
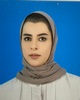
- Open Access
- Authors : Manal Ali , Hamada Esmaiel
- Paper ID : IJERTV10IS110007
- Volume & Issue : Volume 10, Issue 11 (November 2021)
- Published (First Online): 31-12-2021
- ISSN (Online) : 2278-0181
- Publisher Name : IJERT
- License:
This work is licensed under a Creative Commons Attribution 4.0 International License
Improving the Energy and Spectral Efficiency of LiFi Systems using Companding Techniques
Manal Ali, Hamada Esmaiel
Electrical Engineering Department,
Faculty of Engineering, Aswan University, 81542 Aswan, Egypt.
Abstract:- A novel digital companding technique is proposed for light fidelity (LiFi) based on orthogonal frequency division multiplexing (OFDM). The technique builds on the principles of nonlinear companding. During this study, the performance of the two main companding transforms (-law, nonlinear exponential) are compared in terms of PSD with prismal shaped companding as well as BER of all of them. Orthogonal frequency division multiplexing (OFDM) signals suffer from the problem of a high peak-to-average power ratio (PAPR) that complicates the design of the analog front-end of the system which will be analyzed and improved. Simulation is done by using optimum parameters that achieve efficient PAPR reduction for each companding transform and BER also. Both PAPR and moderate performance degradation are critical challenges for a well-designed companding scheme. To obtain efficient PAPR reduction in OFDM systems, this paper proposes a new NCT scheme.
Keywords: LiFi, Nonlinear Companding, OFDM
-
INDEX TERMS
Orthogonal Frequency Division Multiplexing (OFDM), Peak to Average Power Ratio (PAPR), Bit Error Rate (BER), Visible Light Communications (VLC)
-
INTRODUCTION
The wireless technology relying on radio waves has become an integral part of our everyday life, facilitating basic services, such as making a phone call or sending a message, as well as nearfield-communication-aided mobile payment. The radio waves occupy the 3 kHz300 GHz electromagnetic band, which has been widely used for communication purposes. However, with the increase of tele-traffic, as recently predicted by Cisco and shown in Figure 1, the radio frequency (RF) band is becoming more and more crowded. Following the launch of the global fifth-generation (5G) research initiatives [3] conceived for tackling the explosive escalation of wireless tele-traffic, the horizon of communication bands has been expanded from the conventional RF band both to the millimeter-wave [4] and to the visible light [1] frequency band spanning from 400 to 800 THz. The earliest known use of visible light communications (VLC) dates back to 1880 when Bell developed photophone techniques and their applications in their early development phase. Tsonev et al. [5] discussed some of the key networking techniques required for realizing light fidelity (Li-Fi) networks, including the associated multiple access techniques, the potential uplink solutions as well as the optical attocell, and overall network structure.
Sevincer et al. [6] introduced the concept of the so-called LIGHTNETs, which represented the dual function of smart lighting and optical wireless (OW) communications, with special emphasis on the inherent design tradeoffs between these two functions.
There is a clear difference between visible light communications (VLC) and LiFi. LiFi takes VLC further by using light-emitting diodes (LEDs) to realize fully networked wireless systems. Synergies are harnessed as luminaries become LiFi attocells resulting in enhanced wireless capacity providing the necessary connectivity to realize the Internet-of- Things, and contributing to the key performance indicators for the fifth generation of cellular systems (5G) and beyond [7]
LiFi leverages the visible light spectrum that is 1000 times larger than the entire 300 GHz of RF spectrum. The visible light spectrum enables a huge, step-change growth in future data rates, future-proofing wireless networks for the continued exponential growth in demand for wireless data. Intensive system analyses show that LiFi attocellular networks will provide superior user data rates [8]. pureLiFi and the University of Edinburgh have shown that in the medium term LiFi light bulbs can achieve 100 Gbps, over 14 times higher than WiGig, reaching over 1 Tbps in the long term (1 Tbps) by exploiting the huge visible light spectrum resource [9]. This means LiFi technology will be able to deliver the predicted access point data rates. In addition, the atto-cell network of LiFi-enabled lights can deliver data density (Mbps / sqm) that is over 1000 times greater than the state-of-the-art wireless systems.
In addition to increased wireless capacity, LiFi attocellular networks have the potential to be three orders of magnitude more energy-efficient as they are much more efficient in serving multiple users in dense users/devices/things deployments. With the coming of the IoT to prominence, as well as mounting environmental concerns, delivering an energy-neutral communication system is crucial. LiFi has the potential to deliver on this front as well. Recent work [9] has shown that solar panels can be used as signal detectors for LiFi, which enables simultaneous data communication and energy harvesting. With plentiful energy available for scavenging in the form of solar radiation, this is an enabler for the creation of simple and cheap IoT devices that can interface to LiFi networks without generating cost in terms of operational energy. Moreover, with tens of Mbps communication speeds possible [10], solar-enabled LiFi can
deliver connectivity to remote and/or undeveloped areas of the world in a sustainable and infrastructure-independent manner. This presents a solution that brings remote settlements into the connected world without the need for enormous capital expenditure. Finally, with the recent advances in flexible circuit technology and organic flexible solar cells, one can
average power transmitted leading to a decrease in the power efficiency and degrading the bit error rate (BER) performance as well as they require more implementation complexity.
The power spectral density (PSD) of a discrete random process of FBMC signal, is given in the following equation:
S(f)=|G(f)|2 A(( ) (1)
envision almost infinite applications of this technology. 2
Virtually any surface be it solid or flexible could potentially be network connected via LiFi and serve as a sensor host or a smart agent.
The Bit Error Rate or ratio (BER) is defined as the number of bit errors divided by the total number of transferred bits during a studied time interval. BER is a unitless performance measure, often expressed as a percentage number. In a Communication System, the receiver side BER may be affected by the transmission channel noise, interference, distortion, bit synchronization problems, attenuation, wireless multipath fading, etc. The BER may be improved by choosing strong signal strength or by choosing a slow and robust modulation scheme or by line coding scheme or by channel coding schemes. The transmission Bit Error Rate (BER) is the number of detected bits that are incorrect before error correction, divided by the total number of transferred bits (including redundant error codes). The information Bit Error Rate (BER), approximately equal to the decoding error probability, is the number of decoded bits that remain incorrect after the error correction, divided by the total number of decoded bits (the useful information). Normally the transmission BER is larger than the information BER. The information BER is affected by the strength of the forward error correction code.
-
QUADRATURE AMPLITUDE MODULATION SYSTEM AND POWER SPECTRAL DENSITY
In the multicarrier transmission, the subcarriers are independent of each other in the time domain such that their composite signal has a large dynamic range as subcarriers may align to produce cnstructive or destructive superposition. Constructive superposition will result in a signal with high values of envelope peaks whereas destructive superposition may fade the signal completely. Such large variation in signal power is measured in terms of peak to average power ratio [10]. Filter Bank Multicarrier with Offset Quadrature Amplitude Modulation System (FBMC/OQAM) is one of the many new waveforms is proposed for the next wireless communication system (5G). The FBMC system is a multicarrier modulation scheme with relaxed orthogonally, increased frequency efficiency, improved shape, and low out- of-band interference inherent in FBMC/OQAM techniques.
The main drawback of the FBMC/OQAM system is a high Peak to Average Power Ratio (PAPR) of the transmitted signal. To overcome that, many researchers proposed schemes to reduce PAPR for the FBMC system [2]. Active Constellation Extensions (ACE) with projection onto convex sets (POCS) and smart gradient projection (SGP) are used for reducing the PAPR of the FBMC system. But they increase the
where the value of A(( ) is the PSD of the signal, G(f) is
2
the Fourier transform of the prototype filter g{n}, and f is the
normalized frequency with respect to fs or the sampling frequency.
-
BASICS OF COMPANDING METHODS
To implement the OFDM for high data rate transmission, a noticeable increase in cost and complexity is added to the mobile satellite systems. The main advantages of implementing such the OFDM transmission scheme are first, OFDM is an efficient technique for eliminating the multipath distortion; the complexity of channel equalization in OFDM systems is significantly lower than that of single-carrier systems. As a result of the narrow bandwidth of each sub- channel, the channel distortion in OFDM systems can be eliminated by utilizing one-tap frequency-domain equalizers, secondly, OFDM is robust against the narrowband interference; the interference of the subcarriers increases only by a small percentage due to narrowband interference. Finally, the OFDM is attractive for broadcasting applications because it makes single frequency networks possible.
Companding is a well-known technique that reduces the PAPR using a deterministic amplitude transform, with the assumption that the OFDM signal amplitude is Rayleigh distributed. The most popular methods of companding are µ- law and A-law Companding schemes.
The orthogonal frequency division multiplexing (OFDM) technique has been widely adopted in modern wireless communications due to its high spectral efficiency and immunity to the multipath fading effects. However, a critical drawback of the OFDM system is the high peak-to-average power ratio (PAPR) of the transmitted signals. The high PAPR results in the in-band distortion and out-of-band interference when the nonlinear power amplifier (HPA) is utilized at the transmitter. Various PAPR reduction methods have been proposed for OFDM systems over the past decade [3], among which, the nonlinear companding transform (NCT) is perhaps the most attractive and significant method owing to its effectiveness and simplicity. NCT belongs to the category of signal pre-distortion solutions for PAPR reduction and can be employed straightforwardly without any restriction on the OFDM system parameters.
– Mu-law companding technique
The µ-law is an effective companding technique that can be used to reduce PAPR. In the µ-law, a compressor characteristic is a linear form for low-level inputs and a logarithmic form for high-level inputs. µ-law compression signal at the transmitter is expressed mathematically as:
(2)
where µ is the command parameter, x(t) is the input instantaneous amplitude, and xmax(t) is the peak amplitude of x(t).
In this technique, the small amplitudes of the signal are enlarged so the difference between the peaks and small values is reduced The mathematical formulation for the output of µ- law companding and decompanding algorithms are D(x) and D(r) respectively:
Fig.1 block diagram of OFDM system using non-linear companding method.
() = log(1+)
log (1 + ||) sgn(), (3)
(5)
The input information symbols are assumed to be
() =
(
|| log(1+)
1), (4)
statistically independent and identically distributed. If N was larger (e.g. N 64), the real and imaginary parts of sn, denoted
where x is the baseband OFDM signal, r is the received
signal after the channel, is the maximum amplitude of the signal x, µ is the companding level, and sign(.) is the signum function.
Nonlinear Companding is a special type of clipping scheme used to provide great PAPR reduction with better BER performance and less implementation complexity required to build the system. The companding transforms enlarge the small signals while compressing the large signals to increase the immunity of small signals from noise and interference. The companding function is applied at the transmitter to compand the output signals using strict monotone function and at the receiver, the inverse companding function is used to recover the signal in the receiver.
The nonlinear Companding is one of the best PAPR reduction techniques used in multicarrier modulation due to less complexity and good bit error rate BER. The companding function is used in order to attenuate the high peaks and amplify the low amplitudes. At the receiver, the inverse companding function is applied in order to recover the original signal. Moreover, by choosing proper companding parameters, the average transmitted power can be kept unchanged after companding. In this paper, we used different nonlinear companding techniques to reduce the PAPR of the FBMC system. The companding techniques used are hyperbolic tangent (tanh), error function (erf), Logarithmic Function (log), Mu-law, and A-law companding.
The nonlinear companding techniques proposed in the following section have the block diagram of a typical OFDM system using the non-linear companding technique for PAPR reduction. Let N denote the number of sub-carriers used for parallel information transmission and let Sk (0 k N 1) denote the kth complex modulated symbol in a block of N information symbols. The outputs Sn of the N-point Inverse Fast Fourier Transform (IFFT) of Sk are the OFDM signal samples over one symbol interval, or mathematically
by Re{sn} and Im{sn}, are independent and identically distributed Gaussian random variables with zero mean and a common variance 2 = E |Sk|2/2, according to the central limit theorem. The amplitude, or modulus, of OFDM signal Sn is given by:
, (6)
The amplitude |sn| has a Rayleigh distribution with the Cumulative Distribution Function (CDF) is as follow:
(7)
The power of OFDM signal Sn can be calculated by:
, (8)
The value of peak power occurs when N modulated symbols are added with the same phase. By using the non-linear companding technique, the OFDM signals sn are companded before they are converted into analog waveforms and amplified by the HPAs. The companded signal tn (0 n N
1) is given by:
tn=h(Sn)
The function changes only the amplitudes of input signals. Then OFDM signals are transmitted into the radio channel. Consider an Additive White Gaussian Noise (AWGN) channel, the received signals rn after the analog-to-digital (A/D) conversion would be:
= + = () + , (9)
-
LITERATURE REVIEW
To address the high PAPR problem of OFDM, many techniques have been proposed to reduce the high PAPR of the OFDM signals. Techniques such as clipping and filtering, block coding, and nonlinear companding
transform (NCT) have been considered. Moreover,
Wong et al. proposed PAPR reduction by switching null subcarriers and data subcarriers [12]. This method utilizes the null subcarriers that do not transmit data but energy. The
transmitter searches for the data subcarriers that would lead to the highest PAPR reductionand the null subcarriers are switched with the selected data subcarriers, thereby achieving high PAPR reduction.
A selective mapping (SLM) based PAPR reduction technique was proposed in [13] with reduced complexity and error performance. Conventionally, in the SLM, a data sequence is multiplied with a series of phase rotation vectors, and the product is processed by a set of IFFT operations, and the signal with the lowest PAPR is selected to be transmitted.
It also proposes a low complexity PAPR estimation method using search-and-partial-interpolation (SPI) and it was contended in [13] that the proposed SPI combined with the SLM reduces the complexity of the conventional SLM process.
Kai et al. addressed the issue by assigning PAPR with a time-varying number of subcarriers to the OFDM signal [14]. The basic idea of this scheme is that it divides the data symbols into several numbers of sub-blocks and input the sub-blocks into a set of IFFT blocks. The number of subcarriers assigned to the sub-blocks at a time is determined by an algorithm based on the PAPR of the output signal of the IFFT. Kai proposed that the division of the symbol stream into sub-blocks leads to a shorter IFFT operation than the classical OFDM, thereby resulting in a relatively lower PAPR. Although this scheme shows a reduction of PAPR, it increases the computation complexity because of the set of IFFT and fast Fourier transform (FFT) blocks used.
In [15], Ryu et al. presented a dummy sequence insertion (DSI) method to reduce the PAPR and they claimed that it is simpler than the SLM method and spectrally efficient. Also, it shows that DSI results in a better error performance than SLM since the inserted sequence does not work as side information.
The techniques mentioned above offer attractive features but suffer from some drawbacks. Nevertheless, clipping and NCT methods are largely considered simpler and
easily applicable techniques than others. In clipping, however, high signal peaks are cut at some power level, so that the distorted signal cannot be recovered. On the other hand, NCT is derived in such a way that small signal amplitudes are expanded while large signal amplitudes are compressed at the same time. Therefore, NCT is more advantageous than clipping, since the signal distortion can, to some extent, be removed by applying the inverse transform function to the received signal. Also, the average power of the OFDM signal can remain unchanged during the companding transformation.
-
PROPOSED COMPANDING TECHNIQUE The nonlinear companding method can reduce the PAPR of
transmitted (companded) OFDM signals by transforming the statistics of the amplitudes of these signals into a uniform distribution. The proposed scheme changes control parameters where the degree of freedom to control non-linearity was increased, resulting in effective PAPR reduction capability and decreasing signal distortions of the designed system. The proposed companding scheme changes only the magnitude of
the input signal keeping the average power constant. The focus of the proposed companding function is to transform the dth power of the magnitude of Rayleigh distributed OFDM signal into the prismal shaped distribution. dis said to be the degree of prismal shaped companding scheme. Distribution for the proposed companding scheme is illustrated in fig.2. the function has a maximum value when z=0 where f(z)=a and decreased by increasing z up to reach minimum value when
|z|=hp where f(z) is:
, (10)
Fig.2 Distribution for the proposed scheme
The distribution of the original OFDM signal transforms into the trapezoidal-shaped distribution in the interval [0, hp], according to the condition (hp>0).
The parameter, a, is used to specify the forms of the trapezium distribution, where 0 1 . The CDF of the
proposed scheme is given as:
, (11)
The relationship between FY(y) and Fp(z) is given by: FY(y)=Pr(Y<y)=Cp(Y)<Cp(y) = ( ()), (12)
The companding function of the proposed prismal scheme will be found by:
, (13)
By substituting the above equations to obtain companding function as follows:
, (14)
The companding function has an inverse transform function and restricted to a monotonically increasing function, becomes decompanding function. Thus, the decompanding function of the proposed scheme is found to be:
, (15)
To make the power of the companded signal the same as that of the original OFDM signal. The power of the companded signal can be represented as:
, (16)
Where the value of hp is represented as:
, (17)
Knowing that a=q/hp, then by substitution the companding function will be:
To compare the results with the original mu companded signal which was illustrated in fig.3 illustrating the difference in PSD of both techniques.
nonlinear companded mu companded
nonlinear companded mu companded
20
Power Spectral Density (dB/ rad/sample)
Power Spectral Density (dB/ rad/sample)
15
10
5
0
-5
-10
, (18)
And can be re-written to obtain the companded signal as:
-15
-20
-60 -40 -20 0 20 40 60
frequency
Fig.4 Power Spectral Density (dB/ rad/sample) VS frequency for both nonlinear and µlaw companded signals
, (19)
The companded signal, that composed of an attenuated signal component and companding noise vn can be represented as :
Sn= xn+vn.
Where the function is given by:
, (20)
The OFDM system uses companding schemes through AWGN and multipath channels as shown above work. The effect caused by the channel distortion after compensating, the received signal can be expressed as:
rn =sn+ n. (21)
-
PERFORMANCE EVALUATION
To verify the performance of the nonlinear companding scheme in the reduction of power or being energy efficient, The spectrums of original OFDM signals and companded signals were plotted as shown below:
20
Power Spectral Density (dB/ rad/sample)
Power Spectral Density (dB/ rad/sample)
15
10
5
0
-5
-10
-15
Due to the high PAPR, original OFDM signals have a very sharp, rectangular-like power spectrum (in Fig. 4). This good property will be affected by the PAPR reduction schemes, e.g. slower spectrum roll-off, more spectrum side lobes, and higher adjacent channel interference.
PAPR reduction capability: This is the most important factor in choosing a PAPR reduction technique. Some techniques show the result in other harmful effects. For example, the amplitude clipping technique removes the time domain signal peaks but results in in-band distortion and out- of-band radiation. The power increase in transmitting a signal: Some techniques require a power increase in the transmit signal after using PAPR reduction techniques. For example, Tone Reservation (TR) requires more signal power because for the PRCs.
Tone Injection (TI) uses a set of equivalent constellation points for an original constellation some of its power must be used point to reduce PAPR. The all equivalent constellation points original constellation point, the transmit signal will have more power after applying TI.
BER increase at the receiver: Some techniques may have an increase in BER at the receiver if the transmit signal power is fixed or require larger transmit signal power to maintain the BER after applying the PAPR reduction technique. For example, the BER after applying ACE will be degraded if the transmit signal power is fixed. In SLM, PTS, and interleaving, the entire data block may be lost if the side information is received in error and increase the BER at the receiver. The resulted BER of analysis was as shown in the following figure where the performance was plotted over a wider range of SNR.
-20
-60 -40 -20 0 20 40 60
frequency
Fig.3 Power Spectral Density (dB/ rad/sample) VS frequency
0 BER performance different companding over range of SNR
10
qpsk 16qam
prismal
-5
Bit Error Rate
Bit Error Rate
10
IX. REFERENCES
-
L. Hanzo, H. Haas, S. Imre, D. OBrien, M. Rupp, and L. Gyongyosi, Wireless myths, realities, and futures: From 3G/4G to optical and quantum wireless, in Proceedings of the IEEE, vol. 100, May 2012, pp. 18531888.
-
A. Goldsmith, Wireless communications. Cambridge University Press, 2005.
-
J. Andrews, S. Buzzi, W. Choi, S. Hanly, A. Lozano, A. Soong, and
J. Zhang, What will 5G be? IEEE Journal on Selected Areas in Communications, vol. 32, no. 6, pp. 10651082, June 2014.
-
S. Rangan, T. Rappaport, and E. Erkip, Millimeter-wave cellular
-10
10
-15
10
0 5 10 15 20 25
SNR
Fig.5 BER of companding techniques over a wide range of SNR
-
-
CONCLUSIONS
wireless networks: Potentials and challenges, Proceedings of the IEEE, vol. 102, no. 3, pp. 366385, Mar. 2014.
-
A. G. Bell, W. G. Adams, Tyndall, and W. H. Preece, Discussion on the photophone and the conversion of radiant energy into sound, Journal of the Society of Telegraph Engineers, vol. 9, no. 34, pp. 375 383, 1880.
-
D. Tsonev, S. Videv, and H. Haas, Light fidelity (Li-Fi): towards alloptical networking, in SPIE OPTO. International Society for Optics and Photonics, Feb. 2014, pp. 900 7021900 70210.
- [3] H. Haas, L. Yin, Y. Wang, and C. Chen, "What is LiFi?," in Journal of Lightwave Technology , vol.PP, no.99,
-
C. Chen, D. A. Basnayaka, and H. Haas, "Downlink Perormance of Optical Attocell Networks," in IEEE Journal of Lightwave
Generally, companding techniques expand small signals while compressing large signals or compress large signals without affecting small signals. In this paper, different PAPR reduction companding transforms are compared.
The prismal shape transform is an effective technique in reducing the PAPR of OFDM signals. In addition, the schemes based on the companding technique have low implementation complexity and no constraint on modulation format and sub- carrier size. In this paper, we have proposed and evaluated a new non-linear companding technique based on trapezoidal or prismal shaped function. It can adjust the amplitudes of both large and small input signals, while maintaining the average power unchanged by properly choosing transform parameters, to make the output signals have a uniform distribution. Simulation results have shown that the proposed companding schemes could offer better system performance in terms of PAPR reduction, BER performance, power spectrum than the
µ-law companding or original nonlinear schemes.
Technologies, vol. 34, no. 1, pp. 137-156, Jan. 2016.
-
Dobroslav Tsonev, Stefan Videv, and Harald Haas, "Towards a 100 Gb/s visible light wireless access network," Opt. Express 23, 1627- 1637 (2015)
-
Z. Wang, D. Tsonev, S. Videv and H. Haas, "On the Design of a Solar-Panel Receiver for Optical Wireless Communications With Simultaneous Energy Harvesting," in IEEE Journal on Selected Areas in Communications, vol. 33, no. 8, pp. 1612-1623, Aug. 2015.
-
Jeng, S., & Chen, J. (2011, June). Efficient PAPR reduction in OFDM systems based on a companding technique with trapezium distribution. IEEE Transactions on Broadcasting, 57(2), 291298. doi: 10.1109/TBC.2011.21122
-
K. T. Wong, B. Wang, J. C. Chen. OFDM PAPR reductionby switching null subcarriers and data-subcarriers. Electronics Letters, 2011, 47(1): 6263.
-
C. L.Wang, S. J. Ku, C. J. Yang. A low-complexity PAPR estimation scheme for OFDM signals and its application to SLMbased PAPR reduction. Journal of Selected Topics in Signal Processing, 2010, 4(2): 637645.
-
K. Shao, Z. G. Guo, L. Zhuang, et al. An effective scheme for PAPR reduction of OFDM signals using time-varying subcarrier Proc. of the IEEE International Conference on Signal Processing,
-
Communication and Computing, 2013: 16. F. J. Richards. A flexible growth function for empirical use. Journal of Experimental Botany, 1959, 10(20): 290301