
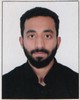
- Open Access
- Authors : Nikhil Shinde , Zaid Salema , Burhanuddin Sakarwala
- Paper ID : IJERTV9IS120040
- Volume & Issue : Volume 09, Issue 12 (December 2020)
- Published (First Online): 11-12-2020
- ISSN (Online) : 2278-0181
- Publisher Name : IJERT
- License:
This work is licensed under a Creative Commons Attribution 4.0 International License
An Investigation of Non-Return Valves as Possible Sources of Pump Failure and A Comparative Analysis with Tesla Valves
Nikhil Shinde Computational Sciences in Engineering Technical University of Braunschweig,
Braunschweig, Germany
Zaid Salema Mechanical Engineering University of Mumbai, Mumbai, India
Burhanuddin Sakarwala Mechanical Engineering University of Mumbai, Mumbai, India
Abstract In any industrial application where the continuous movement of fluids is concerned, Non-Return Valves (NRVs) are treated as essential components to prevent any backflow of the fluid under observation. NRVs conventionally contain mechanically actuated moving parts which operate when the pressure at the suction side exceeds that of the discharge side. The current market is dominated by mechanically actuated NRVs, and those with no moving parts are not used extensively, despite the availability of information and resources that may yield desirable alternatives. Tesla valves are the most commonly documented NRVs that lack moving parts, and hence their comparison with traditional pieces of equipment is imminent. In this paper, we lay bare the differences between both categories of NRVs in their ability to prevent the backflow of fluids and note the reasons why mechanically actuated NRVs may cause pump failure, with the sole aim of decreasing the number of moving parts, wear and tear and friction. In the end, they are given a review.
Keywords Tesla valve; NRV (Non-Return Valve); Check valve; Diodicity; Losses; Vortex; Siphon; Pumps; Computational Fluid Dynamics (CFD); Heat and Mass Transfer; Net Positive Suction Head; Reynolds Number; Laminar Flow; Turbulent Flow.
-
INTRODUCTION
The boilerplate term for a valve which has no moving parts is a Tesla Valve, the valve was planned as a piece of Tesla's new steam engine with the expectation of expanding power plant productivity. Nonetheless, not exactly a month after Tesla documented the patent, he filed for bankruptcy. This began the end of a considerable lot of the aggressive technological breakthroughs for which he had worked intensively all his life. Because of his ignominy after the creation of the light bulb and the resulting expiry of the patent for his valve, the 'valvular conduit' planned by him turned out to be all the more broadly acknowledged as no one who put together their plans concerning the first one ever needed to pay any sovereignties. Tesla named it as a 'valvular conduit' whose insides were given augmentations, breaks, projections, astounds, or containers which, while offering essentially no protection from the section of the liquid one way, other than surface friction as well as
resistance, comprise a practically obstructed boundary to its stream the other way.
Figure 1. Two consecutive segments of a Tesla valve
-
The Geometry of a Tesla Valve
It could likewise be named as a fixed geometry passive check valve, because of the inner edges and curves incorporated into it. The valve is intended to forestall the reversal of any working liquid that flows through it and the interior curves and edges inside the valve. Any liquid that endeavours to flow in reverse into the valve is parted into secondary and tertiary streams that go through the twists in the valve to slam into and confine the progression of the primary stream, to where the primary stream is decreased to a couple of beads overflowing from the opening at the opposite finish of the valve. The inventor himself claimed that pressure ratios up to 200 could be generated with enough consecutive sections in the valve. The Tesla valve finds broad use in thermodynamics, micro fluids, and siphoning applications. Gamboa et al [1] depicted the Tesla valve as offering advantages, for example, strength, versatility and simplicity of creation in an assortment of materials over its peers. Tesla outlines this with drawings, demonstrating one potential development with a progression of eleven stream control portions, albeit an n-number of such fragments could be utilized as required, to increment or abate the flow regulation effect.
-
The internal structure of a Tesla Valve
A Tesla valve is a valvular conduit and a fluid diode that might be utilized in an assortment of miniature channel applications for passive flow correction and control. There aren't nearly enough investigations of microvalves with no-moving parts. There exists a limited amount of literature for research purposes. The valve's geometry in microchannel applications has not been accounted for. This absence of data has impeded the utilization of NMPV (no moving parts valve).
-
Methods of ascertaining a Tesla valves adequacy
The valve's adequacy is evaluated by the Diodicity, which is primarily governed by the incoming flow speed, its design and direction-dependent minor losses throughout its structure during forward and reverse flows. It has been recently demonstrated that the Reynolds number at the valvular delta does not effectively predict the behaviour of the whole stream system all through the Tesla valve. Hence, pure- laminar estimation techniques are not dependable. Local flow instabilities reveal both transitional and turbulent characteristics. The Reynolds-averaged NavierStokes equations (or RANS equations) based flow simulation approach predicts Tesla valve diodicity via three-dimensional (3D) computational fluid dynamics (CFD) for inflow Reynolds numbers up to Re = 2,000.
-
Mechanically Actuated NRVs
Conflictingly, conventional Non-Return Valves are constantly added at the exit ends of siphoning (pumping) applications and
are often liable for the failure of pumps. The working of the valve is as per the following, a spring with a plug machined to fine graded tolerances against the interior breadth of the casing, worked to counter the NPSH (Net Positive Suction Head) of a liquid provided over a line, and once the NPSH needed to actuate or push the spring is sufficient, it is lifted by the lower part of a cylinder that opens the valve. NRVs are complex fluid control systems and they are dependent upon the impact of elements rather than attractions and release heads. NRVs are reasonably complicated fluid control mechanisms and they are subject to the influence of factors other than suction and discharge heads. Their internal mechanisms, once installed, are beyond visual observation and thus their problems are not easily detected. The colossal improvements in computational evaluation techniques, mathematical strategies and computing innovations have made Computational Fluid Dynamic one of the most essential tools for hydrodynamic design of valves, pumps and other fluid- related machinery. A valve designer can assess his plan mathematically using CFD well in advance of experimental model tests and obtain a significant amount of information on 3-D flow fields, which is potentially very valuable to improve the design.
-
-
LITERATURE SURVEY
A systematic literature survey was conducted in the domain of tesla valves, NRVs, hydrodynamic design systems based on CFD and losses in pipes over the range of the last fifty years (1970 onwards), and more than 30 articles were referred from peer-reviewed international journals and publishers (Emerald, Springer, ASME, IEEE, Taylor and Francis, Elsevier and others). The detailed literature survey has been carried out in the coming sections.
Types of non-return valve
Types of non-return valve
Mechanical nrv (moving parts)
Non mechanical NRV
(no moving parts)
Tesla valve
Mechanial nrv (moving parts)
Non mechanical NRV
(no moving parts)
Tesla valve
Swing check valve
Ball check valve Failures or malfunction of
check valve
Stop check valve
Swing check valve
Ball check valve Failures or malfunction of
check valve
Stop check valve
Vertical or in-line check valve
Vertical or in-line check valve
Wear and tear
Foreign materials
Wear and tear
Foreign materials
Effects of failures in check
Effects of failures in check
Corrosion
Flange or failure of flange bolt
Corrosion
Flange or failure of flange bolt
Contamination of
other streams
Bogging of pipework Low pressure sequence trip
Lubrication
Contamination of
other streams
Bogging of pipework Low pressure sequence trip
Lubrication
Preventive actions Flushing Cleaning
Maintenance or rapair of valve
Preventive actions Flushing Cleaning
Maintenance or rapair of valve
Figure 2. A flowchart describing NRVs and their common modes of failure
-
Mechanically Actuated Non-Return Valves
-
Swing Check Valve:
In swing check valves a disc swings across a fixed guide pin that opens or closes according to the fluid flow pressure across the pipes. Many alterations are available in the design of the swing valve for the various applications. The valve disc rests on the seat which is fitted at the closing position of the swing check valve. There are certain variations in the seat angles; larger seat angles cut back the disc travel, leading to quick closing of the valve and thereby reducing the noise and vibration in the system. Sometimes valves are fitted at inclinations of about 5-7 degrees.
-
Ball Check Valve:
A Ball check valve consists of a ball inside the valve which rests on the seat. The ball has a slightly larger diameter than the hole (seat). When the pressure behind the seat exceeds that above the valve, then the liquid enters through the hole, but when the pressure above the valves dominates the pressure before the seat it returns to rest and creates a blockage in the fluid flow and seals the hole properly to avoid any backflow of the fluid.
-
Stop Check Valve:
A stop check valve can be used either as a one-way valve or isolation(stop)valve, similar to that of a gate valve. This valve is used as a traditional check valve in pipes. When required, it is often used as a complete blockage of the fluid flow. This valve is closed with the assistance of the screw-down stem that isnt mounted to the disc valve. the stem, once totally screwed down, holds the free-floating disc against the valve seat as like in gate valves. These valves are available in a different configuration like tee(T) pattern, Wye-pattern and inclined pattern. The swing and piston lift disc-style check valves are normally used as stop check valves.
-
Vertical or in-line Check Valve:
In-line check valves consist of a spring and disc which is rigidly attached to the outflow casing and it can be utilized in each vertical and horizontal pipeline. In-line vertical check valve and guided disc inline check valves should be incorporated with a spring-assisted closure that is employed in the horizontal pipelines. In vertical lines, the guided disc is sometimes supplied with spring-assisted closure. The purpose of the spring-assisted system is not solely for closing the valve quickly but to reduce the effects of the loud noise by preventing flow reversal of the fluid.
Causes of Valve Malfunctions:
-
Wear and tear
-
Foreign materials
-
Corrosion
-
Flange or failure of the flange bolts
Effects of failure of Non-Return Valves:
-
Contamination of other streams or fluids particles
-
Contamination from the external sediments from the atmosphere
-
Low-pressure sequence trips
-
Bogging of pipework
Actions to be taken if any malfunctions occur:
-
Flushing of fluid
-
Cleaning of pipes
-
Maintenance or replacing of the valve
-
The following points should always be considered when selecting NRV:
-
Flow media:
The valve must have a fully open and clear bore design to allow solids to pass.
-
Flow rates:
It allows the correct valve size to be calculated. Different check valve types reach the fully open position at different flow velocities. To optimize system efficiency, the valve must be in the fully open position under normal flow conditions as this will minimize the head loss across the valve. It also ensures that wear and tear against the internal moving parts is minimized and that it will operate under stable conditions.
B. Vortex Diodes
B. Vortex Diodes
Figure 3. The composition of the most common forms of failure in traditional NRVs
A working NRVs are utilized to shield pressure pipelines from hydraulic impingements, they can be applied as flow control and correctional components of high operational dependability as fluid hardware in pump manufacturing and microfluidics. The nature of NRVs is controlled by diodicity the proportion of diode water-powered protection from the stream in forward and turn around bearings. Vortex diodes are the most effective ones as far as diodicity is concerned. It is usually realized that diodicity from obstruction relies upon the geometrical construction of the NRV stream part and the fluid stream design. The intention was to confirm the dependence of hydraulic resistance coefficient of the Reynolds number. To accomplish this, a vortex NRV was constructed and tentatively experimented with. Air was utilized as the working medium. Test outcomes demonstrated that for the Reynolds quantities of under 500, the hydraulic resistance coefficient had practically linear dependence on the Reynolds number and decreased strongly with the Reynolds number increment. In the scope of Reynolds numbers from 500 to 1300, the irregular progress from laminar to turbulent flow systems and the scattering of
experimental points were observed. In the section of flow initial turbulent conditions, within the range of Re from 1300 up to 3000, hydraulic resistance coefficient was balanced across the line and with Reynolds numbers, more than 3000, the area of resistance self-similarity was observed. Thus, the application of vortex diodes seemed appropriate only for Reynolds numbers over 3000 (RM) Kharabova et al [2] A void combining SI and CGS units, such as current in amperes and magnetic field in oersteds often leads to confusion because equations do not balance dimensionally. If you must use mixed units, clearly state the units for each quantity that you use in an equation.
-
Computational Fluid Dynamics Flow Simulation Vortex Diodes
Vortex diodes are utilized as slightly leaking NRVs in applications, where it behooves us to reject valves with moving parts. No itemized investigation of the flow inside the vortex diodes is available, despite their use in practice for several decades. A methodology was inferred for the CFD re- enactments of the vortical flow in NRVs. An adequate arrangement was seen between pressure drop over the channel source ports from CFD recreations. The simulations showed that in the backflow situations, tangential velocity dominated the streams and resulted in maintenance of angular momentum in the valve until it reached the axial exit port. This vortical motion-induced a substantial pressure drop (DPr). The axial velocity gradient over the valve cross-section helped in inducing re-laminarization of the flow. In the forward flow mode, the fluid was distributed radially over the valve and exited through the tangential port, yielding low DPf. The analysis showed that the performance of a NRV is strongly affected by diode geometry, aspect ratio, construction, Reynolds number ad nozzle arrangement. Among different arrangements, the nozzles with entry port size equal to NRV yielded higher diodicity. In the research conducted by Kulkarni et al [3] the comparison with smaller angles, and at higher flow rates a marginally higher diodicity was obtained using axial nozzles with a larger radius of curvature for the valve section.
iH
Rei =
-
Static Non-Return Valves/Tesla valves
A Tesla valve is a valve which has no moving parts (NMP). In the development of a Tesla valve, there are liquid channels with directionally dynamic stream resistances. The liquid channels control the progression of fluid flow in a single direction. There are predominantly two channels namely primary and secondary channels. The primary stream passes unimpeded initially in the straight line and the secondary streams along the redirected direction.it is unidirectional because primary stream flows along the straight passage and secondary stream over the redirected passage. As the liquid passes from the secondary redirected channel, it loses its force and causes a pressure drop into the primary and the secondary stream causes vortices and heavily intense turbulence in the primary stream. Due to the vortices generated the incoming fluid stream from primary path flows from the divergent part, and hence it acts as a non-return valve. The correct valve sizes must be selected so that the framework will work easily with
upgraded productivity, have substantially decreased maintenance, and have an increased tool life.
Figure 4. The cross section of a single Tesla valve
At first, intended for vaporous flow control, a tesla valve is a passive gadget requiring no external interference for activity. As shown, the valve acts as a fluidic diode taking into consideration special forward and backward flow heads because of its special characteristics which create directionally specific pressure drops. There is an assortment of other static, no-moving-parts valves (NMPVs) for fluidic correction and stream control, including the nozzle/diffuser valve, terminal check valve, bridge check valve, and ball-shaped check valve. In contrast to these different kinds of valves, the Tesla valve can achieve detached stream control by bifurcation and jet impingement instruments. a run-of-the-mill Tesla valve comprises two parallel conduits, which incorporate the helix (the bifurcating corridor or bent segment), and the storage compartment (the beginning conduit or straight-channel divide). Tesla valves are adaptable in size and promptly manufacturable and, dissimilar to micropumps with moving parts, can be adequately utilized with colloidal suspensions.
-
Losses in Pipes
Ntengwe et al [4] demonstrated that fluid flow in pipes is constantly impacted by the resistance to flow offered by the roughness of pipe at the walls established by the law of similarity. Smooth pipes offer negligible resistance to flow while rough surfaces offer incrementing resistance depending on the degree of roughness. Such resistance affects velocity distribution and flow rate (Q) of process fluid in the pipe. Every organization requires that the transportation of process fluids be proficient, i.e., that the transporting system must not provide undue hindrance to the flow of fluid in the pipe. Any fittings, valves and bends placed in the fluid-transport system should also offer little resistance to flow of material in order to maintain high efficiency in conveyance and minimalize losses due to friction whether the fluid is a single, double or triple phase system.
Majorly, the transference of fluids is reliant on the quantity of fluid and capacity of the pump and pipe line, properties of the fluids to be transported are imperative as these determine whether the fluid will flow with little or high resistance. The choice of any pipe for a particular process fluid is based on the correlation between the flow rate and cross-sectional area of pipe as given. Valves and bends present a challenge, as stated
earlier, in the transportation of fluids in pipe-lines of the process industry. The D of the pipe can then be determined for a particular maximum discharge.
2
CAD modelling and an inverse design model. The design system is directly associated with a rapid prototyping production system and a flexible manufacturing system composed of a group of DNCs (Direct numerical control)
machines. The use of this novel design system leads to a
=
=
4
0.5
radical decline of development time of pumps having high performance, high reliability, and innovative design concepts.
= { }
-
Hydrodynamic Design System for Pumps Based on 3-D CAD, CFD, and Inverse Design Method
A computer-aided design system has been developed for hydraulic parts like tesla valves, NRVs and geometries involving impellers, volutes, blow diffusers and vaned return channels. The crucial prerequisite technologies include, automatic grid generation, CFD analysis, three-dimensional
The system structure and the design process of Valve Design System and Network Design System are presented. The reviewed geometries include a tesla valve, mechanically actuated NRV, centrifugal impeller with suppressed secondary flow, a bowl diffuser with suppressed corner separation, a vaned return channel of a multistage pump, and a centrifugal pumps volute casing. The results of experimental validation, including flow fields measurements, have been presented in the surveyed paper by Akira et al [5] and discussed briefly.
-
-
METHODOLOGY
CFD
AREA OF RESEARCH
Oil and Gas applicaions
Oil and Gas applicaions
Hydrodynamic Fluid flow
Heat and Mass transfer
Discussion with experts Literature Survey
Identification of problems
e.g.: Water hammer effect, Internal wear and tear, corrosion
Identifying search engine
Seeking assessment
from a pool of experts
Selection
of experts
Administer Questionnaires
Proposal of Ideas
and viewpoints Brainstorming
Obtain various opinions and feedbacks
Consolidate feedback
Summarize results
Seeking assessment
from a pool of experts
Selection
of experts
Administer Questionnaires
Proposal of Ideas
and viewpoints Brainstorming
Obtain various opinions and feedbacks
Consolidate feedback
Summarize results
Discussion with experts Literature Survey
Identification of failures in NRV
Taylor & Francis
Science Direct
Springer
Google Scholar
Contamination
Bogging of pipework Flow rate
CFD simulation of flow in vortex diodes Classifictaion of NRV's
Vortex diodes
Selection of search engine Identify keywords
Searching in selected search engine based on
keywords
Discussion with experts
Literature Survey
Download relevant articles form reputed journals
Hydrodynamic Design System for Pumps Based
on 3-D CAD, CFD, and Inverse Design Method
Scrutinize based on keywords
Reading and Identifying objectives,
methodology, findings, conclusion and future
Discussion with experts
Literature Survey
direction
Methods for failure detection in mechanically actuated NRVs
Identify Literature Gap
Implementation of an algorithm that would aid a soft sensor arrangement in the detection of NRV failure
Valve condition monitoring
Visual inspection
Pressure losses for fluid flow in curved pipes
Experimental Methods to determine NPSH
Discussion with experts Literature Survey
Parameters for 3D fluid flow through a Tesla valve
Results and discussions
Applications
Applications
Conclusion
Conclusion
Figure 5 Functional flow block diagram of research methodology
-
Methods of detecting the failure in traditional/mechanicallyactuated NRVs
The problem brought by valves with moving parts is that they dont last long, they are heavy and expensive. What is wanted from a valve is to be inexpensive, easy to manufacture, with a high life expectancy and reliability. Valves without moving parts are being preferred in gas turbines.
The function of an NRV is to prevent complete back flow of any fluid medium it is responsible for handling. Multiple failures of pumps were detected consecutively, i.e. one after the other. After a series of failed investigations, where the integrity of the pump and motor shaft and other externalities were studied extensively, the measurements of vibrations during pump change overs brought the real issues to light.
Keri et al [6], in a study titled, A Non-Return Valve: A Possible Source for Pump Failure, in which their research identified the failure of mechanically actuated NRVs. A power plant and its centrifugal lift pumps functioned appropriately for six months after the NRVs were commissioned to be installed. After the first six months, regular and incessant failures were observed, eventually leading the researchers to study the possible sources of failure. At first, it was assumed that the misalignment between the pump and its motor shafts and their connecting rods was the reason for regular shutdowns, and after vibrational analysis of the frequencies of the acoustical waves through the apparatus, it was concluded that the values they observed were normal and hence decided to abandon that specific interpretation.
However, the vibrational analysis during the pump change over phase, during which the NRV must begin operating, resulted in some interesting conclusions. Large impacts had been detected, far exceeding the normal acceptable range for a pump-motor combination. The averages of several readings from accelerometers installed at the valve, inlet and outlet pipes revealed that the lack of discharge from the pump, and when water in the outlet pipe, with exceedingly high total energy head, returns and applies an impulsive load to the non- return valve. This phenomenon is what accrues the satirical term water hammer to a Non-Return Valve. The high vibrations from the NRV travel to the Motor and Pumps, which while working in tandem become misaligned from the exceedingly high shocks emanating from the water-hammer. Hence, the water-hammer due to water back pressure on the non-return valve during the pump operation changeover, was revealed to be the root cause for the frequent failures of the pump. This is a common problem because these pumps generally operate unattended and/or without any monitoring system (including visual inspection). Phenomena such as, in this case, the water hammer in the valve during pump changeover are going unnoticed.
Hence the source of the impact is definitely the operation of the NRV. This is a common problem in many old systems where valves are generally not spring-loaded or
Motor-operated. Spring Loaded Valves as well as Motor Operated Valves are a possible solution. However, even these valves tend to fail.
Tero et al [7] showed that failure in the NRV can be detected by monitoring the shaft torque behaviour during the system shutdown and by comparing this instantaneous shutdown behaviour to separately stored reference behaviour for the pump. This method assumes that the pump shutdown behaviour remains constant if the NRV is operating correctly. The research involved the implementation of an algorithm that would aid a soft sensor arrangement in the detection of NRV failure. The sensors detected the pump-motor shaft as well as the speed of rotation of said shaft, along with a VSD arrangement and flow sensors. This allowed the researchers to test pump shutdown conditions using different parameters. They observed regular valve shutdown behaviour after certain periods of time that would not lead to reversed impeller rotation in normal circumstances.
However, a test which would emulate the occurrence of the NRV being jammed into the opened position, and after a certain point of time, with no prevention of backflow by the now open NRV, the reversed rotation induced a significant torque in the opposite direction of rotation capable of adversely damaging the mechanism between the pump and motor. This could be viewed by the algorithm as a possible reason for pump failure, i.e., the failure of the NRV.
Valve condition monitoring is thus, just as important, if not more important than the maintenance of pump and motor units. They are directly responsible for the correct performance of any pumping system and must be relied upon for their proper functioning. Thus, a positive torque reference due to reverse flow is to be viewed by the algorithm with readings from the VSD as visible evidence for pump shutdown behaviour and can also be used as criteria to monitor valve conditions.
-
Pressure Losses For Fluid Flow in Curved Pipes
Results pertaining to the study of the flow of water in smooth- walled, large-radius curved pipes for the viscous and turbulent regimes over a range of Reynolds numbers from 500 to 60,000. Two series of tests were made by Garbis et al [8]. In the first series pressure losses were measured on ten bends, and concurrent measurements were made on the downstream tangents. The greater part of the paper is devoted to a consideration of the results in the viscous flow regime. In this part of the investigation care was taken to reassure parabolic velocity distribution, at the entrance of the bends. Empirical formulas are given for the effective resistance coefficient of an entire bend and for the downstream tangent. A method of computing to a first approximation the length of curve required for the establishment of the velocity distribution characteristic of a curved pipe is obtained. Critical numbers for bends of different curvature ratios when the entering flow is laminar are briefly discussed. Average values for the relative increase in resistance in a bend as compared with straight pipe in the regime of turbulent flow are given for the range of Reynolds numbers from 40,000 to 60,000.
-
Evaluation Of Friction Losses In Pipes And Fittings Of Process Engineering Plants
The influence of flow rate (Q) on the head loss (hL) in pipes and fittings was studied on different sizes or diameters (D) of pipes fitted with gate valve, 45 and 90 degree bends using water as process fluid. Sizes of pipes ranged from 25 to 100 mm while the fluid flow rates ranged from 0 to 50 m3/h. Hazen-Williams, Darcy-Weisbach, and Poissellis methods were used to estimate friction losses. The results showing increasing D of the pipe and decreasing the hL in the pipeline, gate valve, 45 and 90 degree elbows, entry and exits to pipes are presented, Ntengwe et al [4]. The outcomes of increasing Q with increasing exponential values of hL regardless of D of pipe also presented. Consequently, a variety of selections can be made amongst transporting process fluids using small D pipes (50>D>25 mm) and Reynolds (Re) numbers in the laminar region and large D pipes (100>D>50 mm) using Re numbers in turbulent regions.
-
Experimental Methods to determine NPSH
Experimental investigations concerning cavitation in radial flow pump for three different leading-edge profiles of the vane were carried by Kumaraswamy et al [9] out in an open circuit system. The operating condition of the radial flow pump under cavitation case was understood by measurement of noise and vibration along with the pump parameters for various speeds and flow rates. The consequence of the experimental results revealed that the noise and vibration were superior predictors
The boundary conditions utilized for solving the problem comprised of:
-
no-slip along the channel wall,
-
uniform velocity at the inlet,
-
uniform pressure at the outlet, and
-
isothermal walls.
Two three-dimensional parametric models of the Tesla-type valve have been proposed to desribe as many valve shapes as possible. Based on the models the effects of the valve aspect ratio on its performance have been numerically investigated in terms of the diodicity and the relationships between the pressure drop and the flow rate, for Reynolds number Re up to 2000. The predicted results show that for the same hydraulic diameter, the valves with higher aspect ratio will give better performance, that is, higher diodicity Di, and hence will improve the flow control ability of such valves. However, simulations based on models shown should be also carried out to obtain a more complete understanding of the effect of the valve's shape on its performance. In Porwal et al [11], The effectiveness of the tesla valve as a thermal diode and fluid is determined by the temperature difference across its structure like inlet/outlet cross-sections for each flow.
Pressure Diodicity:
it is the ratio of total pressure drop in the reverse direction to that in the forward direction (Dip) Zhang et al [12]
of inception and development of cavitation.
Determination of Net Positive Suction Head (NPSH) value in the range of best efficiency is important to certify correct operation of centrifugal pumps and fluid networks. Empirical formulas of NPSH determination including the specific speed, head coefficients accounting the valve specifications. The
p
=
r
=
f
2
=
4
0.5
formulation accounting friction including viscosity, diameters and velocities enables to determine the NPSH correction factor during the pumping of viscous fluids. Obtained results show that the NPSH correction factor depends on the specific speed.
Thermal Diodicity:
= { }
NPSH correction factor is also compared with those obtained by Nelik et al [10]. This comparison enables the validation of a formula for the examined range of NRV and centrifugal pump models. This new formula facilitates manufacturers and users the NPSH prediction of standard NRV and centrifugal pumps whatever the viscosity of the fluid.
it is defined as the ratio of the average Nusselts number (Nu) for the flow in the reverse direction to that of flow in the forward direction (Dit).
¯
Nur
NPSH
= (
2
+ )
t =
¯
Nuf
A
2
-
-
-
RESULTS AND DISCUSSIONS
-
Parameters for 3D fluid flow through a Tesla valve
The three-dimensional stream through the valvular conduit was assumed to be:
-
at steady-state;
-
incompressible, Newtonian, single-phase with constant, temperature-independent properties;
-
laminar; and
-
independent from gravity (i.e., the flow was set as to be perpendicular to gravity vector).
-
Head loss in pipes valves elbows and bends
From the experimental study conducted by Ntengwe et al [4], it was concluded that hL decreased with the increase in D of pipes but increased with the increase in velocity or Q regardless of size of pipe. However, exit and entry losses increased with the decrease in D of pipe and increased with increase in Q. The 25 mm pipe produced the highest level in comparison with other pipe sizes. All changes in pipe
dimensions were found to contribute to the hL dependent on D of pipe and the Q values.
-
Valve Diodicity and its optimization:
Nguyen et al [13], found that Valve diodicity is inversely proportional to the radius R, and that optimum configurations a, L depend on flow rates.
Nguyen et al identified the design optimization parameters for Tesla valves and studied different valve configurations with numerical software. It was proved that the valve diodicity is inversely proportional to the radius R, and that optimum configurations (a, L) depend on flow rates. They also deduced the formulas for optimum values of a and L for different flow rates.
-
Diodicity and its phases
Saravann et al [14] found the large difference in mass distribution in both valve channels between forward and reverse flow indicated that diodicity is produced in two-phase flow.
-
Variable speed drives and their effects on fluid handling
[7] created two alternative VSD (variable speed drive) -based methods which were proposed for detecting a malfunction in the nonreturn valve operation. It was concluded that automatic monitoring of non-return valve operation was possible with a variable-speed drive that was able to monitor the fluid handling system operation during its shutdown. Moreover, this paper introduces how the proposed methods can be implemented into the VSD through its internal programmable logic controller. -
The relationship between optimised valves and pumps
-
discovered a design process for optimizing fixed-geometry valve shape and size was unearthed. Optimization of valve shape using CFD that incorporated automatic search methods was demonstrated on a Tesla-type valve with a significant increase in calculated diodicity compared to a commonly used reference valve within the range of Reynolds number 0 to 2000. The calculated results compared favorably with experiment. A linear system model was used to determine all the valve sizes and membrane thickness for the best pump performance. Estimates made at the manufactured pumps indicated that net weight and stream for a pump with advanced valve shape was very nearly 3 times more noteworthy than that for a cloned pump with valves of non-streamlined shape. This study also evaluated the value of the linear model used, it was originally developed for silicon/Pyrex fabrication, but was used successfully in this study for designs in acrylic/ polycarbonate, which have significantly different material properties. And the basic simplicity of fabrication for NMPV pumps was demonstrated by the ease with which they were able to fabricate pumps using new materials.
-
-
Vortex diodes and their behaviour
-
found that fluidic resistance of the flow part of a vortex diode and diodicity were found to be seriously unstable considering the Reynolds numbers were less than 3000.
Fluidic diodes have been discovered to be useful for Reynolds numbers meeting the condition of the self-similarity resistance. In vortex diodes the self-similarity area is at Reynolds numbers exceeds 3000.
The experimentally achieved Diodicity of the vortex diode is far less when compared to the estimated one.
-
-
Various flow rates for optimized Tesla valves
Nguyen et al [13] identified the design optimization parameters for Tesla valves and studied different valve configurations with numerical software. It was proved, with simulation results, that the valve diodicity is inversely proportional to the radius R, and that optimum configurations (area, Length) depend on flow rates. What was also deduced were the formulas for optimum values of a and L for different flow rates.
-
The water hammer effect
Stalin et al [15] discovered the effect of a water hammer is studied and the process of reduction of the energy of the fluid is done. The major pressure surge wave is contained within the accumulator and the braked shutting of the NRV (slider crank mechanism) prevents the rapid closing of valves which practically prevents the causing of water hammer. This saves the adverse effects of the water hammer and protects the valves and pump impellers from damage and results in the life of the pipe and pumps impeller to increase.
-
Models for simulation in Tesla valves
Thompson et al [16] used the three-dimensional CFD, and found the diodicity across a Tesla valve for larger Reynolds number was estimated and compared with available experimental data. Three transitional/turbulent models bundled inside ANSYS FLUENT were utilized and compared, including the: k- model, k-kL- model, and SST k- model. The main conclusions are as folows:
-
For ReD > 500, 3D CFD with turbulence modeling via the k-kL- model or SST k- model provided more accurate fluid simulation in a Tesla valve relative to standard (laminar) 2D CFD.
-
For Reynolds numbers up to 1,500, the k-kL- model performed the best providing only a 6% maximum percent relative error with available experimental data. For Reynolds number up to 1,000, the model was found to be superior, with 0% percent relative error.
-
-
Topology optimization in Tesla valves
Yongbo et al [17] created the design procedure of NMP fluid resistance micro valves through the topology optimization method and complex FEA tools that had been presented. In order to find a suitable optimization procedure, the ratio of fluid work is used to demonstrate the diodicity of a valve. The optimized valve had a different layout when compared with the typical Tesla valve, and the diodicity of the optimized valve has been improved marginally.
-
-
APPLICATIONS
-
The ability of a Tesla valve to function as a mini-to- micro-type heat exchanger, thermal diode, and/or check valve can benefit several thermal/flow control applications. Porwal et al [11]
-
Tesla valves are used in many different applications. They are often placed on the outlet side of a pump, to protect the pump from backflow which causes impeller failure most of the time.
-
Tesla valves are very often used in HVAC-systems (Heating, Ventilating and Air Conditioning-systems). HVAC-systems are e.g. used in large buildings, where a coolant is pumped many storeys up. The Tesla valves are here installed to make sure that the coolant is not flushing back down. Rosa et al [18].
-
Tesla valves are used extensively in Jet engines, Nanofluids, heart stents and valves (in nature they will be seen within the valves of the guts. The ability of a Tesla valve to function as a mini-to-micro-type device, thermal diode, and/or check valve can benefit several thermal/flow control applications. Porwal et al [11]
-
The use of Tesla valves for flow control and rectification in mini and microfluidic applications is with regards to their passive operation and no- moving-parts design. Tesla valves are incorporated in manifold applications, for instance they're often placed on the outlet side of a pump, to guard the pump from backflow which results in frequent impeller failure
-
Centrifugal pumps, the foremost common form of water pumps, aren't self-priming, and so Tesla valves are essential for keeping water within the pipes.
-
Pulse Jet engines use Tesla valves extensively so as for combustion to occur in controlled pulses. The obstructive design of a Tesla valve in a very certain direction leads to the jet engines to make hot streams of gases in consecutive and tightly controlled loops.
-
Tesla valves are used in microfluidic applications and when designing fire-safety equipment. If combined with other pressure relief valves it can lower the demand for the performance on the pressure relief valves.
-
-
CONCLUSION
The most promising application of Tesla-type valves is for flow rectifying elements in a membrane micro pump to produce a net one-way flow. The chamber with larger depth in such a micropump can realize a swept volume adjust function, which gives the flexibility of the flow control of the micropump. This brings forward the problem of the aspect ratio of the valve since it is connected directly to the chamber since the valve in itself is a channel.
From the aforementioned studies, it is clear that the traditional, mechanically actuated NRVs are at times, a liability in pumping and fluid processing applications. When a scientific system becomes widely accepted, no matter how incorrect or
dangerous it may be, it has a way of becoming robust, and is then difficult to replace with better alternatives, owing to the complex industrial manufacturing processes backing that system. It is far too early to comment on the replaceability of regular mechanically actuated NRVs, but it is also too late to state that the pumping industry needs to make significant improvements in the use of their apparatus, as the water hammer effect is single-handedly being named as a culprit in pump failure, and the NRV used bears the burden of that blame.
In order to be just as widely accepted as traditional NRVs, Tesla valves, apart from being completely robust in their construction, demand a significant update in their design and performance in order to compete with existing industry workhorses. The internal baffles of the Tesla valve need upgrades, including a revolutionary new design, as Tesla valves yet have not been able to completely stop the flow of fluid passing through them, the geometry required to do so would require the use of the most modern CFD techniques and manufacturing prototyping methods as well as the use of traditional fluid mechanics to decide upon a construction without any moving components so as to ensure a complete cancellation of backflow
-
REFERENCES
-
Gamboa, Adrian R., et al. Improvements in Fixed-Valve Micropump Performance Through Shape Optimization of Valves. Journal of Fluids Engineering, vol. 127, 2005, pp. 339 – 342. DOI: 10.1115/1.1891151g.
-
Khabarova, D. F., et al. Experimental Investigation of Fluidic Diodes. International Conference on Industrial Engineering, vol. 206, 2017, pp. 93 – 98, www.sciencedirect.com.
-
A. A. Kulkarni, and V. V. Ranade. CFD Simulation of Flow in Vortex Diodes. American Institute of Chemical Engineers, vol. 54, 2008.
-
F. W. Ntengwe, et al. Evaluation Of Friction Losses In Pipes And Fittings Of Process Engineering Plants. INTERNATIONAL JOURNAL OF SCIENTIFIC & TECHNOLOGY RESEARCH, vol. 4, no. 10, 2015.
-
Akira Goto, et al. Hydrodynamic Design System for Pumps Based on 3-D CAD, CFD, and Inverse Design Method. Journal of Fluids Engineering, vol. 124, 2002, http://fluidsengineering.asmedigitalcollection.asme.org/.
-
Elbhbah, Keri, et al. A Non-Return Valve: A Possible Source for Pump Failure. pp 1 – 3 ed., Manchester, Maintenance and Engineering, March 1, 2006.
-
Ahonen, Tero, et al. Monitoring of Non-Return Valve Operation with a Variable-Speed Drive. LAPPEENRANTA UNIVERSITY OF TECHNOLOGY School of Energy Systems, 2014, pp. 3 – 7.
-
Garbis H, et al. PRESSURE LOSSES FOR FLUID FLOW IN CURVED PIPES. Journal of Research of the National Bureau of Standards, vol. 18, 1937.
-
S. Christopher, and S. Kumaraswamy. Identification of Critical Net Positive Suction Head From Noise and Vibration in a Radial Flow Pump for Different Leading Edge Profiles of the Vane. Journal of Fluids Engineering, vol. 135, 2013, http://fluidsengineering.asmedigitalcollection.asme.org/.
-
Nelik, L., 1999, Centrifugal and Rotary Pumps: Fundamentals With Applications, CRC Press, Boca Raton, FL.
-
Porwal, Piyush, et al. Heat transfer and fluid flow characteristics in multistaged Tesla valves. An International Journal of Computation and Methodology, vol. 73, no. 6, 2018, pp. 347-364,
: https://doi.org/10.1080/10407782.2018.1447199.
-
S Zhang, et al. PERFORMANCE SIMULATIONS OF TESLA MICROFLUIDIC VALVES. 2007, pp. 1-5, : http://proceedings.asmedigitalcollection.asme.org.
-
Nguyen, Nam-Trung, and T-Q Truong. Simulation and optimization of Tesla valves. Nanotech 2003, vol. 1, 19 December 2009, pp. 1-5. ISBN 0-9728422-0-9.
-
Saravanan, K. G., and N. Mohanasundara Raju. Structural Analysis of Non Return Control Valve using Finite Element Analysis. International Journal of Engineering Research & Technology (IJERT), vol. 4, no. 4, April 2015, pp. 1 – 5, ISSN: 2278-0181.
-
Stalin, T., and C. Manivannan. Manipulation of Water Hammer Problem by Modification of NRV Valve. AMAE Int. J. on Manufacturng and Material Science, vol. 1, no. 1, May 2011, pp. 12 – 13, DOI: 01.IJMMS.01.01.60.
-
Thompson, Scott M., et al. TRANSITIONAL AND TURBULENT FLOW MODELING IN A TESLA VALVE. Proceedings of the ASME 2013 International Mechanical Engineering Congress & Exposition, November 15-21, 2013,, pp.1
– 8, https://www.researchgate.net/publication/267596828.
-
Deng, Yongbo, et al. OPTIMIZATION OF NO-MOVING PART FLUIDIC RESISTANCE MICROVALVES WITH LOW REYNOLDS NUMBER. IEEE, 2010, pp. 68 – 70, 978-1-4244- 5764-9/10.
-
Rosa, A. Dalla, et al. Method for optimal design of pipes for low- energy district heating, with focus on heat losses. ELSEVIER, vol. 36, 2011.