
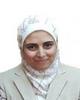
- Open Access
- Authors : Heba Abd El-Aziz , Manal Abdelmoniem , Taher Mohammed Hassan
- Paper ID : IJERTV10IS050035
- Volume & Issue : Volume 10, Issue 05 (May 2021)
- Published (First Online): 07-05-2021
- ISSN (Online) : 2278-0181
- Publisher Name : IJERT
- License:
This work is licensed under a Creative Commons Attribution 4.0 International License
Assessment of the Potential of Groundwater Injection with Cooling Water from The West Assuit Power Plant
Heba Abd El-Aziz, Manal Abdelmoniem, and Taher Mohammed Hassan
Research Institute for Groundwater, National Water Research Centre, Cairo, Egypt
Abstract:- West Assuit power plant, in the desert area west of Assuit governorate, is one of the power generation mega projects in Egypt. The plant was converted from simple to combined cycle with additional capacity of 500 megawatts. Groundwater is the source of raw water for demineralized water production used in the power plant. In this work, numerical simulation modeling using Visual MODFLOW is utilized to assess long term potential of groundwater injection with cooling water from the west Assuit power plant as a mean of disposing treated effluent released by the power plant. Groundwater injection facilities in the power plant comprise three deep injection wells, constructed to recharge the treated effluent into the deep aquifer. Injection tests were conducted to examine recharging capacity of the three deep injection wells. The modeling overall objective in this research is to predict the aquifer response under various injection scenarios and to identify a maximum operational injection rate that would satisfy quantitative and qualitative constraints over 50 years operation horizon. Flow modeling results indicated that the maximum allowable long-term injection rate collectively for the three injection wells should not exceed 1000 m3/d. Result of solute transport modeling for the identified allowable injection rate showed no negative impact on the deep aquifer salinity. This research gives good insight into the existing injection wells. It is essential for any further development of injection facilities and disposing treated effluent released by the power plant.
Keywords: Injection, Groundwater, West Assiut power plant, Numerical modelling.
-
INTRODUCTION
With a rapidly growing population and development of major energy-intensive industries, Egypt requires a large supply of power to maintain growth. Accordingly, the Egyptian Ministry of Electricity and Renewable Energy implemented a mega projects power generation program including West Assuit Power Plant (WAPP) in Assiut governorate in Upper Egypt. The WAPP has been constructed in two stages, the first stage was construction of a simple cycle power plant with a capacity of 1,000 megawatts, and the second stage was converting the plant from simple to combined cycle with additional capacity of 500 megawatts (EEHC, 2017). The plant consists of the main items; power generating facilities, mechanical works, electricity works, 37 groundwater production wells, 3 groundwater injection wells, drainage system, and water treatment and desalination systems (EECH, 2017). Groundwater is used in plant, for the different processes, since it is away from the Nile. Hence, groundwater from production wells drilled in the Quaternary aquifer is the source of water for the WAPP (RIGW, 2015). The overall objective of this work is to assess the long-term potential of groundwater injection as a means of disposing treated effluent released by the power plant.
Several studies discussed artificial recharge and groundwater injection (USGS, 1996). The functions for applying groundwater injection or recharge of groundwater are either using injection wells to fill the gap between groundwater supply and demand or using injection wells for the disposal of effluents in non-exploited deep aquifer. An injection well is used to place fluid underground into porous geologic formations. Protection of aquifer is a major concern when using injection to dispose effluent into groundwater. Therefore, to protect aquifer resources, injected fluids should stay within the well and the intended injection zone and the injected fluids should not adversely affect quantitative and qualitative states of the aquifer system. Accordingly, a groundwater monitoring program should be developed as part of the groundwater injection plan. According to the U.S. Environmental Protection Agency (EPA), the first documented large-scale use of injection wells is the disposal of oil field brine into the originating formation in Texas in the 1930s. Such fluids, injected deep into porous rock formations, consist largely of saltwater, and may contain pollutants. These injection wells have raised concerns about the safety of drinking water supplied from aquifer systems. To protect groundwater drinking sources from contamination, EPA introduced the federal Underground Injection Control Program (UIC). This UIC program regulates constructing, operating, testing, and monitoring for a classification of five types of injection wells (UIC, 1980).
Models can be helpful tools to solve complex groundwater problems and simulate complicated aquifers with irregular geometry, non-linearity, and complex boundary conditions (Craig and Read, 2010). Numerous studies discussed application of numerical simulation models to groundwater injection. Numerical modeling of artificial recharge of the Dammam Formation in Kuwait was carried out using MODFLOW-MT3D and HST3D to assess recharge feasibility. They considered induced rise in head during injection and the volume of recovered water with specified quality (Mukhopadhyay and Al-Otaibi, 2002). Groundwater recharge from injection well as a point source was simulated using an Explicit Finite Difference Model (FDFLOW) and a Galerkin Finite Element Model (FEFLOW), and the modeling results were highly sensitive to injection rate and moderately sensitive to transmissivity (Kulkarni, 2015). Numerical modeling was applied to simulate the flow system of a confined aquifer in Karbala
southwest of Iraq to predict the aquifer recovery behavior using a suggested recharge rate and arrangement of injection wells to replenish groundwater resources and reuse it in dry seasons (Karim and Abd Ali, 2017).
Based on previous studies, the methodology of the study was developed, which is summarized in the use of numerical models in evaluating the effect of injecting liquid wastes resulting from cooling operations in power plants on groundwater.
In this study, assessment of the potential of groundwater injection is based on a combination of field investigations, injection testing, and groundwater flow modeling using Visual MODFLOW. Injection well tests were conducted to examine recharging capacity, being a key criterion in deciding the number of injection wells required to dispose the released effluent. The modeling overall objective in this research is to predict the aquifer response under various injection scenarios and to identify a maximum operational injection rate that would satisfy long-term quantitative and qualitative constraints of induced buildup of groundwater heads and changes in quality.
-
STUDY AREA
-
Site Description
The West Assuit Power Plant (WAPP) is located in the northern west of Assiut city, in Assiut governorate in Upper Egypt. Regionally, the study area is located on a tilted plateau which sloping NE towards the Nile Valley. The topographic level in the study area is nearly homogeneous and has no acute heights, mountains, scarps, or valleys. WAPP lies between the latitudes 30o 59 40 and 31o 00 15 E and between the longitudes 27o 10 35 and 27o 11 10 N, as shown in Figure (1).
Figure (1) Location of the study area
The WAPP consists of a simple cycle power plant with a capacity of 1,000 megawatts, and a combined cycle with additional capacity of 500 megawatts (EEHC, 2017). A combined-cycle power plant uses both a gas and a steam turbine togther to produce up to 50 percent more electricity from the same fuel than a traditional simple-cycle plant. The waste heat from the gas turbine is routed to the nearby steam turbine, which generates extra power. Groundwater is the source of raw water used in the WAPP for steam generation, cooling, sealing, control nitrogen oxides (De-NOx), washing and other purposes.
-
Hydrogeology
The study area is a part of the transition zone between the Nile valley and the western plateau. It is cropping out in geological formations dated between the Quaternary and Tertiary periods, with a relatively complicated geological regime. Structurally the entire zone is part of the old Nile Valley zone. Hydrogeologically, three main aquifers exist in the Assiut area. These aquifers are distinguished from the top into: i) Upper Pleistocene aquifer composed of intercalations of fluvial sands and gravels in the Nile Valley area, and intergranular conglomerates formations in Wadi El Assiuty; ii) Plio-Pleistocene aquifer composed of fine-grained sandstone formations; and iii) Lower Eocene limestone aquifer consisting of fracture carbonate rocks (RIGW, 2017).
According to hydrogeological mapping (RIGW-IWACO, 1992), groundwater in the study area is identified to occur within a low- productive categorization of aquifer systems, taking place in the top Quaternary inter-granular aquifer of graded sands and gravels with clay beds and lenses. The depth from the ground surface to the top of the aquifer in this area ranges between 60 and 190 meters, with increasing thickness northwards as shown in the hydrogeological cross section Figures (2). The aquifer is locally recharged from adjacent aquifers with insignificant surface recharge and depth to the static groundwater level varies from 50 to 62 meters, measured from the ground surface (RIGW, 2017). The groundwater flow direction in the study area adheres to the topography from high to the low elevations, as it flows from the plateau escarpment to the Nile Valley in southwest to northeast direction. Groundwater salinity ranges from 1000 to 5000 ppm, decreasing northwards (RIGW, 2017).
Figure (2) West East Hydrogeological cross-sections in the study area (RIGW, 2017)
-
-
FIELD INVESTIGATIONS
Throughout the period from 2015 to 2019, the Research Institute for Groundwater conducted field investigation to determine the hydrogeological characteristics in the study area, to study the feasibility of abstracting groundwater as a source of water for the different processes in power generation. Field investigations comprised collection and analyses of data from geophysical prospecting, drilling of wells, and pumping and injection testing. In the study area, 37 production wells were constructed. Data collected from these wells on groundwater altitude above mean sea level (amsl) and salinity twice in five years are given in Table (1).
Table (1) Groundwater levels (amsl) and salinity for the production and injection wells
Well Name
Water level (m)
TDS (ppm)
Well Name
Water level (m)
TDS (ppm)
Prod 1
25.62
3036
Prod 20
24.88
1369
Prod 2
26.9
4352
Prod 21
25.72
2464
Prod 3
27.19
3712
Prod 22
25.65
4698
Prod 4
28.01
2732
Prod 23
27.12
2280
Prod 5
28.06
1813
Prod 24
27.97
3840
Prod 6
28.34
2240
Prod 25
27.94
2368
Prod 7
28.07
2112
Prod 26
28.06
2890
Prod 8
30.52
2240
Prod 27
28.17
2368
Prod 9
28.46
2624
Prod 28
28.29
2483
Prod 10
28.82
2643
Prod 29
28.07
2626
Prod 11
29.18
2400
Prod 30
28.53
2400
Prod 12
28.95
2508
Prod 31
28.28
2400
Prod 13
28.13
3328
Prod 32
28.1
3040
Prod 14
28.73
2368
Prod 33
29.17
3776
Prod 15
28.45
2035
Prod 34
29.55
2816
Prod 16
28.54
2432
Prod 35
29.15
2022
Prod 17
27.5
2000
Prod 36
24.43
1785
Prod 18
25.83
2112
Prod 37
25.02
1800
Prod 19
25.07
1286
Three injection test wells were constructed to test the potential of disposing treated effluent released by the power plant into the deep aquifer. Location of the injection wells is shown in Figure (4). Main characteristics of the injection test wells are given in Table (2). After completion of well construction and development, step and continuous pumping tests were performed on each well. The pumping test data were analyzed, and the results indicate that the aquifer transmissivity ranges from 25 and 250 m2/d, while the average value of storativity amounts to 1.564×10-5 (RIGW, 2017). Injection tests were then conducted to examine recharging capacity of the three deep injection wells, as well as, the aquifer response under different injection rates. The source for the injection tests was water from the groundwater pumped in the study area. Both step-rate, and continuous injection and
falloff tests were performed in the injection wells using gravity-fed recharge and pressure-forced recharge. During each injection test, the water levels in the injection well and in two nearby observation wells were measured continuously. Based on the results of the continuous injection tests using gravity-fed recharge, it is concluded that the three wells collectively have a total capacity to inject about 1500 m3/d. On the other hand, results of the continuous injection tests using pressure-forced recharge suggest that the three wells collectively could have a total injection capacity of about 2400 m3/d (RIGW, 2017).
Table (2) Main characteristics of the deep injection wells (RIGW, 2017)
Well Name
Well Coordinates
Well Depth (m)
Screen Length (m)
Depth to Groundwater (m)
TDS
(ppm)
East
North
Inj W1
30o59 3.5
27o 11 08.7
300
119
72.04
5280
Inj W2
31 00 11.1
27 10 57.5
310
125
58.55
2405
Inj W3
31 00 07.1
27 10 47.1
310
101
59.85
2391
-
NUMERICAL MODELING
Numerical groundwater modeling is a powerful and helpful tool for planning and decision-making involved in groundwater sustainable development, and aquifer restoration. The objective of this modeling work is to simulate groundwater flow in the WAPP study area to predict the long-term aquifer response to groundwater injection as a means of disposing treated effluent released by the power plant.
Numerical solution is a mathematical way to approximately solve the nonlinear partial differential equations that expresses the physical processes described by the model. The partial differential equations are therefore converted into a system of algebraic equations that are subsequently solved through numerical methods to provide approximate solutions to the governing equations. Numerical modeling is at present widely used to simulate groundwater flow problems. Combining Darcys law with the continuity equation we obtain the Partial differential equation, known as Laplace Equation. One way to solve this equation is the finite differences technique. In this study, numerical groundwater flow simulation is applied using VISUAL MODFLOW for flow simulation (Harbaugh et al., 2000). MODFLOW solves three-dimensional groundwater flow and solute transport equations and also can be used to solve problems of steady and unsteady states for groundwater. The governing equations for groundwater flow in transient conditions for an anisotropic saturated porous media is
Where: Kx, Ky, Kz are values of hydraulic conductivity along the x, y and z coordinate axes [L/T]; h: is the potentiometric head [L]; Ss: is the specific storage of the porous material [L-1]; and t: is time [T].
2
2
2
= + +
2 2 2
Where: Dx, Dy and Dz are hydrodynamic dispersion coefficients in the x, y and z directions [L2/T]; : is is the advective transport or seepage velocity in the x direction [L/T]; : is an effective first order decay rate due to combined biotic and abiotic processes [1/T]; and R: is the linear, equilibrium retardation factor.
MODFLOW simulates steady and non-steady flow in an irregularly shaped flow system in which aquifer layers can be confined, unconfined, or a combination of confined and unconfined. Flow from external stresses, such as flow to wells, areal recharge, evapotranspiration, flow to drains, and flow through riverbeds, can be simulated. The simulation package is Visual MODFLOW Program 4.2 package, which is a multi-dimensional, finite difference, block-centered, saturated groundwater flow code. MT3D numerical solute transport model is used to map the impact of injected effluent on groundwater quality (TDS), compared to the original groundwater salinity in the WAPP study area.
The model is run under three injection scenarios over 50 years prediction horizon to identify maximum operational long-term injection rate that on induced buildup of groundwater heads and changes in salinity. The solute transport package is then used to simulate groundwater and predict the corresponding change in concentration.
-
Conceptual Model
The Hydrogeological Conceptual model for West Assiut Study area represents the aquifer by seven main layers in the vertical direction to better simulate the different lithological structures of the aquifer system, as illustrated in Figure (3). The first layer
represents a shallow aquifer which consists of graded sand with thickness varies from 200 to 250 m (extraction zone according to production wells results), the second layer represents a clay layer with thickness of about 50 m, the third layer represents an aquifer consisting of graded sand attains a thickness of about 100 m (injection zone according to constructed injection wells), the fourth layer represents clay layer with thickness about 50 m, the fifth layer represents confined aquifer consists of shale and clay with 400 m, the sixth layer represents clay layer with thickness about 50 m and the seventh layer represents a confined sandstone aquifer with a thickness of about 400m.
Figure (3) Hydrogeological Conceptual Model of the Study Area
-
Modeling Domain and Inputs
The flow model domain of the WAPP study covers an area of 25 km2. The model grid consists of 100 columns and 100 rows, with a uniform grid size of 50 m by 50 m as shown in Figure (4).
Figure (4) Model Grid and the location of the Injection Wells in the Power Plant
Correct definition of boundary conditions is a critical step in modeling groundwater flow systems. Boundary conditions are mathematical statements specifying the head or fluxes of the problem domain. When the interest area is small that the model boundaries are far away from natural hydrogeological boundaries, as the case of our model area, artificial distant boundary conditions need to be specified according to long-term observation of groundwater at those boundaries. Accordingly, the northern and north east boundaries are simulated as a constant head boundary (equal to 32 m amsl), the southern and south west boundaries are simulated as a constant head boundary (equal to 10 m amsl), and the south-eastern and north-western boundaries are simulated as a "no flow boundary".
The hydraulic parameters assigned to the model are those determined from conducted field investigations in this study and reported values from previous studies. Spatial distribution of hydraulic conductivity in the power plant area is shown in Figure (5). Measured groundwater levels and flow directions in the power plant study area for the year 2019 are shown in the contour map Figure (6). The general groundwater flow direction is north east to south west.
The solute transport package (MT3D) is used to predict the change in groundwater concentration. The concentration of salts in the study area before injection was used as initial concentrations in the solute transport model.
Figure (5) Model Assigned Hydraulic Conductivity (m/day)
Figure (6) Groundwater levels (in meters) and flow directions, 2019
-
Flow Model and Solute Transport Calibration
Calibration aims to obtain an optimal fit between the calculated and the measured data The calibration process is conducted by changing the model parameters to achieve such fit between field measurements and calculated data with acceptable error criterion. Hydraulic conductivity is used as a calibration parameter. The value was changed in the area of the wells and around the station. The model is calibrated under steady-state condition. The model is calibrated according to the year 2019 water levels for the production wells in layer1, injection wells in layer three and deep wells in layer 5 and layer 7. The residual mean square error is found to fall within ± 20 cm as shown in Figure (7).
The MT3D code was calibrated using the existing salinity field data of the year 2019. Longitudinal dispersivity and horizontal hydraulic conductivity are used as a calibration parameter Figure (8) shows the calibration curve for salinity values at year 2019.
Figure (7) Flow Model Calibration
Figure (8) Solute Transport Model Calibration
-
Simulated Injection Scenarios
The calibrated model is run under three scenarios of different injection rate over 50 years prediction horizon. The simulated scenarios, in terms of the total applied injection rate in the three injection wells, varied from 500 m3/d for the first scenario, 1000 m3/d for the second, and 1500 m3/d for the third scenario. The objective of this scenario analysis is to identify maximum long- term operational injection rate that would satisfy stipulated requirements for the protection of the power plant sub-structures against induced buildup of groundwater heads and changes in salinity. The decision criterion used to discriminate among the results of the three scenarios is that thedepth to groundwater after 50 years at any of the injection wells should not be less than 25 m, measured from the ground surface.
Buildup in groundwater heads after 50 years of simulated injection for the first, second and third scenarios are shown in Figure 9 (a), (b) and (c), respectively. The model results for the simulated scenarios, expressed in terms of groundwater rise and depth to groundwater from ground surface at each injection well after 50 years of injection, are given in Table (3). According to these results, it is concluded that the second scenario with injection rate of 1000 m3/d is the recommended maximum long-term operational injection rate that would satisfy the decision criterion to protect the sub-structures at the power plant from excessive groundwater rise.
-
First Scenario
-
Second Scenario
-
Third Scenario
-
Figure (9 a, b, and c) Groundwater Rise after 50 Years
Table (3) Model results at each injection well for the simulated scenarios
Injection Well No.
Variables
Scenario 1
Scenario 2
Scenario 3
Inj W1
Depth to groundwater before injection (m)
72.04
Groundwater rise after 50 years (m)
17.21
35.50
49.80
Depth to groundwater after 50 years (m)
54.38
36.54
22.24
Inj W2
Depth to groundwater before injection (m)
58.55
Groundwater rise after 50 years (m)
6.80
14.67
16.20
Depth to groundwater after 50 years (m)
51.75
43.88
42.35
Inj W3
Depth to groundwater before injection (m)
59.85
Groundwater rise after 50 years (m)
15.50
31.23
32.80
Depth to groundwater after 50 years (m)
44.35
28.62
27.05
The average value of salts concentration in the first layer is about 2600 mg/l and the average value in the third Layer is 3600 mg/l. The regional solute transport model was linked with the calibrated flow model. Therefore, changes in groundwater water quality at any location in the study area are attributed to changes in recharge and discharge rates. The model was run to assess the impact of the recommended injection scenario on the initial concentrations. Salinity prediction after 50 years of simulated injection for the second scenario is shown in Figure (10). Hence, the model result showed that the recommended injection scenario has no negative impact on the deep aquifer salinity.
Figure (10) Predicted Salinity (in ppm) for the Second Injection Scenario
-
-
CONCLUSIONS
Groundwater is the source of raw water for demineralized water production used in the West Assuit Power Plant. In this study, the potential of groundwater injection with cooling water from the power plant as a means of disposing treated effluent released by the power plant was assessed. Groundwater injection facilities in the power plant comprise three deep injection wells, constructed to recharge the treated effluent into the deep aquifer.
The simulated scenarios were set-up in terms of total applied injection rate in the three injection wells, which varied from 500 m3/d for the first scenario, 1000 m3/d for the second, and 1500 m3/d for the third scenario.
Scenario analysis was performed with the objective of identifying maximum long-term operational injection rate that would satisfy requirements for the protection of the power plant sub-structures against excessive groundwater rise and changes in salinity. The decision criterion used to discriminate among the simulated scenarios is that the depth to groundwater, measured from the ground surface, after 50 years of injection should not be less than 25 m, at any of the injection wells. Accordingly, it is concluded that the recommended maximum long-term operational injection rate for the existing three injection wells is the second scenario, with injection rate of 1000 m3/d.
Obtained results of the solute transport modelling confirmed that the recommended injection scenario has no negative impact on the deep aquifer salinity.
This research gives good insight into the existing injection wells and is essential for any further development of injection facilities and for long-term operation and management procedures for disposing treated effluent released by the power plant. Implementation of continuous monitoring programs for heads and quality of groundwater, and the quality of the injected effluent are highly recommended to conserve the aquifer system, to protect the sub-structures at the power plant, and to maintain efficient injectivity of the injection facilities.
ACKNOWLEDGEMENT
We would like to thank the staff of the Research Institute for Groundwater, Ministry of Water Resources and Irrigation for their help in collecting the data and field investigation used in this research. We especially thank Prof. Ahmed Khater for his careful follow-up and review of the research
REFERENCES
-
EECH, 2017. Arab Republic of Egypt, Ministry of Electricity and Renewable Energy, Egyptian Electricity Holding Company (EEHC) annual report, 2017.
-
Craig, J. R., and W. W. Read, 2010. The future of analytical solution methods for groundwater flow and transport simulation, in XVIII International Conference on Computational Methods in Water Resources, pp.18, International Center for Numerical Methods in Engineering.
-
Harbaugh A. W., Banta E. R., Hill M. C. and McDonald M. G., 2000. MODFLOW-2000, U.S. Geological Survey modular groundwater model: User guide to modularization concepts and the groundwater flow processes; USGS Open File Report 00-92, Washington DC, USA.
-
Karim I. R. and Ali A. M., 2017. " Artificial Recharge of Groundwater by Injection Wells (Case Study)", International Journal of Scientific Engineering and Technology Research, Volume 06, IssueNo.31, October-2017, Pages: 6193-6196
-
Kulkarni, N.H, 2015. Numerical simulation of groundwater recharge from an injection well. International Journal of Water Resources and Environmental Engineering 7(5):75-83.
-
Mukhopadhyay A, Al-Otaibi M (2002) Numerical simulation of fresh-water storage in the Dammam Formation, Kuwait. Arab J Sci Eng27(2B):127 150
-
RIGW-IWACO, 1992. Hydrogeological Map of Assiut- Egypt, 1:100,000, Cairo, Egypt.
-
RIGW, 2015. "Hydrogeological study and Groundwater Modeling results of West Assiut Power Generation Plant " Technical report of drainage wells.
-
RIGW, 2017. "Technical Feasibility study for proposed Brine Water Drainage System at Assuit Power Generation Plant " Technical report of drainage wells.
-
UIC, 1980. Underground Injection Control Program, Statement of Basics and Purpose, U.S. Environmental Protection Agency, Office of Drinking Water, National UIC Program Docket Control Number D 01079.
-
USGS, 1996. Artificial Recharge of Groundwater by Well Injection for Storage and Recovery, Cape May County, New Jersey, U.S. Geological Survey.