
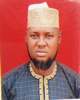
- Open Access
- Authors : Oyelakin. M. A, Olahan. A. B, Komolafe. T. F, Abdulazeez. K. B, Ajide. S. O
- Paper ID : IJERTV10IS070290
- Volume & Issue : Volume 10, Issue 07 (July 2021)
- Published (First Online): 11-08-2021
- ISSN (Online) : 2278-0181
- Publisher Name : IJERT
- License:
This work is licensed under a Creative Commons Attribution 4.0 International License
Improvement of Engineering Properties of Sub-Grade Soil with Locust Bean Waste ash and Quarry Dust
*Oyelakin, M. A.1, Olahan, A. B. 1, Abdulazeez, B. K.2, Komolafe, T. F. 1, Ajide, S. O. 1, Adeyemi, A. O1
1Department of Civil Engineering, Federal Polytechnic, Offa, Nigeria
AbstractThe experimental program was designed to study the effect of locust bean waste ash admixed with quarry dust on the index and strength properties of lateritic soil. The percentage mixtures of QD/LBWA were 0, 2, 4, 6, and 8% by dry weights of the soil, respectively. The soil sample used for was obtained along Ajase-Ipo interchange of Ilorin-Offa and Ilorin-Oro Road, Kwara State, Nigeria. The preliminary investigations conducted on the soil sample indicate that the soil is highly plastic with about 71% of the soil particles passing the B. S. No 200 sieve. The plasticity index values for all the concentration of the additive exceeded the 30% value prescribed for sub-grade materials by the Nigeria General Specification (1997). The peak MDD values recorded for BSL, WAS and BSH compactive efforts are respectively 1.50, 1.68 and 1.72Mg/m3 at 6% OPC/6% LBWA treatment. The OMC, on the other hand, decreased with higher compactive efforts but increased with higher LBWA contents. The unconfined compressive strength (UCS) values for natural soil compacted with BSL, WAS and BSH energies at 7 days curing period are 47, 390 and 750kN/m2 respectively and increased to 586, 1125 and 1240kN/m2 at 6% QD/6% LBWA treatment. In conclusion, the optimum blend of 6% QD/6% LBWA treatment on the lateritic is to be used as sub-base material when compacted with the British Standard heavy energy.
Keywords: Curing; Lateriti Soil; Locust Bean Waste Ash; Quarry Dust; Sub-grade
-
INTRODUCTION
To the civil engineer, soil is any uncemented accumulation of mineral particles formed by weathering of rocks. Soils are generally used as foundation or as construction materials. A class of soil, known as expansive soils, when used as foundation material, is usually affected by environmental conditions and they undergo detrimental volumetric and hydraulic conductivity changes because of the variation in moisture contents. Expansive soils are, therefore, soils with potential for shrinking or swelling under changing moisture condition [1]. These soils cause more damage to structures, particularly light building and pavements, than any other natural hazards, including earthquakes and floods [2].
The subgrade soil is the underlying soil on any ground surface and it must be able to support loads transmitted from pavement structure without excessive deformation under adverse climatic and traffic conditions. It is an established fact that not all soils possess the desirable qualities for use as pavement material. Additionally, a site where ground water table is high, the strength of such
subgrade is also adversely affected by moisture infiltration due to capillary action. When such soils cannot be replaced, their subgrade performance could be improved by several modification techniques [3]; [4]; [5]; [6].
Deficient soils are soils with potential for shrinking or swelling under changing moisture condition [1]. They contain more of montmorillonite with subsequent manifestation of swell properties and expansive tendencies [7]. They are poor and undesirable for engineering purposes but their properties could be improved by modification/stabilization processes to reduce the swell shrink tendencies of the soil and also makes the soil less plastic ([7]; [8]; [5]; [9].
Pozzolanas are siliceous and aluminous industrial or agricultural waste materials that possess little cementituous value but, will, in the presence of moisture chemically react with calcium hydroxide at ordinary temperature to form compounds possessing cementituous properties. Recent studies [10]; [5]; [11]; [12]; [9]; [13];
[14]; [16] [17]; [18]; [19], have focused on the use of these materials in the improvement of deficient soils. Additionally, the use quarry dust dates back the beginning of the century and in a recent research by [20], it was observed that the use of quarry dust increased the dry density of a deficient soil by 20% and decreased the moisture content by 15% -
MATERIALS AND METHODS
-
Materials
-
Lateritic Soil: The soil sample used for the research was obtained along Ajase-Ipo interchange of Ilorin- Offa and Ilorin-Oro Road, Kwara State, Nigeria. The top soil was removed to a depth of 0.5 before the soil samples were taken. They were sealed in plastic bags to avoid loss of moisture and taken to the laboratory. The soil samples were air dried before pulverizing to obtain particles passing BS sieve 4.75mm
-
Quarry Dust: The quarry dust used for the work was obtained from Bora Garden along Offa-Ajase road close to Ome-Green Park, Ijagbo, Kwara State. The materials were kept properly in a closed container and air-dried before passing through BS sieve no. 4.75mm
-
Locust Bean Waste Ash: The locust bean waste ash (LBWA) used was obtained locally from burning the husks sourced from Igbawere village along Offa-Ojoku road, Offa, Kwara State. The dried husks were completely burned at a temperature of 1000oC with Murfle furnace at Science Laboratory Technology, SLT, department, Federal Polytechnic, Offa. The ash was passed through BS sieve no. 850um before it was admixed in the appropriate percentages
conducted in accordance with Test 1[21]. Part 2 for the natural soil and [23] for the stabilized soils
7) Compaction characteristics
Maximum dry density
The compaction tests were carried out for the natural soil and the stabilized soils (in different percentages); all according to [21], using the British Standard Light, West African Standard and the British Standard Heavy, in accordance with the Nigerian General Specification (1997)
The bulk density in Mglm3 was later calculated for each compacted layers using:
-
-
Methods
m2 m1
The experimental program was designed to study the effect of locust bean waste ash admixed with quarry dust on the index and strength properties of lateritic soil. The percentage mixtures of QD/LBWA were 0, 2, 4, 6, and 8% by dry weights of the soil, respectively.
-
Natural Moisture content: The natural moisture content of the soil as obtained from the site was determined in accordance with [21]. The natural moisture content (as collected from the site) is calculated as the average as given by eq. (3.1)
w M 2 M 1 100
M 3 M 1
where w is the moisture content in percentage.
-
Specific gravity: The determination of specific gravity was carried out according to [21] test (B) for finegrained soils. The specific gravity is calculated using eqn. (3.2)
-
1000
The dry density was also calculated using the equation:
d 100 /(100 W )
where w is the moisture content of each compacted
layer.
Optimum moisture content
The corresponding values of moisture contents at maximum dry densities (MDD), deduced from the graph of dry density against moisture contents, gives the optimum moisture contents (OMC). The results are given in Tables A3.7a, b, c for each compactive effort.
-
Strength characteristics
Unconfined compressive strength
The unconfined compressive strength (UCS) tests were performed on the soil samples according to BS 1377; 1990 Part 7 using the British Standard Light
GS
m2 m1
(m4 m1) (m3 m1)
—————————-
(BSL), West African Standard (WAS) and British Standard Heavy (BSH) energy levels. The UCS was calculated from
———————– (3.2)
The same procedure was repeated for all the percentages of
the following equation:
Failure load
the admixtures, and the results are shown in Table A3.1
Compressiv e strength
-
Linear shrinkage: The test was conducted in accordance with Test 5 [21]. linear shrinkage was calculated using eqn. (3.3)
Linear Shrinkage (Initial lenght – Dried lenght) 100 %
Initial Length
-
Free swell: The test was conducted in accordance with the United States Bureau of Reclamation (USBR)
method [22].
Free Swell Final Volume – Initial Volume 100 – – –
Inital Volume
Surface Area of Specimen
-
-
Durability Assessment (Resistance to Loss in strength): The durability assessment (under adverse field conditions) of the soil sample was determined by resistance to loss in strength when immersed in water. It was expressed as the ratio of UCS of the specimen cured for 7 days and soaked for another 7 days to the UCS of the specimen cured for 14 days. [7] proposed 80% resistance to loss in strength for the ratio of the UCS of a specimen cured for 7 days and soaked for 4 days to the UCS of the specimen cured for 14 days.
UCS(7 day cured 7 day soaked)
-
Particle size distribution: The particle size analysis test carried out in accordance with BS [21]. Wet sieving was conducted by measuring 200g of the soil sample
Resistance to loss in strength(%)
UCS(14 day cured)
100%
and soaking it for 24hours. The sample was then washed through BS sieve No 200.
-
Atterberg limits: The test includes the determination of the liquid limits, plastic limits and the plasticity index for the natural soil and the stabilized soils. They were also
-
-
DISCUSSION OF RESULTS
-
Properties of Materials Used in the Study
Preliminary tests conducted to determine the natural properties of the soil revealed that the soil has very
Table 2 Oxide composition of LBWA
Oxide
LBWA (%)
CaO
1.08
SiO2
55.38
Al2O3
14.93
Fe2O3
0.278
MnO
0.09
Na2O
0.18
K2O
2.00
SO3
—-
P2O5
0.23
Loss on Ignition
10.63
Table 2 Oxide composition of LBWA
Oxide
LBWA (%)
CaO
1.08
SiO2
55.38
Al2O3
14.93
Fe2O3
0.278
MnO
0.09
Na2O
0.18
K2O
2.00
SO3
—-
P2O5
0.23
Loss on Ignition
10.63
high moisture content of 15%. The index properties are summarized in Table 1, while its oxide compositions are summarized in Table 2. The soil belongs to the CH group in the Unified Soil Classification System [24] soil group of the AASHTO soil classification system [25]. The soil is reddish brown in colour (from wet to dry states) with a liquid limit of 43.9%, plastic limit of 29.0% and plasticity index of 21.9%.
The soil has a free swell of about 55%, soaked CBR values of 5%, 6% and 9% for the three energy levels of British Standard Light, West African Standard and the British Standard Heavy, respectively and UCS values of 251, 364 and 687kN/m2 for the energy levels, respectively. The soil was found to be highly plastic and falls below the standard recommendation for most geotechnical construction works especially for sub-base or base courses in highway construction [26]; [27].
Table 1: Properties of the Natural Soil
Property
Quantity
Percentage passing BS No 200 sieve, %
65.0
Natural Moisture Content, %
15.0
Liquid Limit, %
43.9
Plastic Limit, %
21.0
Plasticity Index, %
22.9
Linear Shrinkage, %
17.0
Free Swell, %
55.0
Specific Gravity
2.34
AASHTO Classification
A-7-6(13)
USCS
CH
NBRRI Classification
High swell potential
Maximum Dry Density, Mg/m3
1.43
British Standard light
1.54
West African Standard
1.63
British Standard heavy
34.0
Optimum Moisture Content, %
28.0
British Standard light
22.0
West African Standard
235
British Standard heavy
493
Unconfined Compressive Strength, kN/m2
974
British Standard light
6
West African Standard
7
British Standard heavy
11
Colour
Reddish Brown
Dominant clay minerals
Kaolinite
Table 1: Properties of the Natural Soil
Property
Quantity
Percentage passing BS No 200 sieve, %
65.0
Natural Moisture Content, %
15.0
Liquid Limit, %
43.9
Plastic Limit, %
21.0
Plasticity Index, %
22.9
Linear Shrinkage, %
17.0
Free Swell, %
55.0
Specific Gravity
2.34
AASHTO Classification
A-7-6(13)
USCS
CH
NBRRI Classification
High swell potential
Maximum Dry Density, Mg/m3
1.43
British Standard light
1.54
West African Standard
1.63
British Standard heavy
34.0
Optimum Moisture Content, %
28.0
British Standard light
22.0
West African Standard
235
British Standard heavy
493
Unconfined Compressive Strength, kN/m2
974
British Standard light
6
West African Standard
7
British Standard heavy
11
Colour
Reddish Brown
Dominant clay minerals
Kaolinite
The little amount of calcium in LBWA (1.08%) to be used for the stabilization process was supplemented by the calcium present in a typical of OPC (of 63%). The higher silicon and aluminium oxides in LBWA also aided by the coarse nature of the quarry dust will provide the required reaction needed for stabilization.
PERCENTAGE PASSING
PERCENTAGE PASSING
Particle size (mm)
Fig. 1: Particle size distribution curve of the natural soil
Plasticity Index (%)
Plasticity Index (%)
-
Atterberg Limits :Plasticity Index: The variation of the plasticity index of soil-cement mixtures with LBWA contents are shown in Fig.2. The reduction in plastic limit and increase in liquid limit were accompanied by a general increase in the plasticity index value from a value of 22.9% to a peak value of 48.6% at 4% QD/6% LBWA treatment. Akinmade (2008) reported the same trend of increasing LL, PI and decreasing PL with increasing admixture contents on stabilizing black cotton soil with LBWA. The same trend was also reported by Stephen (2005) when he researched into the effect of baggase ash on the stabilization of the soil.
0% C
2% C
4% C
6% C
8% C
0% C
2% C
4% C
6% C
8% C
LBWA Contents (%)
Fig.2 Variation of plasticity index of soil-QD mixtures with LBWA contents
-
-
Compaction Characteristics
0%
C 2% C 4% C 6%
C
8%
C
0%
C 2% C 4% C 6%
C
8%
C
-
Maximum dry density: The variation of maximum dry densities (MDD) of soil-cement mixtures with LBWA contents for the three compactive efforts, respectively, are presented in Figs. 3, 4, and 5
Maximum Dry Density
(Mg/m3)
Maximum Dry Density
(Mg/m3)
LBWA Contents (%)
0%
C 2%
C 4%
C 6%
C 8%
C
0%
C 2%
C 4%
C 6%
C 8%
C
Fig.3Variation of maximum dry density of soil-QD mixtures with LBWA contents (BSL Compaction)
Maximum Dry Density
Mg/m3
Maximum Dry Density
Mg/m3
LBWA Contents (%) Fig.4 Variation of maximum dry density of soil-QD mixtures with LBWA contents (WAS Compaction)
[29] also reported the same trend of increasing MDD to peak values before falling at higher admixture contents where further additives had no significant impact on the MDD values. The same trend was also reported by [30]0% C 2% C 4% C 6% C
8%
C
0% C 2% C 4% C 6% C
8%
C
Optimum Moisture Content
(%)
Optimum Moisture Content
(%)
-
Optimum moisture content: The variation of optimum moisture content of soil-cement mixtures with LBWA contents for BSL, WAS and BSH compactive efforts are shown in Figs. 6, 7 and 8 respectively. It is observed that the OMC values when compaction was at the three energy levels increased to peak values at 6% LBWA for all cement contents for all the compactive efforts.
LBWA Content (%)
Fig.6 Variation of optimum moisture contents of soil-QD mixtures with LBWA Contents (BSL Compaction)
D
D
0% C
2% C
4% C
6% C
8% C
0% C
2% C
4% C
6% C
8% C
0% C
2% C
4% C
6% C
8% C
0% C
2% C
4% C
6% C
8% C
Maximum Dry Density
(Mg/m3)
Maximum Dry Density
(Mg/m3)
Optimum Moisture
Content (%)
Optimum Moisture
Content (%)
LBWA Content (%)
Fig.7 Variation of optimim moisture contents of soil-Q
c mixtures with LBWA contents (WAS Compaction)
LBWA Content (%)
Fig.5 Variation of maximum dry density of soil-QD mixtures with LBWA contents (BSH Compaction)
The WAS compaction MDD decreased to a value of 1.5Mg/m3 at 2% QD/8% LBWA, and that may not be unconnected with the presence of large low density aggregate of LBWA particles replacing the soil with a higher specific gravity.
The variation of MDD for the British Standard Heavy (BSH) compaction is also in conformity with the other two energy efforts. Its MDD increased from a minimum 1.63Mg/m3 in the natural state to a maximum 1.72Mg/m3 at 6% QD/6% LBWA. The trend of increasing MDD with admixture contents was reported by [9] and to the same peak value of 6% for OPC before it experienced reduction in the values at higher admixture contents. [28];
0%
C 2% C 4%
C
6%
0%
C 2% C 4%
C
6%
8%
C
8%
C
C
C
Optimum Moisture
content (%)
Optimum Moisture
content (%)
LBWA Contents (%)
Fig.7 Variation of optimum moisture contents of soil-QD mixtures with LBWA contents (BSH Compaction)
The OMC for BSL compaction increased from 34% for the natural state to 40% at 8% QD/6% LBWA. Further addition of LBWA (to 8%) led to a decrease in the OMC to a value of 26% at 0% QD/8% LBWA. In the case of the WAS compaction, the OMC increased from 28% for the natural state to a maximum value of 38% at 8% QD/6% LBWA which later decreased to 26% at 2% QD/8% LBWA. For the BSH compaction, a similar trend was also noticed
whereby the OMC increased from its natural state of 22% to 33% at 6% QD/6% LBWA.
Research works of [9];[29] ; [31]; [27]; [28]; [32] agree with the trend of increasing OMC with admixture contents. An explanation that was offered for these trends is that there was increasing desire for water which commensurates with the higher amount of the additives because more water was required for the dissociation of admixtures with Ca2+ and OH ions to supply more Ca2+ for the cation exchange reaction. Another reason could be due to the increasing surface area caused by the higher amount of the additives, which required more water for the lubrication of the entire matrix.
It was also observed that OMC decreased with higher compactive efforts because it was easier to breakdown flocculated aggregates, destroy shear planes and eliminate large pores, at higher compactive efforts [9]
-
-
Strength Characteristics
Unconfined compressive strength
The general test recommended for use in the determination of the additive to be used in the stabilization of soil is the unconfirmed compressive strength (UCS) test [33]. It is an important factor in the evaluation of the design criteria for the use of soil as a pavement material [7].
The variation of UCS of soil-cement mixtures cured for 7 days with LBWA contents for BSL, WAS and BSH compactive efforts are shown in Fig.9, 10, and 11 respectively. The UCS values for the BSL compaction showed a sharp increase from 47kN/m2 for the natural soil to 586kN/m2 at 6% QD/6% LBWA. Subsequent addition of LBWA content did not increase the UCS, rather, it recorded a slight decrease to a value of 323kN/m2 at 6% QD/8% LBWA because of insufficient water to take the pozzolanic reaction to completion.
0% C
2% C
4% C
6% C
8% C
0% C
2% C
4% C
6% C
8% C
UCS (kN/m2)
UCS (kN/m2)
For specimen compacted with WAS energy, the UCS value increased from an initial value of 390kN/m2 to 1125kN/m2 at 6% QD/6% LBWA. Further treatment of the soil did not produce a positive effect, because the UCS value reduced to a minimum 762kN/m2 at 2% QD/8% LBWA.
LBWA Content (%)
Fig.8 Variation of UCS (7 days curing) of soil-QD mixtures with LBWA contents (BSL Compaction)
LBWA Content (%)
0% C
2% C
4% C
6% C
8% C
0% C
2% C
4% C
6% C
8% C
UCS (kN/m2)
UCS (kN/m2)
Fig8 Variation of UCS (7 days curing) of soil-QD mixtures
0% C
2% C
4% C
6% C
8% C
0% C
2% C
4% C
6% C
8% C
with LBWA ontents (WAS Compaction)
UCS (kN/m2)
UCS (kN/m2)
LBWA Content (%)
Fig.8 Variation of UCS (7 days curing) of soil-QD mixtures with LBWA contents (BSH Compaction)
The UCS for BSH compaction increased from 750kN/m2 for the natural soil to 1240kN/m2 at 6% QD/6% LBWA. Additional treatment with LBWA reduced the UCS value to 1008kN/m2 at 6% LBWA/8% OPC.
The increase in the UCS values (or the gain in strength) was primarily due to the formation of various compounds such as calcium silicate hydrates (CSH) and calcium aluminate hydrates (CAH) and micro fabric changes, which are responsible for strength development. Though, there were increments in the UCS values, they fell short of 1710kN/m2 specified by [35] as criterion for adequate stabilization using OPC. The decrease in the values of the UCS for all the compactive energies beyond 6% LBWA was as a result of insufficient water to bring the pozzolanic reaction to completion [9]. It was stated that larger percentages of cement (8% and beyond) are considered in view of the recommendation of [34], that larger addition of quarry dust is normally considered bad due to the generation of high strength and hence widely spaced cracking by shrinkage or by service stresses or both.
-
Durability Assessment
Durability assessment of soil samples involves the simulation of some of the worst conditions that could be obtained in the field.
Resistance to Loss in Strength
(%)
Resistance to Loss in Strength
(%)
LBWA Content (%)
Fig.9 Variation of Resistance to Loss in Strength of soil-QD mixtures with LBWA contents (BSL Compaction)
Resistance to Loss in Strength
(%)
Resistance to Loss in Strength
(%)
it falls under A-7-6(13) classification for [25] and CH according to Unified Soil Classification System [24]. The natural soil has high moisture content of 15%. It has liquid limit of 44%, plastic limit of 21%, plasticity index of 23%, linear shrinkage of 17%, free swell of 55%, specific gravity of 2.34 and NBRRI Classification of high swell potential. All these values indicate that the soil is highly plastic with about 71% of the soil particles passing the B. S. No 200 sieve. The strength characteristics are also very low, thereby rendering the soil unfit for sub-base or road base courses. The plasticity index values for all the concentration of the additive exceeded the 30% value prescribed for sub-grade materials by the Nigeria General Specification (1997).
0% C
2% C
4% C
6% C
8% C
0% C
2% C
4% C
6% C
8% C
0% C
2% C
4% C
6% C
8% C
0% C
2% C
4% C
6% C
8% C
The MDD increased with higher additive blends and compactive efforts; which is in conformity with the same trend reported by [9]; [30]; [29]; [28]. The peak MDD values recorded for BSL, WAS and BSH compactive efforts are respectively 1.50, 1.68 and 1.72Mg/m3 at 6% OPC/6% LBWA treatment. The OMC, on the other hand, decreased with higher compactive efforts but increased with higher LBWA contents.
LBWA Content (%)
0% C
2% C
4% C
6% C
8% C
0% C
2% C
4% C
6% C
8% C
Resistance to Loss in
Strength (%)
Resistance to Loss in
Strength (%)
Fig.10 Variation of Resistance to Loss in Strength of soil- QD mixtures with LBWA contents (WAS Compaction)
LBWA Content (%)
Fig.11 Variation of Resistance to Loss in Strength of soil-QD mixtures with LBWA contents (BSH Compaction)
In the variation of resistance to loss in strength of soil-QD mixtures with LBWA contents for BSL, WAS and BSH respectively shown in Figs. 12, 13 and 14, the resistance to loss in strength increased from 15, 27 and 35% for the natural soils compacted with BSL, WAS and BSH energies to peak values of 32, 43 and 61% at 6% QD/8% LBWA, 8% QD/0% LBWA and 6% QD/6% LBWA,
respectively. All the peak resistance to loss in strength values fell short of the acceptable 80%.
1V. CONCLUSION AND RECOMMENDATION
-
Conclusion
The preliminary investigation conducted on the lateritic soil collected at Ajase-Ipo, Kwara State shows that
The unconfined compressive strength (UCS) values for natural soil compacted with BSL, WAS and BSH energies at 7 days curing period are 47, 390 and 750kN/m2 respectively and increased to 586, 1125 and 1240kN/m2 at 6% QD/6% LBWA treatment. The values of UCS for both BSL and WAS fell short of the requirement based on Road Note 31 (TRRI, 1977) requirement for economic range of OPC stabilization. However, the UCS value of the BSH compaction could be acceptable for sub- base courses of pavements.
The resistance to loss in strength of the soil increased from 15, 27, and 35% for the natural soil to peak values of 32, 43 and 61% for BSL, WAS and BSH energies at 6% OPC/8% LBWA, 8% OPC/0% LBWA and 6%
OPC/6% LBWA, respectively. Only 61% resistance to loss in strength (39% loss in strength) at 6% OPC/6% LBWA with BSH compaction is close to the limiting value of 80% resistance to loss in strength [7] based on 4 days soaking. The 6% OPC/6% LBWA treatment of the soil can be used, at BSH compaction, for sub-base material because the soil was subjected to a harsher condition (of 7 days soaking) and due to the time dependent gain in strength advantage of the pozzolana.
-
Recommendation
Based on the results obtained, the optimum blend of 6% QD/6% LBWA treatment on the lateritic is to be used as sub-base material when compacted with the British Standard heavy energy.
V. ACKNOWLEDGEMENT
The research was fully funded by the intervention of Tertiary Education Trust Fund, TETFund, of the Federal Government of Nigeria, through the Institution Based Research, IBR, at the federal Polytechniuc, Offa, Kwara State, Nigeria
REFERENCES
[1]. Fredlund, D. G. and Reharjo, H. (1993). Soil Mechanics for Unsaturated Soils, Wiley, New York. [2]. Jones, D. and Holtz, J. (1973). Expansive soils: Hidden Disaster. Civil Engineering, Vol. 43 pp 54. [3]. Yoder [4]. Gilliot, J. E. (1987). Clay in Engineering Geology. Elsevier Publishing Company Amsterdam. [5]. Osinubi, K. J. (1995). Lime modification of black cotton soils. Spectrum Journal, Vol. 2, Nos 1 and 2, pp. 112 122. [6]. Nicholai, J. G. and Lester, A. H. (1999). Traffic and Highway Engineering. 2nd edition Books/Cole Publishing Company, New York, USA. [7]. Locat, Y. Bembe, M. A. and Choquette, M. (1990). Laboratory investigation on the lime stabilization of sensitive clays; shear strength development. Canadian Geotechnical Journal, Vol. 27, pp 294-304. [8]. Rechardjo [9]. Ola, S. A. (1974) Need for estimated cement requirement for stabilizing lateritic soil. J. Transport Div., ASCE, Vol. 17,No 8, pp. 379-388. [10]. Balogun, L. A. (1991). Effect of sand and salt additives on some geotechnical properties of lime stabilized black cotton soil. The Nigeria Engineer, Vol 26, No 2, pp. 15-24. [11]. Osinubi, K. J. (1999). Evaluation of admixture stabilization of Nigerian black cotton soil. Nigerian Society of Engineers Technical Transactions, Vol. 34, No 3, pp 88-96 [12]. Osinubi, K. J. (1998a). Laboratory investigation of engineering use of phosphatic waste. Journal of Engineering Research, University of Lagos, Vol. JER-6, No 2, pp 47-60. [13]. Osinubi, K. J. (1998b). Stabilization of tropical black clay with cement and pulverised coal bottom ash admixture. In: Advances in Unsaturated Geotechnics. Edited by Charles, D., Shackelford, SandraL. Houston and Nien-Yin Cheng. ASCE Geotechnical Special Publication, No 99, pp 289-302.
[14]. Osinubi, K. J. (2000a). Laboratory trial of soil stabilization of Nigerian black cotton soils Nigerian Society of Engineers Technical Transactions, Vol. 35, No 4 pp 13 21. [15]. Osinubi, K. J. (2000b) reatment of laterite with anionic bitumen emulsion and cement: A Comparative Study. Ife Journal of Technology, Obafemi Awolowo University, Ile-Ife, Vol. 9, No 1, pp. 139145. [16]. Cokca, E. (2001), Use of class C fly ashes for stabilization of expansive soils. Journal of Geotechnical Engineering, Vol 127, No 7. pp 568 573. [17]. Medjo, E. and Riskowiski, G. (2004). A Procedure for processing mixtures of soil, cement and sugar cane bagasse. Agricultural Engineering International Journal of Scientific Research and Development. Manuscript BC 990, Vol. III, pp 1-6. [18]. Osinubi and oyelakin 2012 [19]. Oyellakin 2014 [20]. Pavani [21]. B.S. 1377 (1990). Methods of Testing Soil for Civil Engineering Purposes. British Standards Institute, London. [22]. Holtz, W. G. and Gibbs, H. J. (1956). Engineering properties of expansive clays. ASCE Transaction, Vol. 121 No 1, Paper 2814. [23]. B.S. 1924 (1990). Methods of Tests for Stabilized Soils. British Standards Institute, London. [24]. ASTM (1992). Annual Book of Standards Vol. 04.08, American Society for Testing and Materials, Philadelphia. [25]. AASHTO (1986). Standard Specifications for Transport Materials and Methods of Sampling and Testing. 14th Edition, American Association of State Highway and Transport Officials (AASHTO),Washington, D.C
[26]. Butches, F. and Sailie, E. L (1984) Swelling behaviour of tropical black clays. Proceedings of the 8th Regional Conference for Africa on Soil Mechanics and Foundation Engineering, Harare. 81 86. [27]. Osinubi, K. J. and Medubi, A. B. (1997a). Evaluation of cement and phosphatic waste admixture on tropical black clay road foundation. Proceedings of 4th Intnl. Conference on Structural Engineering Analysis and Modeling (SEAM 4), Kumasi, Ghana, 9-11 July, Vol. 2, pp. 297-307. [28]. Akinmade (2008). The Effect of Locust Bean Waste Ash on the Geotechnical Properties of Black Cotton Soil. Unpublished M.Sc. Thesis, Department of Civil Engineering, Ahmadu Bello University, Zaria. [29]. Stephen, A. T. (2006). Stabilization Potential of Bagasse Ash on Black cotton Soil. Unpublished M.Sc. Thesis, Department of Civil Engineering, Ahmadu Bello University, Zaria. [30]. Osinubi, K. J. and Stephen, A. T. (2007). Influnce of compactive efforts on bagasse ash treated black cotton soil. Nigerian Journal of Soil and Environmental Research, Vol. 7, pp. 92 101. [31]. George, M. (2006). Stabilization of Black Cotton Soil with ordinary Portland Cement using Bagasse Ash as Admixture. M.Sc. Thesis,Department of Civil Engineering, Ahmadu Bello University, Zaria
[32]. Ochepo, J. (2008). The Effect of Elapsed Time on the Geotechnical Properties of Black Cotton Soils Stabilized with Lime-Bagasse Ash Admixture. Unpublished M.Sc. Thesis, Department of Civil Engineering, Ahmadu Bello University, Zaria. [33]. Singh, G. (1991): Highway Engineering, 3rd Edition, Standard Publishers Distributors. Pp. 599 619. [34]. Ingles, O. G. and Metcalf, J. B. (1972). Soil stabilization Principles and Practice, Butterworths, Sydney. [35]. TRRL (1977). A guide to the structural design of bitumen surfaced roads in tropical and sub-tropical countries. Transport and Road Research Laboratory, Road Note 31, H. M. S. O., London.