
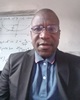
- Open Access
- Authors : Ogini Arthur. A , Airen Osariere. J
- Paper ID : IJERTV10IS100116
- Volume & Issue : Volume 10, Issue 10 (October 2021)
- Published (First Online): 01-11-2021
- ISSN (Online) : 2278-0181
- Publisher Name : IJERT
- License:
This work is licensed under a Creative Commons Attribution 4.0 International License
Parameter Specification and 2-D Geo-Electrical Resistivity Modeling for Groundwater-Iron Prediction in Selected Niger Delta Transition Aquifers, Southern Nigeria
Ogini Arthur. A.1
1Department of Physics University of Benin, Benin City, Edo State, Nigeria
Airen Osariere. J.2
2Department of physics University of Benin, Benin City, Edo State, Nigeria
Abstract- The use of geo-electrical resistivity survey to identify water bearing zones (aquifers) in porous rocks is trivial, but the delineation of groundwater quality using the same scheme still remains a predominantly subjective exercise with peculiarities that may vary from locality to locality. 2-D geoelectrical resistivity modelling with acquisition/interpretational parameter specification for localized groundwater-iron prediction was carried out in selected transition aquifers in Bayelsa State, Southern Nigeria. The aim of the study was to predict the concentration of iron in groundwater by specifying parameters for its direct interpretation from 2-D resistivity survey using integrated geoelectrical and physicochemical data in the study area. It was observed that an index resistivity value greater than 200 m ( 200 m) could be tied to iron (II) Fe2+ concentrations less than the WHO limit of 0.3 mg/lit indicative of best groundwater quality in the study area. Also, a 2-D resistivity survey dipole spacing of 2.5 m and depth of investigation of about 12.5 m using a 21 electrode system in a combined Wenner-Schlumberger Configuration was recommended for near surface groundwater aquifer exploration in the survey area.
Keywords- Parameter Specification; Geoelectrical Resistivity Modelling; Groundwater Iron Prediction
I. INTRODUCTION
The geology of the Niger delta is summarized by three major formations: the underlying Akata formation composed mainly of shale (hydrocarbon source rocks), the Agbada formation composed of sandstone and shale intercalation which constitutes the major reservoir rocks that harbour the regions hydrocarbon, and the topmost Benin formation which is of hydrogeological significance as it is known for groundwater resources potentials [25, 26, 29 and 16]. The sedimentation pattern as well as stratification determines both the quality and quantity of water in the Niger delta aquifers, this calls for detailed investigation of the Benin formation [7and 4]. The main body of water in the Niger Delta is contained in very thick and extensive sands and gravel of the Benin formation wherein three main subzones were differentiated: (1) a
northern bordering zone consisting of shallow aquifers of predominantly continental deposits, (2) a transition zone of intermixing of marine and continental materials and (3) a coastal zone of predominantly marine deposits. Distinct trends in aquifer properties have been observed following this division [10, 3 and 9] even as these three major hydrological aquifers have been tied to water quality in some known towns/cities according to the following category: Port Harcourt, Ogoni, Elele and parts of Imo state including Owerri have been tied to the Northern and North-eastern Niger Delta hydrological aquifer composed mainly of unconfined, clean and friable sandstone of continental origin situated at depths of less than 60 m from the surface, they constitute the most prolific aquifer with best water quality in the Niger Delta. The second, referred to as the transition zone aquifers, were found to occur in Bayelsa and Delta states, a common feature of this zone is clay embodiment or intercalation within the aquifers. It was noted that the quality of water in the transition zone is usually poor often infested with provocative smells in some places and predominantly high iron concentrations in most places. The third hydrological aquifer zone in the Niger Delta falls within the coastal bars and beaches where saltwater contamination remains a major problem [20]. It was noted that the transition aquifers in the study area are highly internally subdivided causing water quality variation even within a locality with high iron concentration being the major problem to groundwater development plans [22]. In groundwater exploration, various geophysical methods have been employed to locate suitable points for productive boreholes; one of such methods commonly used is the electrical resistivity method in which Vertical Electrical Sounding (VES) and Horizontal Profiling (HP) are commonly carried out [24, 6, 14, 12 and 15]. The electrical resistivity of rock is a property which depends on lithology and fluid contents.
[31] and [18] have detailed the principles and application of the resistivity method with well developed software presently in use, it was noted that where good electrical resistivity contrast exist between a water bearing formation and the underlying rocks, current is conducted electrolytically as well as superficially in the effective surface of dry minerals in dry/wet layers. Aquifer resistivity is controlled by the resistivity of pore fluids (water content and water quality),small grain sizes particularly with the presence of clay minerals can cause spurious fluctuations in aquifer resistivity signals [5]. The inclusion of clay as admixtures in aquifers is most likely to produce poor groundwater quality which manifests as low electrical resistivity signals where conductivity in aqueous medium varies considerably depending on such factors as; the amount of dissolved chlorides, sulphates and other mineral present. While the geometrical arrangement of the interstices of porous rocks may seem to have less pronounced effect on conductivity, it was found that this property referred to as texture/packing have potential of causing some level of anisotropy in field observations [27, 13]. In most parts of the Niger delta, government and institutions are ignorant of the near surface geology, hence lacked relevant knowledge and information on the groundwater quality variation which may be remarkably inhomogeneous within a particular locality, this is attributable to an inherent paucity of relevant studies in the area making effective groundwater development plan difficult with high groundwater iron concentrations being the major nuisance [22]. In the presence of the identified data paucity especially in the bayelsa state axis of the Niger Delta, it is worthy of note that similar studies have been carried out in some parts of southern Nigeria in recent times. For instance,
[17] employed electrical resistivity survey to delineate aquifers in Akwa Ibom state, Niger delta. [19] also used similar method to evaluate groundwater potential in parts of Delta state, Niger delta while [23], [28] and [1]) are all recent studies wherein Electrical Resistivity Imaging (ERI) was integrated with seismic and physicochemical methods to map out the subsurface lithology and/or water content in the south-western part of Nigeria. The same can be said of similar studies carried out by [2] and [9]; to all intent and purpose, the studies integrated geoelectrical, physicochemical and perhaps acoustic methods to decipher lithology and water content (aquifer). But the aspect of calibrating water quality in a subsurface zone that has been interpreted to be an aquifer with quantitative electrical resistivity range of values so that water quality can be inferred directly from a resistivity profile with known background geology based on a localized template is yet to be addressed, and that is the perspective this study seeks to illuminate. Also, its pertinent to note that portable drinking water is defined based on colour, odour, taste, coli form load and dissolved mineral content [30]. The World Halth Organization (WHO) prescribed the limits of iron (II) in drinking water to be 0.3 mg/L.STUDY AREA
The study area is located in Bayelsa state South-South Nigeria, referred to as the transition zone of the Niger Delta and known for its complexity in near surface sedimentation and groundwater characteristics. In terms of geospatial coordinates, the area lies within longitude (60 12 30 and 60 24 30) E; and Latitude (40 47 00 and 050 03 00) N
(Figure A1 and A2).
Figure A1: Map of the Niger Delta Showing Bayelsa State and Yenagoa (adapted from STRATFOR.com)
Figure A2: Map Showing the Three (3) Study Locations
MATERIALS AND METHOD
-
Geoelectrical Investigation
Pasi Earth Resistivity Meter model 16GL-N was used for data acquisition and Wenner-Schlumberger survey geometry was employed using a 21 electrode system with spacing of 10 m or 5 m in some profiles depending on the availability of space. Four reels of wire; two potential and two current electrodes wires each provided with crocodile clips. 2-D geoelectric resistivity profiles were run in three (3) locations: four (4) in the premises of the Niger delta University Teaching Hospital (NDUTH) Okolobiri as shown in Figure 4.1; and three (3) profiles each in Okaka/Ekeki communities and the Federal University Otuoke (FUO) respectively. 2-D resistivity data acquisition procedure as prescribed by [18] was used where the instrument recorded voltage and currents as input signals and outputs resistance (R) in ohms. The data processing was done by multiplying instrument field resistance R readings with a geometric factor k representing the influence of the earths geometry, texture, structure and composition on the observed field recording [14, 8]. Inversion was done using DiproWin software and data interpretation done by extracting relevant information such as strata logs, depth to aquifer, electrical resistivity
values of the various units (clay, silts and sands) from the 2-D resistivity structure. At the aquifer level, zones of uniform electrical resistivity with wide lateral extent of sand body were noted and picked as horizons of interest. The drilling points were carefully selected at locations where these extensive sand bodies characterized by uniform electrical resistivity contours bulge closer to the surface with a view to minimize drilling costs. Apparent resistivity logs were also extracted from 2-D profiles to show the electrical resistivity variation with depth and perhaps to provide pre information on what should be expected from the drilling. Also, resistivity surfaces corresponding to aquifer horizons were extracted from the geo-electrical data to produce a three dimensional view of the electrical behaviour of the aquifers under investigation with resistivity as the third dimension. The structural closures of the resistivity highs constitute possible targets in line with mathematical relations highlighted in [21].
-
Lithologic logging/Physicochemical analysis
2- – –
2- – –
Guided by aquifer depth information derived from the geoelectrical data interpretations, six (6) boreholes were proposed; two (2) in each of the three (3) locations. While drilling, lithology logs were generated at 3 m interval, and water samples collected at the reach of major aquifers. The water samples were immediately labelled with masking tape and taken to the laboratory for physicochemical analysis within 12 hours from the time of collection. Six (6) samples in all comprising of five (5) borehole samples and (1) bottled water which served as control were analysed. Six (6) major cations (Mn2+, Fe2+, K+, Na+, Mg2+, Ca2+) and four (4) anions (Cl-, SO4 , NO3 , HCO3 ) were analysed, their concentrations recorded in parts per million or mg/litre, and the World Health Organisation (WHO) standards for water quality used as reference.
-
Data Integration
Integrated/comparative analyses were carried out on geoelectrical/physicochemical data sets and final inference made based on statistical measures of central tendency.
RESULTS AND INTERPRETATION
Figure 4.2 is the 2D resistivity section of traverse 1 at the Niger Delta University Teaching Hospital location of the study area. The major sand body occurred at depth less than
10 m, corresponding to the 20 m electrode position with maximum resistivity of 169 m. To the right of the section, resistivity values seem to be spurious with values as low as
2.1 m, 3.4 m, 9.1 m, 39 m and 64 m indicative of the presence of conductive fluids and/or layers with clay minerals. The 3D resistivity surface extracted at the horizon containing the major aquifer (figure 4.4) showed major resistivity contours of 50 m, 100m and 150 m, with the 150 m closures forming domes while observable resistivity structural depressions occur between the 50 m closures. Figure 4.3 correlates resistivity to observed lithology with respect to depth in the Niger Delta University Teaching Hospital (NDUTH) study location. The above interpretation was repeated in the remaining two study locations; Federal University Otuoke (FUO) and Okaka Primary School Field
(OPS)/Ekeki Flood Plane (EFP), where comparison was made between physicochemical and geoelectrical parameters of the near surface aquiferous units of the study area as summarized in the tables 4.1 and 4.2 respectively. Table 4.3, is the statistical analysis of physicochemical data and figure 4.5; the Piper trilinear plot of the hydrochemical facies of the study area [32].
Figure 4.1: Google Earth Satellite Imagery showing 2D Survey Profiles at the NDUTH Project Location (http//www.google/imagery/earth)
Figure 4.2: 2D Structural Resistivity Sections of Traverse1 (NDUTH)
Figure 4.3: Lithologic and Apparent Resistivity Logs of Borehole at Niger Delta University Teaching Hospital Okolobiri.
Figure 4.4: 3D Resistivity Surface of Water Bearing Horizon/Aquifer in the Okolobiri/NDUTH Study Location
Table 4.1: Physicochemical Parameters of Water Sample with WHO Limits
NB. FUO Federal University Otuoke; NDUTH Niger Delta University Teaching Hospital; OPS Okaka Primary School; EFP Ekeki Floodplain; C1 – First Control; C2 Second Control
Table 4.2: Horizon Resistivity-to- Hydrochemical Parameter Tie
Table 4.3: Mean and Standard Deviation of Major Mineral Components of Borehole Water Samples
NB. NDUTH Niger Delta University Teaching Hospital; FUO Federal University Otuoke; OPS – Okaka Primary School field; EFP – Ekeki Floodplain
Figure 4.5: Piper Trilinear Plot Showing Hydrochemical Facies in the Study Area
DISCUSSION
The water bearing horizon at the Niger Delta University Teaching Hospital profile 1 (NDUTH-1) occurred at a depth of 9 12 m with fine-medium sands as litho facies (table 4.2). The observed resistivity fell within the range 64 104 m corresponding to an iron concentration of 1.48 mg/lit whereas all other chemical components are observed to be relatively low in comparison with WHO standards (table 4.1). In profile-5 of the Federal University Otuoke study location (FUO-5) (see Figure A2), the water bearing sands occur at a depth range of 10 12 m with iron concentration of 1.64 mg/lit which was the highest recorded groundwater iron (II) concentration in the study. On the other hand, traverse EFP- 10 in the Ekeki flood plain (Figure A2) recorded the highest resistivity 273 – 377 m coinciding with zero iron (II) concentration where the major water bearing sand unit occurred at a depth range of 5-10 m. With the correlation of table 4.2, it was observed that the groundwater iron (II) concentration has an inverse relationship with the observable resistivity signals, meaning that where the iron (II) concentration is relatively high; resistivity tend to be low, and where iron concentration is relatively low, resistivity tends to be high. This observation is however in line with classical
theoretical relationships between electrical resistivity and conductivity inearth materials (Ogini and Airen, 2021). From table 4.2, it can be inferred that aside the relatively high concentrations of sodium and chloride ions of 86.7 mg/lit and 44 mg/lit respectively observed in OPS-9; the concentrations of all other major anions and cations tend to cluster around common value ranges. For instance, the concentration of magnesium in all four boreholes, cluster within the range (4.6
5.6) mg/lit, the same observation held good for all the major ions in all three locations. A computation of their Standard Deviation (SD) across the four data points (table 4.3) showed small Standard Deviation (SD) values in comparison with their statistical means. It could be inferred that the data are less dispersed or tend to cluster about their mean values which makes logical the assumption that the contribution to electrolytic conductivity within the aquifers of the study area due to variation in the concentrations of major hydrochemical ions shown in the Piper trilinear plot (figure 4.5) may not have played significant role in the localized variation of electrical resistivity recorded by the 2D electrical survey even when the Piper diagram confirmed that the groundwater in the study area is of the calcium chloride type. Rather, the two observable high concentrations of iron (II) at the Niger Delta University Teaching Hospital (NDUTH) and the Federal University Otuoke (FUO) as seen in table 4.1 seem to have been represented in the subsurface geoelectrical signature as an increase in electrical conductivity. It could be seen that the concentration of iron (II) in the aquifers presents a bolder image when viewed under the lens of electrical resistivity wherever it occurs in excess of 1 mg/lit in the subsurface of the study area (Figure 4.2).
CONCLUSION
In consideration of the surface to aquifer depth relationship in the study area where the aquifers have been found not to exceed 10 m of depth on the average, and with a view to optimize resolution in data acquisition, the following parameters were specified: For 2D electrical imaging in the study area using a 21 electrode integrated Wenner- Schlumberger configuration with groundwater exploration objectives; the probe depth should be placed at 12.5 m which is achievable using an electrode dipole (a) spacing of 2.5 m. Also, for the purpose of predicting the concentration of groundwater iron from 2D geoelectrical resistivity data prior to drilling in the study area; it was specified that a subsurface electrical resistivity greater than 200 m (>200 m) occurring in a lithology interpreted to be sand (aquifer) is indicative of groundwater iron (II) concentration less than 0.3 mg/lit (WHO limit) where all other factors remain unchanged.
REFERENCES
-
Adedibu S. A., and Isaac, B. O. (2021) Subsurface geological, hydrogeophysical and engineering characterisation of Etioro-Akoko, southwestern Nigeria, using electrical resistivity tomography, NRIAG Journal of Astronomy and Geophysics, 10:1,43-57, DOI: 10.1080/20909977.2020.1868659
-
Akingboye, A. S., Osazuwa, I. B., and Mohammed, M.
Z. (2019). Electrical resistivity tomography for
sustainable groundwater development in a complex geological area. Mat Geoenviron. 66 (2):121128. doi:10.1515/rmzmag-2019-0004
-
Amanjor, L.C (1989). Geological Appraisal of Groundwater Exploration in Eastern Niger delta: (ed. Ofoegbu, E.O) Groundwater and Mineral Research of Nigeria: Braunsschweig/Weisbaden, FriedrViewage and Sohn, pp. 85-100.
-
Amajor, L. C., and Gbadebo, A. M. (1992). Oilfield brines of meteoric and conate origin in the eastern Niger Delta. Journal of pet. Geol. Vol (15) issue 3, pp. 481-488
-
Archie, G. E. (1942). The Electrical Resistivity log as an Aid in Determining Some Reservoir characteristics. Transactions of the American Institute of Mining, Metallurgical and Petroleum Engineering (AIME), 146: 398 409
-
Dey, A. and Morrison, H. F. (1979). Resistivity modelling for arbitrary shaped 2-dimensional structures. Geophysical Prospecting (27) 1020 1036.
-
Dickey, P., George, G. O., Baker, C. (1987). Relationships between oil and water compositions in the Niger Delta. Am. Assoc. Pet. Bull., 71(10):1319-1328.
-
Dobrin, M.B., (1976). Introduction to geophysical prospecting, 3rd ed., McGrawHill, New York.
-
Emekeme, R. I., Ofomola, M. O., Bawallah, M., and Ochuko, A. (2017). Lithological Identification and Underground Water Conditions in Jeddo Using Geophysical and Geochemical Methods. Hydrology 4(42). Doi:10.3390/hydrology 4030042.
-
Etu-Efeotor, J. O. and Akpokodje, E.G (1990). Aquifer systems of the Niger Delta. Journal of mining and geology, 26(2): 279-285.
-
Etu-Efeotor, J. O. and Odigi, M. I. (1983). Water supply problems in the Eastern Niger Delta. Jour. Min.Geol. 26(2):279 279.
-
Griffihts, D. H., Turnbull, J., and Olayinka, A. I. (1990). 2- Dimensional Resistivity Mapping with Computer Controlled Array. First Break (8) 121 129.
-
Hadi, T. N (2009). Geoelectric investigation of the Aquifer Characteristics and groundwater Potential in Behbahan Azad University farm, Khuzestan province, Iran. Journal of Applies sciences 9(20): 3691-3698.
-
Kearey P., and Brooks, M. (1991). An Introduction to Geophysical Exploration, Blackwell science limited, London. Pg 281
-
Kizlo, M. and Kansbergs, A. (2009). The Cause of the Parameter Changes of Soil Resistivity. The 50th International scientific Conference, Power and Electrical Engineering pp. 43-46
-
Kogbe, C. A. (1976). Geology of Nigeria. Elizabeth Pub. Co. Lagos Nigeria.
-
Laouini G, Sunday EE, Okechukwu EA (2017) Delineation of aquifers using Dar Zarrouk parameters in parts of Akwa Ibom, Niger Delta, Nigeria. J Hydrogeol Hydrol Eng 6(1):18. https://doi.org/10.4172/2325-9647.1000151
-
Loke, M. H. (2000). Topographic Modeling in Resistivity Imaging Inversion. 62nd EAGE Conference and Technical Exhibition Extended Abstracts
-
Nwanchukwu, S., Bello, R., and Balogun, A. O. (2019). Evaluation of groundwater potential of Orogun South-South part of Nigeria using electrical resistivity method, Journ of applied water science 9(84)
-
Nwankwoala, H.O and Ngah, S.A (2014). Groundwater resource of the Niger Delta: Quality implication and management consideration. Internal journal of water resource and environmental engineering, 6(5), 155-165.
-
Ogini, A. A. and Airen, O. J (2021). Electrical Characterization of near Surface Lithology in Otuoke Community: An Inquiry into Localized Geoelectrical Lithologic Logging in Bayelsa State, Niger delta,
Southern Nigeria. JMEST, 8(2), pp. 13452 13458
-
Okiongbo, K. S., and Akpofure, E. (2012). Determination of Aquifer Properties and Groundwater Vulnerability Mapping using Geoelectric Method in Yenagoa city and its Environs in Bayelsa state, south-South Nigeria. Journal of Water Resource and Protection, (4), pp. 354-362.
-
Olatinsu, O. B., Oyedele, K. F., Ige-Adeyeye, A. A. (2018). Electrical resistivity mapping as a tool for
post-reclamation assessment of subsurface condition at a sandfilled site in Lagos, southwest Nigeria. SN Applied Sciences. 1(1):24. doi:10.1007/s42452-018-0028-5
-
Omosuyi, G. O., Adeyemo, A. and Adegoke, A. O. (2007). Investigation of groundwater prospect using electromagnetic and geoelectric sounding at Afunbiowo, near Akure southern Nigeria. pacific journal of science and technology, 8: 172 182
-
Rayment, R. H. (1965). Aspects of the geology of Nigeria: the Stratigraphy of the Cretaceous and Cenozoic deposits. Ibadan University press. 23-27.
-
Short, K. C. and Stauble, A. J. (1967). Outline Geology of the Niger Delta. AAPG bull 51;761-779.
-
Telford, W. M, Geldart, L. P, Sherrif, R.E, and Keys, D., (1976). Applied Geophysics, Cambridge University press. New York.
-
Uwaezuoke, C. C., Ishiola, K. S.,and Ayolabi, E. A. (2021). Electrical Resistivity Imaging and Multichannel Analysis of Surface Waves for Mapping the Subsurface of a Wetland Area of Lagos, Nigeria. NRIAG journal of astronomy and geophysics, 10(1), pp. 300-319.
-
Weber, K. J., and Daukoru, E. M. (1975). Petroleum Geology of the Niger Delta. Journal of Mining Geol (12); 9-12.
-
W.H.O, (2021). Guidelines for drinking water quality, 4th edition, incorporating the 1st adendum
-
Zohdy, A. A. R., Eaton, J. P. and Mabey, D. R. (1974). Application of Surface Geophysics to Groundwater Investigation, 1st edition. USGS publication, USA, pp. 166
-
Piper, A. M. (1944). A Graphical Solution in the Geochemical Interpretation of Water Analysis. American Geophysical union Transaction.25(6):835-837