated with fecal bacteria. Other contaminants may be contributed from agricultural sources, such as nitrates, phosphates, and pesticides. Finally, surface water is more vulnerable to industrial contamination. In urban and peri- urban areas, surface water often receives untreated wastes from industrial factories, untreated solid wastes, and urban runoff. As a result surface waters can have very high biochemical oxygen demands (BOD), and be seriously contaminated with organic compounds and trace metals. Surface water can also have naturally high levels of inorganic compounds, eroded from source rocks upstream, that can cause human health problems.
-
-
Materials And Methods
An environmental scientist or consultant matches the service provider, modify if necessary, with the requirement.
-
Natural Water includes some discussion on hard and soft water. Softening hard water for boiler, cooler, and domestic application is discussed therein. These treatments prepare water so that it is suitable for the applications.
-
Water Biology deals with water and biology. Drinking water is part of making water suitable for living. Thus, this link gives some considerations to drinking water problems.
-
There are many different industry types, and waters from various sources are usually treated before and after their applications. Preapplication treatment and water treatment offer a special opportunity or challenge. Only a general consideration will be given to some industrial processes.
-
General municipal and domestic water treatment converts used water (waste) into environmentally acceptable water or even drinking water. Every urban centre requires such a facility.
Residential Specialty Water Treatment Systems
-
Softener/Filters available with computer metered or clock timer controllers
-
Hard Water Systems
-
Hard Water and Nitrate Systems
-
Hard Water and Tannin Systems
-
Hard Water and Carbon Added Systems
-
-
Soft n` Pure water purification system with a softener and a chemical / bacteria filter
-
Better Life Series patented filters and softeners with integrated UV
-
Nitrate Removal Systems
-
Tannin & Organic Removal Systems
-
Iron & Hydrogen Sulfide Removal Systems
-
Sediment Removal Systems with automatic or manual backwashing
-
Carbon Filter Systems with automatic or manual backwashing
-
Radon Removal Systems
-
Arsenic Removal Systems
-
VOC Reduction Systems
-
MTBE Reduction Systems
-
Hydrogen Peroxide Dosing Systems
Commercial / Industrial Equipment
-
Commercial Sediment Filters with automatic backwashing
-
Commercial Carbon Filters inline and automatic backwashing
-
Specialty Carbon Systems for arsenic, chloramines, radon, VOC, BOD, & MTBE reduction
-
Commercial MetalEase Filters iron, hydrogen slfide, and heavy metal filters with automatic backwashing
-
Industrial Water Softeners and Filters
-
Commercial UV Systems 15 to 50 GPM
-
Commercial / Industrial UV Systems 40 to 1,345 GPM
-
Commercial RO Systems 400 to 18,000 GPD
-
Commercial RO Systems for very high TDS, brackish water, river intake, seawater intrusion, & seawater desalination
WATER FILTRATION COMPONENTS
Residential, Commercial, and Industrial Components
-
Tank Heads and Manual Backwashing Valves
-
Custom Machined Plastic Tank Heads and Manifolds
-
Residential and Light Commercial Filter and Softener Control Valves
-
Commercial Filter and Softener Control Valves
-
Commercial Deionizer Control Valves
-
Industrial Filter and Softener Stagers and Controllers
-
Industrial Diaphragm Valve Kits – pre-designed for all types of systems
-
Fiberglass Wrapped Pressure Tanks with ABS Liners 5 to 24 inch diameter
-
Fiberglass Wrapped Pressure Tanks with Polypro Liners 7 to 13 inch diameter
-
Fiberglass Wrapped Pressure Tanks with Vinylester Liners 24 to 48 inch diameter
-
Fiberglass Wound Pressure Tanks with Polyethylene Liners 6 to 48 inch diameter
-
FRP Molded Pressure Tanks – 6 to 16 inch diameter
-
Steel Pressure Tanks lined and primed
Filtration Media and Ion Exchange Resins
-
FilterEase and FilterEase-HD sediment filtration, lighter than sand, 20 micron particulate filtration
-
MetalEase , MetalEase-AS and MetalEase-61 removes iron, hydrogen sulfide, arsenic, radium, & other heavy metals without chemicals simple backwashing cleans the bed
-
ProActive Coal-Based Carbon proprietary brand of non-viscous, coal-based carbon acid washed available
-
ProActive Coconut Shell Carbon standard 20 x 50 mesh and catalytic 12 x 40 mesh
-
Anthracite sized to any specification
-
KDF for low level chlorine treatment
-
pH Neutralizer to raise the potential hydrogen in water
-
Manganese Greensand Equivalent (MTM) oxidizing agent carrier
Filter Housings and Cartridges
-
Pentair / Plymouth Products Housings and Filter Cartridges (Pentek)
-
Bag Filters and Multi-Cartridge Filter Housings
-
Fiberglass RO Housings
Filter Housings
-
5 and 10 inch Plastic Filter Housings
-
20 inch and Jumbo Plastic Filter Housings
-
Stainless Steel Filter Housings
-
Valve-In-Head Filter Housings
-
Mounting Brackets and Wrenches
Filter Cartridges
-
Sediment
-
Carbon
-
Specialty
-
Inline Filter Cartridges
-
Absolute Rated Filter Cartridges
-
-
-
MTBE Reduction Systems
MTBE (methyl-t-butyl ether) is a member of a group of chemicals commonly known as fuel oxygenates. Oxygenates are added to fuel to increase its oxygen content. MTBE is used in gasoline throughout the United States to reduce carbon monoxide and ozone levels caused by auto emissions. MTBE replaces the use of lead as an octane enhancer since 1979.
Releases of MTBE to ground and surface water can occur through leaking underground storage tanks and pipelines, spills, emissions from marine engines into lakes and
reservoirs, and to some extent from air deposition. MTBE (methyl-tertiary-butyl-ether) is an oxygenate that is added to gasoline throughout the United States to make it burn cleaner. It reduces carbon monoxide and ozone levels caused by auto emissions. However, MTBE can contaminate water supplies during gasoline spills.
-
Major uses and sources in drinking-water:
The major use of MTBE is as a petrol additive, with production and consumption for this purpose increasing markedly in the 1990s in most parts of the developed world. MTBE is added to petrol at levels of up to 15% by volume as an oxygenate to improve combustion and lower exhaust emissions, particularly carbon monoxide emissions. Fugitive emissions from petrol refineries and petrol filling stations are major environmental point sources of MTBE, whereas vehicles themselves emit sufficient MTBE to be a significant source in dense traffic areas. Surface water can be contaminated by petrol spills and by the use of petrol-powered boats, particularly those using two-stroke engines. Spills and leaking storage tanks can cause more serious problems in groundwater. MTBE is seen as a potentially serious long-term threat to drinking- water supplies if it comes to be widely used at high concentrations in petrol, particularly where there is inadequate control on leakage from underground storage tanks.
-
-
TECHNOLOGIES FOR ARSENIC REMOVAL
In some areas, arsenic-contaminated water will be abundant, and arsenic-free sources scarce or polluted with other compounds. In these areas it may be most efficient to remove arsenic from the contaminated water, at least as a short term measure. Many technologies have been developed for the removal of arsenic. Most of the documented experience has been with large municipal treatment plants, but some of the same technologies can be applied at community or household levels. All of the technologies for arsenic removal rely on a few basic chemical processes, which are summarized below:
-
Oxidation/reduction: reactions that reduce (add electrons to) or oxidize (remove electrons from) chemicals, altering their chemical form. These reactions do not remove arsenic from solution, but are often used to optimize other processes.
-
Precipitation: Causing dissolved arsenic to form a low-solubility solid mineral, such as calcium arsenate. This solid can then be removed through sedimentation and filtration. When coagulants are added and form flocs, other dissolved compounds such as arsenic can become insoluble and form solids, this is known as coprecipitation. The solids formed may remain suspended, and require removal through solid/liquid separation processes, typically coagulation and filtration
-
Adsorption and ion exchange: various solid materials, including iron and aluminum hydroxide flocs, have a strong affinity for dissolved arsenic. Arsenic is strongly attracted to sorption sites on the surfaces of these solids, and is effectively removed from solution. Ion exchange can be considered as a special form of adsorption, though it is often considered separately. Ion exchange involves the reversible displacement of an ion adsorbed onto a solid surface by a dissolved ion. Other forms of adsorption involve stronger bonds, and are less easily reversed.
-
Solid/liquid separation: precipitation, co- precipitation, adsorption, and ion exchange all transfer the contaminant from the dissolved to a solid phase. In some cases the solid is large and fixed (e.g. grains of ion exchange resin), and no solid/liquid separation is required. If the solids are formed in situ (through precipitation or coagulation) they must be separated from the water. Gravity settling (also called sedimentation) can accomplish some of this, but filtration is more effective. Most commonly, sand filters are used for this purpose.
-
Physical exclusion: some synthetic membranes are permeable to certain dissolved compounds but exclude others. These membranes can act as a molecular filter to remove dissolved arsenic, along with many other dissolved and particulate compounds.
-
Biological removal processes: bacteria can play an important role in catalyzing many of the above processes. Relatively little is known about the potential for biological removal of arsenic from water.
-
Boiling does not remove arsenic from water.
-
-
VOC Reduction Systems
-
Most VOCs found in the environment result from human activity. When VOCs are spilled or improperly disposed of, a portion will evaporate,
but some will soak into the ground. In soil, VOCs may be carried deeper by rain, water or snow melt and eventually reach the groundwater table. When VOCs migrate underground to nearby wells, they can eventually end up in drinking water supplies. The U.S. Environmental Protection Agency (EPA) estimates that Volatile Organic Chemicals are present in one-fifth of the nation's water supplies. They can enter ground water from a variety of sources. Benzene, for example, may enter ground water from gasoline or oil spills on the ground surface or from leaking underground fuel tanks. Other examples of commonly detected VOCs are dichloromethane (methylene chloride), an industrial solvent; trichloroethylene, used in septic system cleaners; and tetrachloroethylene (perchloroethylene), used in the dry-cleaning industry. Public water systems are required to be monitored on a routine basis for contamination. For private water supplies, however, it is the homeowner's responsibility to regularly have water quality evaluated.
-
VOCs vary considerably in their toxic (or harmful) effects. Drinking water containing high levels of volatile organic compounds (VOCs) may be harmful to human health. Volatile organic compounds may have a variety of harmful health effects. At high levels of exposure, some VOCs may be harmful to the central nervous system, the kidneys or the liver. Some VOC's are known or suspected carcinogens (cancer causers). Current scientific theory on how cancer starts indicates that even a small level of exposure to a carcinogen may cause an equally small level of risk to some people.
-
Water treatment systems are available which can remove or reduce VOCs. Some home filter systems – such as activated carbon filters – can effectively remove VOCs if they are properly installed and maintained. Granular activated carbon (GAC) filters are typically used to reduce VOC levels in home drinking water. Filtration systems may be installed for point-of-use treatment at the faucet, or point-of-entry treatment where water enters the home. Point-of-entry systems are preferred for VOCs because they provide safe water for bathing and laundry, as well as for cooking and drinking. (VOCs may enter the body through skin absorption or through inhalation of water vapor.) It is important to determine exactly which contaminants are present in water before choosing a system. Then, treatment systems should be checked periodically to ensure that they are operating properly.
-
CONCLUSION
Thus, we can say that Safe Water Technologies has to be used protecting atmosphere and water pollution. Safe Water Technologies also protect human for diseases. Government has to supply the pure water for the poorest people in developing countries.
REFERENCES
-
WHO 1993 Guidelines for drinking-water quality: Volume 1, Recommendations. 2nd edition, World Health Organization, Geneva.
-
WHO 1997a Environmental Health Criteria 194: Aluminum, World Health Organization, Geneva. WHO 1997b Guidelines for drinking-water quality: Volume 3, Surveillance and control of community supplies. 2nd edition, World Health Organization, Geneva.
-
WHO 1998 Guidelines for drinking-water quality: Addendum to Volume 2, Health criteria and other supporting information. 2nd edition, World Health Organization, Geneva.
-
Yacoob, M. and Rosensweig, F. 1992 Institutionalizing community management: processes for scaling up. WASH technical report no. 76, Water and Sanitation for Health Project, Arlington, VA. Yaziz, M., Gunting, H., Sapari, N. and Ghazali, A. 1989 Variations in rainwater quality from roof catchments. Water Research, 23(6), 761-765.
-
Walton, J., Tuniz, C., Fink, D., Jacobsen, G. and Wilcox, D. 1995 Uptake of trace amounts of aluminum into the brain from drinking water. Neurotoxicology, 16(1), 187-190.
-
Waypa, J., Elimelech, M. and Hering, J. 1997 Arsenic removal by RO and NF membranes. Journal of the American Water Works Association, 89(10), 102-114.
-
Viessman, W., Jr. and Hammer, M. 1985 Water Supply and Pollution Control. 4th edition, Harper & Row, New York.
-
Viraraghavan, T., Subramanian, K. and Aruldoss, J. 1999 Arsenic in drinking water – Problems and solutions. Water Science and Technology, 40(2), 69-76.
-
WRC 1989 Disinfection of rural and small-community water supplies: manual for design and operation, Water Research Centre, for WHO, Medmenham.
-
Vaishya, R.C. and Agarwal, I.C. 1993 Removal of arsenic(III) from contaminated ground waters by Ganga sand. Journal of Indian Water Works Association, 25(3), 249-253.
-
Subramanian, K.S., Viraraghavan, T., Phommavong, T. and Tanjore, S. 1997 Manganese greensand for removal of arsenic in drinking water. Water Quality Research Journal of Canada, 32(3), 551-561.
-
Thomas, P. and Greene, G. 1993 Rainwater quality from different roof catchments. Water Science and Technology, 28(3-5), 291-299. Trussell, R.R.,
-
Trussell, A. and Kreft, P. 1980 Selenium removal from groundwater using activated alumina. 600/2-80-153, USEPA, Cincinnati, OH.
-
Dutta, A. and Chaudhuri, M. 1991 Removal of arsenic from groundwater by lime softening with powdered coal additive.
Aqua, 41(1), 25-29
-
Edwards, M. 1994 Chemistry of arsenic removal during coagulation and Fe-Mn oxidation. Journal of American Water Works Association, 86(9), 64-78.
-
Dutre, V. and Vandecasteele, C. 1998 Immobilization mechanism of arsenic in waste solidified using cement and lime. Environmental Science and Technology, 32(18), 2782- 2787.
-
Bajpai, S. and Chaudhuri, M. 1999 Removal of arsenic from ground water by manganese dioxide-coated sand. Journal of Environmental Engineering, 125(8), 782-784.
-
Brewster, M.D. 1992 Removing Arsenic from Contaminated Wastewater. Water Environment & Technology, 4(11), 54-57.
-
Cairncross, S. and Feacham, R.G. 1993 Environmental Health Engineering in the Tropics. 2nd edition, John Wiley and Sons Ltd, Bath.
-
Ahmed, M. and Rahman, M.M. 2000 Water Supply and Sanitation – Rural and
-
Low-Income Urban Communities, ITB-Bangladesh Centre for Water Supply
-
and Waste Management, Dhaka, Bangladesh.
-
Bajpai, S. and Chaudhuri, M. 1999 Removal of arsenic from ground water by
-
manganese dioxide-coated sand. Journal of Environmental Engineering,125(8), 782-784.
-
Bellack, E. 1971 Arsenic removal from potable water. Journal of the AmericanWater Works Association, 63(7), 454.
-
Bonnin, D. 1997 Arsenic removal from water utilizing natural zeolites.Proceedings, AWWA Annual Conference. American Water Works Association, Denver, CO.
-
Buswell, A.M. 1943 War problems in analysis and treatment. Journal American Water Works Association, 35(10), 1303.
-
Chen, H.-W., Frey, M.M., Clifford, D., McNeill, L.S. and Edwards, M. 1999
-
Arsenic treatment considerations. Journal of the American Water Works Association, 91(3), 74-85.
-
Cheng, R.C., Liang, S., Wang, H.C. and Beuhler, M.D. 1994 Enhanced coagulation for arsenic removal. Journal of the American Wter Works Association, 86(9), 79-90.
-
Clifford, D. 1986 Removing dissolved inorganic contaminants from water. Environmental Science and Technology, 20, 1072-1080.
-
Clifford, D. 1999 Ion exchange and inorganic adsorption. In:
-
Letterman [Ed.] Water Quality.
-
-
Gupta, S. and Chen, K. 1978 Arsenic removal by adsorption.
J. Water Poll.
-
Johnston and Heijnen : Safe Water Technology for Arsenic Removal Contr. Fed., 50, 493-506.
-
Ramaswami, A., Isleyen, M. and Tawachsupa, S. 2000 Zero- valent iron for treatment of high arsenic water. Proceedings, 4th International Conference on Arsenic Exposure and Health Effects. SEGH, San Diego, CA.
-
Subramanian, K.S., Viraraghavan, T., Phommavong, T. and Tanjore, S. 1997
-
Manganese greensand for removal of arsenic in drinking water. Water Quality Research Journal of Canada, 32(3), 551- 561.
-
Viraraghavan, T., Subramanian, K. and Aruldoss, J. 1999 Arsenic in drinking water – Problems and solutions. Water Science and Technology, 40(2), 69- 76.
-
Waypa, J., Elimelech, M. and Hering, J. 1997 Arsenic removal by RO and NF membranes. Journal of the American Water Works Association, 89(10), 102-114.
-
Letterman, A. [Ed.] 1999 Water quality and treatment: a handbook of community water supplies. American Water Works Association, McGraw-Hill, New York.