
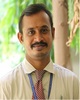
- Open Access
- Authors : Dr. N. Saravanakumar , K. Madumathi , T. Somasundram , C. Yasodha
- Paper ID : IJERTV12IS030003
- Volume & Issue : Volume 12, Issue 03 (March 2023)
- Published (First Online): 15-03-2023
- ISSN (Online) : 2278-0181
- Publisher Name : IJERT
- License:
This work is licensed under a Creative Commons Attribution 4.0 International License
Insulation Materials for Cryogenic Environments: An Investigation
Dr. N. Saravanakumar
ASP/EEE Mahendra institute of Technology, Namakkal,Tamil Nadu
Mr. T.Somasundram
ASP/EEE Mahendra institute of Technology, NamakkalTamil Nadu
Mrs. K. Madumathi
AP AP/EEE Mahendra institute of Technology, NamakkalTamil Nadu
C. Yasodha
AP/EEE Mahendra institute of Technology, Namakkal,Tamil Nadu
Abstract – The high-voltage insulation technology encompasses the invention, selection, design, fabrication, operation, and maintenance of high-voltage electrical equipment insulation structures. Advancements in insulation technology will be crucial to modernizing energy systems in the future. There is an extensive variety of modern technology which includes high-voltage direct current, state-of-the-art electricity garage systems, solar power, electricity-green offshore wind turbines, microgrids, and hybrid renewable electricity. The use of all advanced technologies has meant that insulation systems must meet ever-increasing regulations. The paper reviews the various developments in insulating materials since the early 20th century in terms of their properties and applications, including FGM, electrical strength, and electrical conductivity. Also, this paper affords a precis of the evolution of insulation substances for HVDC applications, in addition to the creation of thermoplastic polymers that enhance product recyclability.
Keywords: High-voltage; Electrical treeing; Electrical Insulation; Electrical Conductivity; Field Grading Materials; Technology.
I INTRODUCTION
While our electrical infrastructure has largely remained unchanged, technological advancements, especially in materials, have opened doors we couldn't even imagine were possible. The advancements of this era have paves the way for major improvements in electrical infrastructure during the next century and beyond. Because we put a more substantial load on the electric grid every day, require increased computer processing, and push toward electric transportation, the redesign is essential. Due to their advanced and miniaturised technology, these devices and infrastructure generate a lot of heat, causing them to fail. In addition, these devices must be electrically remote controlled and weather- resistant[last ref.]. Electrical insulation is being developed around the globe in response to theever-increasing reliability and performance requirements as gadgets and infrastructure improve.Researchers from various Universities are analyzing new materials for electrical insulation, or packaging, that can remove heat more effectively compared to today's insulation.Focusing on one asset alone, which includes thermal conductivity, isn't sufficient to get the essential overall performance and lifespan from digital devices. We want to make sure that substances have big electric resistance, tolerance to severe temperatures,cappotential to address mechanical stress, and resistance to moisture, amongst differentthings. The grand assignment for substances builders is to enhance a lot of these homes simultaneously, rather than the contemporary one-at-a-time approach [20]. If we increase electric insulation in a holistic way, as researchers suggest, we will see enhancements in lots of elements of our lives.The paper is composed as follows: area II gives material modifications for improved performance of insulation like FGM, Materials with Improved Electrical Strength, Materials with Decreased Electrical Conductivity;segment III portrays Measurement Procedure for Dielectric Characterization of Outdoor Polymeric Materials,while segment V underlines the conclusion and future research scope.
II MATERIAL IMPROVEMENTS TO ENHANCE PERFORMANCE
A. Field Grading Materials
Electric field grading (FGM) sometimes also denoted as stress grading refers to the technique of efficiently and reliably controlling the electric field distribution within or around a device. The classification of electric fields in HV and EHV devices has evolved over the years from the application of simple geometries and homogeneous dielectrics with linear properties to complex systems using materials. The FGM concept was later transferred to cable ends and couplings to alleviate critical dielectric stresses. The use of FGM in the design of other electrical equipment including circuit breakers, bushings, instrument transformers, arresters, and insulators is also highly desirable today.
The number one feature of field grading material is that its electric conductivity will increase above a threshold electric field value[3]. This asset is then applied to lessen maximum field stress at crucial places in the insulation system. An FGM composite is made up of a base polymer (such as silicone rubber SIR, ethylene-propylene-diene monomer EPDM rubber, epoxy resin, or thermoplastic) with silicon carbide SiC, zinc oxide ZnO, or carbon black [3, 4, and 46]. However, the latest
studies have centered on the skills of nano-fillers, in particulargraphenenano-platelets (GnP) and graphene oxide (GO).These promise similar fieldgrading qualities with substantially lower filler loads and better material processing. Results produced by the usage of LDPE-GnP primarily based totally nano-composites, for example, display the traits that would be beneficial in enhancing the overall performance of polymer- primarily based totally insulation structures which include HVDC cable terminations.
Fig.1 Field dependency of electrical conductivity of LDPE &GnP
The area dependency of electrical conductivity for all samples studied is compared in Figure1. A crossover effect becomes obvious atafield strength of approximately 20 kV/mm, and the nonlinear behaviour replaces the linear behaviour. According to the results, filled nanocomposites had a lower conductivity than pure polymers at low fields and a higher conductivity at high fields. When graphene oxide was added to a polydimethylsiloxanematrix, similar behaviour was observed[op].
Permeability to various gases is a significant feature for usage in composite equipment insulators. Monitoring gas concentration in a closed volume of air at atmospheric pressurewas used to evaluate the penetration rates of SF6 and CO2 in produced nanocomposite materials [5, 6, and8 ]. The effect of adding GnP on permeation rates against filler content is seen in Figure 2. The size of gas molecules influences this behaviour.
Fig. 2 The effect of adding GnP on permeation rates against CO2 and SF6
B Electrical Strengthening Materials
Electrical treeing has been widely reported as a cause of degradation in cable and epoxy- based insulation systems. On the other hand, even if the materials in composite insulators exhibit similar behaviour, the occurrence of this same degrading process has been fairly rare. When developing composite apparatus insulators, where strong electrical stress applies radially across the insulator's walls, it is vital to consider a material's resistance to electrical treeing [17, 19, and 21]. Although the study used polyethylene (LDPE) as the polymer matrix, the conclusions are expected to be applicable to other polymer systems, including silicones, because the treeing processes observed are similar. The electrical tree initiation level in materials containing various types of stabilisers (e.g. fullerenes, benzil, melamine, and thioxanthone )was tested by the authors of [8]. The majority of chemicals tested had a beneficial influence on resistance to electrical treeing, with increases ranging from 30% to over 100% in the tree initiation field [op]. It was dscovered that the electron affinity of these stabilisers correlates well with their stabilising efficiency by connecting test results with their electrical inheritance [8]. This suggests that electron scavenging is the main driver of the improvement.
C. Electrical Conductivity-Reduced Materials
In particular, low electrical conductivity is a feature suitable for HVDC applications. In contrast to virgin polymer systems, which lack conductivity by nature, a nano-filler approach was used to improve conductivity. In the study, the findings were related to how they affectedelectrical conductivity[23, 24]. Electrical treeing resistance was also evaluated. The researchers investigated LDPE-based nano-composites comprising MgO, Al2O3, and ZnO inorganic particles, as well as graphene nanoparticles [26, 41,and46]. All nano-composites had much lower measured current levels than the reference material, indicating a deterioration in charge transfer as in Fig.3.[53]. The effect of nano-filler content on a material's electric conductivity is also demonstrated[53]. Other researchers have additionally received comparable results.
Fig.3 Time variation of charging current measured for reference LDPE and Al2O3[53]
With a filler content of 4% wt, the nano-composites investigated show a significant reductionin electrical conductivity[56].It appears that this effect is not related to the chemical nature of the nano-filler but rather to the formation of polymer-particle interfacial layers. These provide deeper charge trapping sites and reduce long-range molecular mobility.
III PROCEDURE FOR DIELECTRIC CHARACTERIZATION OF OUTDOOR POLYMERIC MATERIALS
Radio frequency measurement of complex dielectric properties of materials has grown in importance, especially in areas such as material science research. Dielectric dimension is essential due to the fact it is able to offer the electric or magnetic traits of the materials, whichhas proved beneficial in lots of studies and improvement fields [13].There are multiple methods available to measure these complex properties, including time-domain and frequency-domain methods, methods involving one or two ports, etc. Every method is limitedto specific frequencies, materials,and applications by its own constraints. These are known as frequency domain spectroscopy (FDS) measurements in the frequency domain, and they allow extracting the complex impedance of test objects under excitation by voltage signals of varying frequency[2]. FDS is employed in a range of applications, including dielectric material selection and development for electrical insulation applications, where exact knowledge of relative permittivity (r) and dissipation factor (tan )is required. Intricate electrode configurations, on the opposite hand, can impede material characterisation given that correct measurements necessitate electrodes that make good enough touch with check objects.
Placing measured specimens among metallic plates is the most effective scenario. However, specimen surfaces are hardly ever flawlessly easy and uniform in thickness. Because of the appearance of series resistance in the measurement circuit, areas with no direct contact between the electrodes and the surface form current channels along with the test specimen, resulting in a greater loss factor value[53]. Furthermore, in the case of soft-material specimens, the pressure produced by the electrode arrangement can cause deformation and hence affect the observed permittivity. Furthermore, complicated permittivity can be influenced by the series resistance of electrodes and cables, resulting in an additional loss peak at higher frequencies that covers the true relaxation process[53].
To ensure optimal material surface contact, various electrode configurations have been proposed and implemented, each with its own set of benefits and downsides. Deposition of conductive material at the specimen surface, along with steel or graphite painting, steel sputtering and evaporation, fired-on metal films, utilization of conductive glue or grease with metal foils, mercury, and NaCl water solution [53] are only some examples.All of these are standard answers, yet they all add to the level of uncertainty. Avoiding direct contact between the material and parallel plate metal electrodes is a reliable and straightforward way to avoid the contact problem in dielectric response characterization. In this way, the combined response of the specimen and a small air gap is measured. Further, the electrode edge effect has a geometric influence on the distribution of the electric field in this area, causing it to be distorted.
Fig. 4. HTVs electrical permittivity measured with contact and contact-free electrode
In the example of HTV silicone rubber, Figure 4 shows how the measuring process affects the estimation of material dielectric permittivity using contact-free and contact electrode designs[53]. As a result, the materials tested with the contact electrode configuration exhibit a distinct rise in actual permittivity as the testing temperature decreases. The contact measurement may have been influenced by the thermal expansion of the materials.
IV CONCLUSIONS
For more than a century, insulating materials have been developed. The development of insulating materials has been continuous since natural materials were discovered, then organic polymers, then pure polymers, and now nanocomposites, and the properties of these materials have been significantly improved[1.A].In light of current composite insulator technology, building reliable polymeric insulators and getting wide market adoption will take over 30 years. Researchers have previously focused on finding reliable materials for line insulators as well as persuading consumers that they will operate reliably in situations occurring outdoors. Other work was done in tandem to establish appropriate manufacturing technologies, develop insulator design criteria, and develop test procedures for the newconcepts. Polymeric station post and apparatus insulators, as well as elastomeric coverings, were developed later. As a result of all of this, composite insulation solutions are now widely used in power engineering.
The research will focus on the improvement of polymeric materials, particularly as equipment insulators and cable accessories for HVAC and HVDC applications. The purpose of these studies is to optimize the properties of polymeric materials with additives so that their field evaluation, bio-resistance, fracture strength, and DC conductivity can be improved.Additionally, it is crucial to develop suitable test methods for assessing the properties of thesenew materials.Global concerns regarding environmental protection and advances in electrical, thermal, and mechanical properties are driving rapid developments in insulating materials.
REFERENCES:
[1] S. Li, L. Zhong and J. Li, et al: 'A Brief History and Research Progress on Solid Engineering Dielectrics in China', IEEE Electrical Insulation Magazine, 2010, 26, (6),pp. 14-21 [2] Q.Lei:'RecentProgressofEngineeringDielectrics'(SciencePress,Beijing,1999,1stedn.) [3] Hayakawa, N.; Kato, K.; Okubo, H.; Hama, H.; Hoshina, Y.; Rokunohe, T.: Electric Field Grading Techniques in Power Apparatus Using Functional Materials. CIGRE Paris Session, D1-309, Paris, 2014 [4] Secklehner, M.; Hussain, R.; Hinrichsen, V.: Tailoring of new Field Grading Materials for HVDC Systems. INSUCON 2017,Birmingham, 2017
[5] T.Tanakaand T.Imai: 'Advancesinnanodielectric materialsoverthepast50 years',IEEEElectricalInsulation Magazine, 2013, 1, (29), pp. 10-23 [6] T. Tanaka: 'Dielectric nanocomposites with insulating properties', IEEE Transactions on Dielectrics and Electrical Insulation, 2005, 12, (5), pp.914-928
[7] B. Helgee and P. Bjellheim: 'Electric breakdown strength of aromatic polymers: dependence on film thickness and chemical structure', IEEE transactions on electrical insulation, 1991, 26, (6), pp. 1147-1152 [] K. Y. Lau, A. S. Vaughan and G. Chen: 'Nanodielectrics: Opportunities and challenges', IEEE Electrical Insulation Magazine, 2015, 31, (4), pp.45-54
[9] J. K. Nelson: 'Overview of nanodielectrics: Insulating materials of the future'. 2007 Electrical Insulation Conference and Electrical Manufacturing Expo, 2007, pp. 229- 235 [10] T.Tanaka, G. C. Montanari and R. Mulhaupt: 'Polymer nanocomposites as dielectrics and electrical insulation-perspectives for processing technologies, materialcharacterization and future applications', IEEE Transactions on Dielectrics and Electrical Insulation, 2004, 11, (5), pp. 763- 784 [11] J. J. O'Dwyer: 'Breakdown in solid dielectrics', IEEE Transactions on Electrical Insulation, 1982, (6), pp. 484-487 [12] S. Diaham, S. Zelmat and M. Locatelli, et al: 'Dielectric breakdown of polyimide films: Area, thickness and temperature dependence', IEEE Transactions on Dielectrics and Electrical Insulation, 2010, 17, (1), pp. 18-27M. Ieda: 'Dielectric breakdown process of polymers', IEEE Transactions on Electrical Insulation, 1980, (3), pp. 206- 224 [13] G.G.Raju:'Dielectricsinelectricfields'(CRCpress,2003) [14] X. Zhou, X. Zhao and Z. Suo, et al: 'Electrical breakdown and ultrahigh electrical energy density in poly (vinylidene fluoride- hexafluoropropylene) copolymer',Applied physics letters, 2009, 94, (16), p. 162901
[15] X. Zhou, B. Chu and B. Neese, et al: 'Electrical energy density and discharge characteristics of a poly (vinylidene fluoride-chlorotrifluoroethylene) copolymer', IEEE transactions on dielectrics and electrical insulation, 2007, 14, (5), pp. 1133- 1138
[16] N. R. Tuand K. C. Kao: 'High-field electricalconductioninpolyimide films', Journal of applied physics, 1999, 85, (10), pp. 7267-7275 [17] J. J. McKeown: 'Intrinsic electric strengths of organic polymeric materials', Electrical Engineers, Proceedingsof the Institution of, 1965, 112, (4), pp. 824-828 [18] P. Barber, S. Balasubramanian and Y. Anguchamy, et al: 'Polymer composite and nanocomposite dielectric materials for pulse power energy storage', Materials, 2009,2, (4), pp. 1697-1733 [19] Y. Wang, X. Zhou and Q. Chen, et al: 'Recent development of high energy density polymers for dielectric capacitors', IEEE Transactions on Dielectrics and Electrical Insulation, 2010, 17, (4), pp. 1036-1042 [20] B. Chu, X. Zhou and B. Neese, et al: 'Relaxor ferroelectric poly (vinylidene fluoride- trifluoroethylene-chlorofluoroethylene) terpolymer for high energy density storage capacitors', IEEE transactions on dielectrics and electrical insulation, 2006, 13, (5), pp. 1162-1169 [21] J. Wang, L. Zhong and S. Li, et al: 'Handbook of Electrical & Electronic Insulation Technology' (China Machine Press, Beijing, 2008, 1st edn.) [22] Y. P. Mamunya, V. V. Davydenko and P. Pissis, et al: 'Electrical and thermal conductivity of polymers filled with metal powders', European polymer journal, 2002, 38, (9), pp. 1887-1897 [23] A. Pablos, M. I. Osendi and P. Miranzo: 'Effect of microstructure on the thermal conductivity of hotpressed silicon nitride materials',Journal of the American Ceramic Society, 2002, 85, (1), pp. 200-206
[24] J. Zeng, R. Fu and Y. Shen, et al: 'High thermal conductive epoxy molding compound with thermal conductive pathway', Journal of applied polymer science, 2009, 113, (4),pp. 2117-2125 [25] M. Kozako, Y. Okazaki and M. Hikita, et al: 'Preparation and evaluation of epoxy composite insulating materials toward high thermal conductivity'. 2010 10th IEEE International Conference on Solid Dielectrics, 2010, pp. 1-4 [26] X.Huang,P.JiangandT. Tanaka:'Areviewof dielectricpolymercompositeswithhigh thermalconductivity',IEEEElectricalInsulationMagazine,2011,27,(4),pp.8-16 [27] C. Zhi, Y. Bando and T. Terao, et al: 'Towards thermoconductive, electrically insulating polymeric composites with boron nitride nanotubes as fillers', Advanced Functional Materials, 2009, 19, (12), pp. 1857-1862 [28] Y.Xu,D.ChungandC.Mroz:'Thermallyconductingaluminumnitridepolymer-matrixcomposites',CompositesPartA:Appliedscienceandmanufacturing,2001,32,(12),pp. 1749-1757
[29] W. Peng, X. Huang and J. Yu, et al: 'Electrical and thermophysical properties of epoxy/aluminum nitride nanocomposites: Effects of nanoparticle surface modification', Composites Part A: Applied Science and Manufacturing, 2010, 41, (9), pp. 1201-1209 [30] R. Qian, J. Yu and C. Wu, et al: 'Alumina-coated graphene sheet hybrids for electrically insulating polymer composites with high thermal conductivity', RSC Advances, 2013, 3, (38), pp. 17373-17379 [31] I. Tseng, J. C. Chang and S. L. Huang, et al: 'Enhanced thermal conductivity and dimensional stability of flexible polyimide nanocomposite film by addition of functionalized graphene oxide', Polymer International, 2013, 62, (5), pp. 827-835 [32] D. Kumluta, I. H. Tavman and M. T. Çoban: 'Thermal conductivity of particle filled polyethylene composite materials', Composites science and technology, 2003, 63, (1),pp. 113-117 [33] W. Zhou, S. Qi and C. Tu, et al: 'Studyof insulating thermal conductive polymer composites', Chin. Plast. Ind, 2005, 33, p. 99 [34] J. K. Seong, I. J. Seo and J. S. Hwang, et al: 'Comparative evaluation between DC and AC breakdown characteristic ofdielectric insulating materials in liquid nitrogen',IEEE Transactions on Applied Superconductivity, 2012, 22, (3), p. 7701504-7701504
[35] A. Bulinski and J. Densley: 'High voltage insulation for power cables utilizing high temperature superconductivity', IEEE Electrical Insulation Magazine, 1999, 15, (2), pp. 14-22 [36] Y.P.Teng,L.Y.XiaoandS.T.Dai:'InsulationofHTSPowerCableandPropertiesofTheInsulati on',InsulatingMaterials,2005,pp.59-64 [37] M. Kosaki: 'Research and development of electrical insulation of superconducting cables by extrudedpolymers', IEEE Electrical Insulation Magazine, 1996, 12, (5), pp. 17-24 [38] W. Zhixuan, Q. Jie and W. Zhaozuo, et al: 'Summary of dielectric material testing for CD HTS cable', Cryogenics and Superconductivity, 2008, 12, [39] Q.Shu:'SuperconductingEngineering'(ChinaMachinePress,Beijing,1989,1stedn.) [40] R. S. Gorur, J. Montesinos and L. Varadadesikan, et al: 'A laboratorytest for tracking and erosion resistance of HV outdoor insulation', IEEE Transactions on Dielectrics and Electrical insulation, 1997, 4, (6), pp. 767-774 [41] H. Li, G. Liu and B. Liu, et al: 'Dielectric properties of polyimide/Al2O3 hybrids synthesized by in-situ polymerization', Materials Letters, 2007, 61, (7), pp. 1507- 1511 [42] M. Katz and R. J. Theis: 'New high temperature polyimide insulation for partial discharge resistance in harsh environments', IEEE Electrical Insulation Magazine, 1997, 4, (13), pp. 24-30 [43] V. Rajini and K. Udayakumar: 'Degradation of silicone rubber under AC or DC voltages in radiation environment', IEEE Transactions on Dielectrics and Electrical Insulation, 2009, 16, (3), pp. 834-841 [44] M. Billings, A. Smith and R. Wilkins: 'Tracking in polymeric insulation', IEEETransactions on Electrical Insulation, 1967, 3, (EI-2), pp. 131-137 [45] C. Calebrese, L. Hui and L. S. Schadler, et al: 'A review on the importance of nanocomposite processing to enhance electrical insulation', IEEETransactions on Dielectrics and Electrical Insulation, 2011, 18, (4), pp. 938-945
[46] I. Ramirez, E. A. Cherney and S. Jarayam: 'Comparison of the erosion resistance of silicone rubber and EPDM composites filled with micro silica and ATH', IEEETransactions on Dielectrics and Electrical Insulation, 2012, 19, (1), pp. 218-224 [47] S.Singhaand M.J.Thomas:'Dielectricropertiesofepoxynanocomposites',IEEETransactionsonDielec trics and Electrical Insulation, 2008, 15, (1),pp. 12-23
[48] T. Imai, F. Sawa and T. Ozaki, et al: 'Effects of epoxy/filler interface on properties of nano-or micro-composites', IEEJ Transactions on Fundamentals and Materials. A, 2006, 126, (2), pp. 84-91 [49] J. Y. Li, L. Zhang and S. Ducharme: 'Electric energy density of dielectric nanocomposites', Applied physics letters, 2007, 90, (13), p. 132901 [50] J. K. Nelson and J. C. Fothergill: 'Internal charge behaviour of nanocomposites',Nanotechnology, 2004, 15,(5), p. 586
[51] P.C.Irwin,Y.CaoandA.Bansal,etal:'Thermalandmechanicalpropertiesofpolyimidenanocomposites', ElectricalInsulationandDielectricPhenomena.Annual ReportConference,2003,pp.120-123
[52] Z. Han, C. Diao and Y. Li, et al: 'Thermal properties of LDPE/silica nanocomposites'. 2006 IEEE Conference on Electrical Insulation and Dielectric Phenomena, 2006, pp. 310-312 [53] https://www.inmr.com/materials-development-insulators/ [54] P.Maity,S.Basuand V.Parameswaran,etal:'Degradation ofpolymerdielectricswithnanometric metal-oxide fillersduetosurfacedischarges',IEEETransactionsonDielectricsandElectricalInsulation, 2008,15,(1),pp.52-62 [55] S.Zou:'DevelopmentTrendinPolyimide',NewChemicalMaterials,1999,27,(3),pp.3-6 [56] H. LI, L. Guo and B. Liu, et al: 'A study of dielectic properties of polyimide/nano-titanium/dioxide composites films', Insulating Materials, 2005, 6, pp. 30-33