
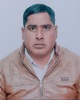
- Open Access
- Authors : Sumit , Dr. S. M. Gupta
- Paper ID : IJERTV10IS090177
- Volume & Issue : Volume 10, Issue 09 (September 2021)
- Published (First Online): 29-09-2021
- ISSN (Online) : 2278-0181
- Publisher Name : IJERT
- License:
This work is licensed under a Creative Commons Attribution 4.0 International License
Performance Based Seismic Evaluation of Multi Storey R.C.C Building
Sumit1
1M.Tech Student (Structural Engineering), Department of Civil Engineering, N.I.T. Kurukshetra, Haryana, India;
Dr. S.M Gupta2
2Professor,
Department of Civil Engineering, N.I.T. Kurukshetra, Haryana, India;
Abstract:- From the recent earthquakes, it is noticed that the seismic risk in urban areas is increasing and the infrastructure facility is far from socioeconomically acceptable levels. Performance based seismic design is an elastic design methodology done on the probable performance of the building under different ground motions. Thus the Performance-based seismic design is a process that permits design of new buildings or upgrade of existing buildings with a realistic understanding of the risk of life, occupancy and economic loss that may occur as a result of future earthquakes. Performance-based design begins with the selection of design criteria stated in the form of one or more performance objectives. In present study an attempt is made to understand the procedure and methodology adopted in performance based seismic evaluation of (G+10) and (G+20) storey RCC building with and without shear wall. Non linear static pushover analysis is performed by using SAP2000, a product of computers and structures international. The results of analysis have been compared in terms of base shear, storey drift, storey displacement and plastic hinge rotations, and found that both buildings satisfy strength as well as drift criteria.
Keywords : Performance Based Seismic Design, Non linear Static Pushover Analysis
INTRODUCTION
Earthquakes is one of the most devastating natural disasters that lead to catastrophic losses of lives and property around the world. According to the existing standard code of practice IS: 1893(part-1)-2002, more than 60% of existing land in india is susceptible to different kinds of earthquakes. Many existing structures are seismically deficient due to lack of awareness regarding seismic behavior of structures. Hence, we need to build structures that are capable to withstanding earthquakes with acceptable damage. The current seismic design codes in India and most of the other countries follow force-based design (FBD) method. Forced based approach does not give an idea about, how a building will perform under seismic loading, whereas displacement based approach is also known as performance-based seismic design (PBSD) evaluates how the building is going to perform under a given seismic loading. The concept of performance based seismic engineering using nonlinear analysis to evaluate the behavior of a building. The most accurate nonlinear analysis is time history analysis, but it is very complex, requires many computations, time and not practical for every design. So from a practical point of view nonlinear static analysis also known as pushover analysis gives acceptable result. Pushover analysis is an incremental static analysis used to determine the force-displacement relationship, or the capacity curve, for a structural element. The analysis involves applying horizontal loads to a computer model of the structure incrementally (i.e. pushing the structure), and plotting the total applied shear force and associated lateral displacement at each increment, until the structure reaches a limit state of collapse condition.
METHODOLOGY
Performance based seismic evaluation of buildings is carried out using pushover analysis. It is a static nonlinear procedure in which the magnitude of the structural loading is incrementally increased. With the monotonic increase in the magnitude of the loading, weak links and the failure modes of the structure are found. As the load and displacement increases, the element (beams, columns, etc.) begin to yield and deform inelastically. Pushover analysis requires capacity curve, demand curve, and performance objective. Capacity curve, which is roof displacement vs. base shear graph, is obtained from the nonlinear static analysis. Capacity curve depends upon stiffness and deformation capacity of the structure. The demand curve is estimated by reducing 5% damped design spectrum by special reduction method. Capacity curve and Demand curve is transformed into Acceleration Displacement Response Spectra (ADRS) which is acceleration vs. displacement graph. The transformed capacity curve is called capacity spectrum. Capacity curve, Demand Curve and ADRS is shown in figure-1.
Figure 1. Capacity Curve, Demand Curve and ADRS.
The Nonlinear properties in beams and columns have to be introduced for analysis which can be introduced using predefined hinge properties in Sap 2000. The flexural default hinges (M3) to the beams at two ends was assigned. The interacting (P-M2- M3) frame hinges type a coupled hinge property was also assigned for all the columns at upper and lower ends. Sap 2000 take auto hinge property from ASCE41-13 tables. All buildings are designed as per IS 456:2000, for adequate main and shear reinforcements, corresponding to the ultimate moment capacity level. Flexural plastic hinges will be developed along with the predicted values of ultimate moment capacity when there is no prior failure in shear. Therefore, there is no need for shear hinge modeling as it is obvious for a code designed building to fail in flexure and not in shear. Two pushover loads Push X and Push Y were defined as displacement controlled. The maximum target displacement of the structure was kept at 4% of the height of the building. Both pushover loads were run from final conditions of dead load.
Description of Analyzed Buildings
In this paper (G+10) and (G+20) stories buildings are preliminary designed by Sap 2000 v.19 for seismic Zone V. Buildings were designed as Special Moment Resisting Frame (SMRF) as per IS1893-2002. Plan Dimensions of buildings kept as 20m x 15m with 5 bays in X-Direction and 3 Bays in Y-Direction (Figure-2).
Figure 2. Plan of Building
Storey height for ground floor is kept as 3.5 m and typical storey height is kept uniform for all stories i.e. 3 m. Slab thickness is kept 125 mm for all floors.. Shear wall is modeled as Mid pier frame element. Thickness of shear wall is taken equal to 200 mm. As the building is symmetric shear wall is provided in central bay of longer side of building frame. M25 Grade of Concrete and Fe415 Grade of steel are used in all members.
Loads Considered
Following loads are considered for analysis of all buildings. Live load: 3.5 kN/m2 on all floors. As per IS 875 (Part-2)
Dead Load: 3 kN/m2 on all floors and 16.8 kN/m wall load on all beams. Earthquake load: as per IS1893:2002 (Part1)
Type of Soil: Medium Soil (Type II) as per IS1893 (2002) Importance factor, I : 1
The time period for both buildings is program calculated as per IS 1893(2002) was used to calculate the earthquake force for both buildings. For earthquake force analysis in Sap 2000 following loads were considered in the mass source: Dead load plus
-
times live load. The building is designed according to IS456:2000 using SAP 2000 v.19 considering live load, dead load and Earthquake load in both directions X and Y with minimum eccentricity. The dimensions of beams and columns and designed reinforcement details of columns are mentioned in Table-1 and Table-2. Columns A1,A2,A3,A4,B1,B2,B3,B4,C1,C2,C3,C4,D1,D2, D3,D4,E1,E2,E3,E4, and F1,F2,F3,F4 can be identified from figure-2.
Table-1 (G+10) Structural Details as per Analysis and Design in Sap2000
Storey
Height m
Beam Size (BxD)
mm
Column Size (BxD)
mm
Reinforcement Percentage in Column %
A1,A4 F1,F4
A2,A3 F2,F3
B1,B4 E1,E4
B2,B3 E2,E3
C1,C4 D1,D4
C2,C3 D2,D3
11th
33.5
200 x 325
325 x 325
0.8
0.8
0.8
0.8
0.8
0.8
10th
30.5
200 x 325
325 x 325
0.8
0.8
0.88
0.8
0.85
0.8
9th
27.5
200 x 375
375 x 375
0.8
0.8
0.83
0.8
0.81
0.8
8th
24.5
200 x 375
375 x 375
0.8
0.8
0.96
1.51
0.86
1.37
7th
21.5
200 x 375
375 x 375
0.8
1.32
1.72
2.69
1.62
2.54
6th
18.5
275 x 425
425 x 425
0.8
0.8
0.81
1.67
0.8
1.65
5th
15.5
275 x 425
425 x 425
0.8
0.92
0.99
2.4
0.95
2.65
4th
12.5
275 x 425
425 x 425
0.8
1.5
1.49
3.34
1.47
3.65
3rd
9.5
300 x 450
450 x 450
0.8
1.41
1.14
3.46
1.14
3.77
2nd
6.5
300 x 450
450 x 450
0.8
1.82
1.49
4.3
1.64
4.65
1st
3.5
300 x 450
450 x 450
1.69
2.98
2.22
5.29
2.31
5.6
Table-2(G+20) Details as per Analysis and Design in Sap2000
Storey
Height m
Beam Size (BxD) mm
Column Size (BxD) mm
Reinforcement Percentage in Column %
A1,A4 F1,F4
A2,A3 F2,F3
B1,B4 E1,E4
B2,B3 E2,E3
C1,C4 D1,D4
C2,C3 D2,D3
21st
63.5
225 x 375
400 x 400
0.85
0.8
0.88
0.8
0.84
0.8
20th
60.5
225 x 375
400 x 400
0.8
0.8
0.8
0.8
0.8
0.8
19th
57.5
225 x 375
400 x 400
0.8
0.8
1.45
1.58
1.29
1.29
18th
54.5
300 x 450
450 x 450
0.8
0.8
1.41
1.63
1.25
1.35
17th
51.5
300 x 450
450 x 450
0.8
1.04
1.65
2.31
1.53
2.08
16th
48.5
300 x 450
450 x 450
1.18
1.82
2.38
3.25
2.27
3.03
15th
45.5
325 x 500
500 x 500
0.8
1.35
1.78
2.63
1.71
2.48
14th
42.5
325 x 500
500 x 500
1.05
1.93
2.12
3.14
2.05
3.11
13th
39.5
325 x 500
500 x 500
1.72
2.77
2.78
4.01
2.74
4.03
12th
36.5
375 x 550
550 x 550
1.13
2.06
2.03
3.08
1.98
3.45
11th
33.5
375 x 550
550 x 550
1.58
2.61
2.38
3.74
2.37
4.17
10th
30.5
375 x 550
550 x 550
2.16
3.25
2.86
4.37
2.86
4.95
9th
27.5
400 x 600
600 x 600
1.56
2.62
2.15
3.72
2.28
4.19
8th
24.5
400 x 600
600 x 600
1.96
3.13
2.5
4.28
2.78
4.86
7th
21.5
400 x 600
600 x 600
2.5
3.75
2.89
4.93
3.3
5.53
6th
18.5
450 x 650
650 x 650
1.93
3.09
2.35
4.14
2.72
4.7
5th
15.5
450 x 650
650 x 650
2.38
3.66
2.77
4.67
3.16
5.32
4th
12.5
450 x 650
650 x 650
2.78
4.16
3.18
5.26
3.64
5.89
3rd
9.5
500 x 700
750 x 750
2.3
3.57
2.64
4.47
2.99
5.18
2nd
6.5
500 x 700
750 x 750
2.68
4.04
2.98
5.04
3.44
5.71
1st
3.5
500 x 700
750 x 750
3.04
4.52
3.44
5.6
3.84
5.07
RESULTS & DISCUSSION
The seismic performance of a building is evaluated in terms of capacity curve, performance point and plastic hinge formation and storey drift.
Capacity Curve
Pushover Analysis gives an idea of the maximum base shear that the structure can resist at the time of the seismic load. For regular buildings, it can also give a rough idea about the global stiffness of the building. Capacity Crve obtained after pushover analysis is plotted in Figure-3 and Figure-4. It is observed that Buildings with shear wall is capable to resist larger base shear as compared to bare frame buildings. Maximum Displacement was also observed in bare frame building.
7000
Base Shear (KN)
Base Shear (KN)
6000
5000
4000
3000
2000
1000
0
0 500 1000 1500
G10 PU X
G10 PU X With SW
Roof Displacement(mm)
Base Shear (KN)
Base Shear (KN)
Figure 3. Capacity Curves of (G+10) Buildings for Pushover in X-Direction
14000
12000
10000
8000
6000
4000
2000
0
G20 PU X
G20 PU X With SW
14000
12000
10000
8000
6000
4000
2000
0
G20 PU X
G20 PU X With SW
0 1000 2000 3000
Roof Displacement (mm)
0 1000 2000 3000
Roof Displacement (mm)
Figure 4. Capacity Curves of (G+20) Buildings for Pushover in X-Direction
Status of Hinges at Ultimate Stage
Status of plastic hinges at the ultimate stage for (G+10) and (G+20) is shown in figure 5 and 6.
Figure 5. Status of Hinges at Ultimate Stage in Pushover-X Case
A table of hinge status at the ultimate stage is shown in Table-3. It was observed that in (G+10) building without shear wall 74 hinges are formed between immediate occupancy and life safety range and 228 hinges are formed beyond collapse range, by addition of shear wall only 18 hinges are formed between immediate occupancy and life safety range .So there is considerable decrease in hinge formation by addition of shear wall and building lies between immediate occupancy and life safety range.
Table 3. Status of Hinges at Ultimate Stage
Building
Monitored Displacement (mm)
Base Force (KN)
O-IO
IO-LS
LS-CP
>CP
Total
(Nos.)
(Nos.)
(Nos.)
(Nos.)
(Nos.)
G10 P X
1340
2753.549
1062
74
0
228
1364
G10 P X SW
226
5687.324
1390
18
0
0
1408
G20 P X
2540
4998.35
2084
96
0
424
2604
G20 P X SW
395
11937.755
2688
0
0
0
2688
Building
Monitored Displacement (mm)
Base Force (KN)
O-IO
IO-LS
LS-CP
>CP
Total
(Nos.)
(Nos.)
(Nos.)
(Nos.)
(Nos.)
G10 P X
1340
2753.549
1062
74
0
228
1364
G10 P X SW
226
5687.324
1390
18
0
0
1408
G20 P X
2540
4998.35
2084
96
0
424
2604
G20 P X SW
395
11937.755
2688
0
0
0
2688
O:
Operational Level, IO: Immediate Occupancy Level, LS: Life Safety Level, CP: Collapse Prevention Level
In (G+20) building without shear wall 96 hinges are formed between immediate occupancy and life safety range and 424 hinges are formed beyond collapse prevention state, but with shear wall addition hinges are not formed beyond immediate occupancy state. Building lies at immediate occupancy state, thus both buildings performed well up to design seismic load and it can be easily said that performance of high rise building is more affected than medium rise building with addition of shear wall.
Figure 6. Status of Hinges at Ultimate Stage in Pushover-X case
Capacity Demand Spectrum
Capacity demand spectrum is the representation of structures ability to resist the seismic demand. The point of intersection of capacity spectrum and demand spectrum is required performance point. Figure 7 and 8 shows the capacity spectrum curve as per ATC-40 of (G+10) and (G+20) buildings with shear wall respectively. Table 4 shows the performance point in terms of different structural parameter like base shear (V), displacement (D), spectral acceleration (Sa), spectral displacement (Sd), effective time period (Teff), effective damping (eff).
Table 4. Performance Point in terms of (V, D) (Sa, Sd) (Teff, eff)
Performance Point
G10 P
G10 P SW
G20 P
G20 P SW
Performance Point (V, D)
(3297.138, 0.222)
(5563.003, 0.165)
(7393.275, 0.287)
(10806.918, 0.262)
Performance Point (Sa, Sd)
(0.081, 0.179)
(0.155, 0.112)
(0.062, 0.223)
(0.099, 0.184)
Performance Point (Teff, eff)
(2.969, 0.243)
(1.707, 0.196)
(3.814, 0.261)
(2.732, 0.182)
Figure 7 Figure 8
Storey Drift
Storey Drift of (G+10) and (G+20) storey buildings for push x case with and without shear wall designed for dead load, live load and the earthquake load is presented in figure-9 and 10. All buildings satisfy the strength requirements as well as satisfy inter-story drift criteria. As per 1893: 2002 clause no. 7.11.1 maximum permissible inter-storey drift is 0.004 times the storey height.
0.09
0.08
Storey Drift (Unitless)
Storey Drift (Unitless)
0.07
0.06
0.05
0.04
0.03
0.02
0.01
0
0 2 4 6 8 10 12
No. of Stories
G10 PU X
G10 PU X with SW
Storey Drift (Unitless)
Storey Drift (Unitless)
Figure 9. Storey Drift in X-Direction for (G+10)
0.1
0.09
0.08
0.07
0.06
0.05
0.04
0.03
0.02
0.01
0
G20 PU X
G20 PU X with SW
0.1
0.09
0.08
0.07
0.06
0.05
0.04
0.03
0.02
0.01
0
G20 PU X
G20 PU X with SW
0 5 10 15 20 25
No. of Stories
0 5 10 15 20 25
No. of Stories
Figure 10. Storey Drift in X-Direction for (G+20)
CONCLUSIONS
In this work (G+10) and (G+20) stories buildings with and without shear wall have been taken for their seismic performance evaluation. Both buildings were designed for seismic zone V of India as per IS1839:2002 and IS456:2000 . Pushover analysis of buildings was carried out using Sap 2000 nonlinear software tool. Based on the present study, the following conclusion can be drawn.
-
From pushover analysis it is seen that capacity of structure increases with the introduction of shear walls in both G10 and G20 buildings.
-
There is a considerable reduction in hinge formation between immediate occupancy and life safety range and no hinge formation above life safety range with addition of shear wall.
-
As per IS 1893-2002 allowable storey drift requiement is 0.004 times storey height which is satisfied by both G10 and G20 buildings.
-
It is concluded that pushover analysis gives a better view of building performance under seismic load and its failure mechanism.
REFERENCES
-
J.Parishith & V. Preetha (2017). Pushover Analysis of RC Frame Buildings with Shear Wall : A Review. IJSRD – International Journal for Scientific Research & Development| Vol. 4, Issue 12, 2017 | ISSN (online): 2321-0613
-
N.M. Nikam and L.G. Kalurkar, (2016), Pushover Analysis of Building with Shear Wall, International Journal of Engineering Science and Computing, vol. 6, no. 8, pp. 2916-2918
-
Nitin Choudhary & Prof. Mahendra Wadia (2014). Pushover Analysis of R.C. Frame Building with Shear Wall. IOSR Journal of Mechanical and Civil Engineering (IOSR-JMCE) e- ISSN: 2278-1684,p-ISSN: 2320-334X, Volume 11, Issue 2 Ver. V (Mar- Apr. 2014), PP 09-13.
-
S. C. Pednekar, H. S. Chore, & S. B. Patil (2015) Pushover Analysis of Reinforced Concrete Structures, International Journal of Computer Applications (0975 8887),International Conference on Quality Up-gradation in Engineering, Science and Technology (ICQUEST2015)
-
Lakshmi K.O., Prof. Jayasree Ramanujan, Mrs. Bindu Sunil, Dr. Laju Kottallil & Prof. Mercy Joseph Poweth (2014) ISOI Journal of Engineering and Computer science Volume 1 Issue 1; Page No. 07-17.
-
M.D. Kevadkar, P.B. Kodag (2013) (IJMER) International Journal of Modern Engineering Research Vol.3, Issue.3, May-June. 2013 pp-1428-1434
-
ASCE, 2000,Prestandard and Commentary for the Seismic Rehabilitation of Buildings, FEMA 356 Report, prepared by the American Society of Civil Engineers for the Federal Emergency Management Agency, Washington, D.C.
-
ATC, 1997a, NEHRP Guidelines for the Seismic Rehabilitation of Buildings,FEMA 273 Report, prepared by the Applied Technology Council for the Building Seismic Safety Council, published by the Federal Emergency Management Agency,Washington, D.C.
-
Dakshes J. Pambhar (2012) Performance Based Pushover Analysis of R.C.C. Frames, International Journal of Advanced Engineering Research and Studies E-ISSN22498974, IJAERS/Vol. I/ Issue III/April-June, 2012/329-333
-
P. Poluraju, P. V. S. Nageswara Rao, (2011) Pushover analysis of reinforced concrete frame structure using SAP 2000, International Journal of Earth Sciences and Engineering ISSN 0974-5904, Volume 04, No 06 SPL, October 2011, pp. 684-690
-
S.R Satish Kumar, G.Venkateswarlu, (2008) Performance based seismic design of reionforced concrete plane frames, The 14th World confrence on earthquqke engineerin October12- 17, 2008, Beijing,China.
-
Vipul Prakash, (2004) Whither Performance-Based Engineering in India? ISET Journal of Earthquake Technology, Paper No. 447, Vol. 41, No. 1, pp. 201-222, March 2004.
-
IS 456: 2000, Plain and Reinforced Concrete – Indian Standard Code of Practice, Bureau of Indian Standards, New Delhi, India. .
-
IS 1893 (Part 1): 2002, Criteria for Earthquake Resisting Design of Structures- Indian Standard Code of Practice, Bureau of Indian Standards, New Delhi, India