
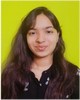
- Open Access
- Authors : Akruti Amol Ingole , Anirban Goutam Mukherjee , Piyush Jagdish Balgote, Sampada Prakash Pendse , Surbhi Balwant Dhoke, Uddesh Ramesh Wanjari
- Paper ID : IJERTV10IS030216
- Volume & Issue : Volume 10, Issue 03 (March 2021)
- Published (First Online): 30-03-2021
- ISSN (Online) : 2278-0181
- Publisher Name : IJERT
- License:
This work is licensed under a Creative Commons Attribution 4.0 International License
A Review on Carcinogenic Heavy Metals
Akruti Amol Ingole1 , Anirban Goutam Mukherjee4 , Piyush Jagdish Balgote6 , Sampada Prakash Pendse2 , Surbhi Balwant Dhoke5, Uddesh Ramesh Wanjari3
1 B. Tech Biotechnology, Priyadarshini Institute of Engineering and Technology, Nagpur, Maharashtra, 440019
2 M.Sc Biochemistry, Department of Biochemistry, RTM Nagpur University, Nagpur, Maharashtra, 440033
3,5 M.Sc Biochemistry, Kamla Nehru Mahavidyalaya, Nagpur, Maharashtra, India, 440024
4 M.Sc Biotechnology, Vellore Institute of Technology, Vellore, Tamil Nadu, India, 632014
6 M.Sc Molecular Biology and Genetic Engineering, RTM Nagpur University, Nagpur, Maharashtra, India, 440033
Abstract Cancer is the leading cause of various incidences of mortality, morbidity, and premature death worldwide. Toxic metals like arsenic, chromium, nickel, aluminium obstruct several physiological processes, including the central nervous system (CNS) and renal functions, having specific interactions with system cells, tissues, and organs. Most importantly, these metals result in the induction of tumors leading to cancer. This review focuses on various heavy metals in the induction of complex disorders and cancer. This work also aims to provide a brief understanding to ordinary people about the most simple day-to-day activities that can get us exposed to heavy metals.
Keywords Cancer, tumor, heavy metal, toxic, mortality
INTRODUCTION
Nowadays, over half of world populations face newly diagnosed cancers, and 70% of all cancer death occur in developing and undeveloped countries [1,2]. The prevalence of cancer varieties is multifactorial polygenic diseases that may vary depending upon the environmental factors and genetic susceptibility because they have identifiable heredity factors. Rapidly changing lifestyle and diet adaptation may influence the heritability of variant phenotypes dependent on the nutraceutical's supplementation for their expression [3,4].
To date, relevant information about carcinogens is available, and many of these agents have already been identified [5]. However, many potential carcinogenic heavy metals are present in food and beverage items and are assumed to be safe when consumed by humans that cause serious health problems. In this regard, Regular consumption of daily requirements may expose them to heavy metals in drinking water which pose the greatest threat to public health. Daily life's basic necessity is water, which is the primary source of heavy metals that contaminate the surface and ground waters by industrial sewage and agricultural runoff [6]. Some people may not afford the purified mineral water, and they consume tap water which is the direct source of contaminated heavy metal mixed water [7].
According to several research studies, the concentration of these carcinogenic groundwater elements is high in some countries, including India, Bangladesh, and Argentina [8,9]. These elements are known to induce cancer of different types leading to genetic and epigenetic effects [10]. Epigenetic mechanisms play a prominent role in the process of carcinogenesis. Such events include reversible modification
of CpG islands of gene promoters and histone proteins that affect somatic cells and germ cells' gene expression level and cause indirect changes genetically [11,12]. Mammalia genes account for nearly 40% of CpG islands (5-CG-3 sequence). The C5 position of cytosine residue results in hypermethylation and hypomethylation, which involved the inhibition of the expression of tumor suppression of genes or an increase in oncogenic gene expression, jointly contributing to cancer development and progression [13].
Deamination of methylated cytosine base may produce a thymine base, resulting in a specific transition mutation in CpG island, for example, in p53 (tumor suppressor gene or as guardian of the genome), the chromatin configuration changes due to histone proteins' hypermethylation [14]. Corresponding alterations in growth control genes such as DNA-repair genes, oncogenes, protooncogenes, apoptotic genes combine to dictate the cellular phenotype and differentiation [12,15]. Comparing these epigenetic effects caused by the following elements in cancer concerned genes shows the impact in biological systems.
I. TOXICITY AND CARCINOGENICITY
The degree of toxicity can be acute and chronic. Toxic metals obstruct several physiological processes, including the central nervous system (CNS) and renal functions, having specific interactions with system cells, tissues, and organs. Like Mercury, Gold, Platinum, Beryllium, chromium, and Nickel, a few metals may induce type I, II, III, or IV hypersensitivity [16]. The half-life of metals within tissues is very important to learn about its toxicity. For Hg, the half-life is 60 -70 days [17], Cd is 10-20yrs [18], and Pb is 10yrs [19] depending upon the type of tissue, for example, lead has a half-life in soft tissues for few weeks however it is of 20yrs in bone tissue.
Another factor is chemical complexity that may alter the metals' pharmacokinetic properties, including the ability for absorption and distribution to reach the cellular and intracellular targets [20]; chemical forms (elemental, organic, inorganic) strongly affect the route of exposure, bioavailability, and toxic profile. Organic forms are highly lipophilic and quickly cross the biological membrane (Blood- Brain barrier and Gastrointestinal wall, Placental membrane). Organic forms of mercury metal (ethyl mercury, phenylmercury, and methylmercury) can accumulate in
lipophilic tissues, crossing the BBB (Blood-brain barrier) give rise to neurotoxicity. Significant toxic effects are on CNS and neuron anatomical regions. Signs and symptoms include dysarthria, constriction of the visual fields, paraesthesia, ataxia, and hearing loss [21]. Inorganic forms are salts of metals (e.g., vapors of atomic Hg and salts HgO, HgS, and Hg2Cl2) having hydrophilic nature, mainly cause renal toxicity [17,22].
Toxic effects of metals are often tissue-specific due to specific proteins that selectively bind metals like Metallothionein (MT) [23]. Cd metal in the liver can bind to GSH (glutathione) and get excreted out of the liver with bile and bind to MT and form Cd-MT complex, representing metal accumulation. Physical and chemical similarities, some nonessential metals that are not required during physiological processes, can compete with essential metals ( requisite metals for numerous biological processes), disturbing the homeostatic ionic equilibrium [24].
Heavy metals are known to demonstrate a high affinity for aminic, thiolic, phosphoric, and carboxylic groups of organic compounds present in inactive or reactive sites of biological proteins and nucleic acids. Heavy metals mainly function as carcinogens or co-carcinogens, producing a cascade of biochemical events during various metabolic processes such as replication, transcription, and DNA repair systems. Ni, Cr, As, Cd, Be, and Pb metals are classified as potential human carcinogens based on epidemiological evidence [25]. However, the exact mechanism of genotoxic action is not entirely understood. For example, arsenic (As) carcinogenicity involves cytotoxicity followed by regenerative cell proliferation; this is due to the formation of reactive metabolite DMAII (Dimethylarsinous acid), which leads to oxidative damage or binds with critical urothelial sulfhydryl groups [26].
I. CARCINOGENIC HEAVY METALS
-
Arsenic (As)
Arsenic is also called an epigenetic carcinogen metalloid. Arsenic has two states: trivalent arsenite has mre carcinogenic properties than pentavalent arsenate [27,28]. Trivalent arsenic has a high binding affinity to thiol groups of proteins and reduced glutathione (GSH) [29]. Uptake of drinking water for a long duration containing low arsenite levels induces carcinogenesis in skin, lung, bladder, and kidney tissues, resulting from alteration of multiple signaling pathways [30]. Exposure to reduced arsenic-induced reactive oxygen species formation is an unavoidable reaction of normal cell metabolism [31].
Arsenic carcinogenesis plays a role in increasing the genotoxicity of other carcinogens, including UV and ionizing radiations, alkylating agents, or oxidants. UV-radiations induce non-melanoma skin cancer. Strands of DNA exposed to photons of ultra-violating radiations (A & B) break, and CPDs (Cyclobutane pyrimidine dimers) are formed [32,33]. UV radiation can activate zinc finger motifs of protein PRAP
(Protein family poly (ADP-ribose) polymerase) of PARP 1 family has an essential role in the regulation of NER (nucleotide excision repair) [34,35]. Around 40- 60% of arsenic intake is excreted into the urine, and the majority is composed of demethylated arsenic [36,37].
-
Cadmium (Cd)
It is a toxic heavy metal naturally present in the environment as a pollutant from industrial and agricultural sources. Atmospheric pollution and the use of Cd-containing fertilizers are found to contaminate background levels in soil, farmland [38]. In non-smoking individuals, Dietary Cd (found in Shellfish, offal products, certain seeds, cereals, potatoes, roots, crops, and vegetables are the primary sources, roughly 80%) is the major source of human exposure to this heavy metal. The average Cd intake from food varies between 9 and 25 mg/day in the US and Europe and between
19.7 and 35.4 mg/day in Asia. Smokers usually absorb a similar Cd from cigarettes as for food ingestion (1-3 mg/day) [39]. The half-life of cadmium retaining capacity in the kidney for many years (half-life: 10-30 years) and the concentration is proportional to that in the urine [40].
Biomarkers of cadmium exposure are blood, urine, hair, and nails. Information regarding Cd's recent absorption in the blood can be taken into account, and its concentration is independent of tissue deposition [41]. Serum Cd normal range in healthy subjects is 0.1-0.5 mg/L [42]. Cd accumulation of Urine-Cd in the kidney mainly reflects its life-long exposure [40]. Hair and nails provide long-term information and are easily accessible for non-invasive sampling. However, some data indicate that Cd accumulates in organs and blood rather than skin appendages [43,44].
However, the results are often inconsistent and sometimes are dependent on the different Cd sources considered (occupational vs. non-occupational settings). Accumulated epidemiological evidence has established a link between Cd exposure and prostate cancer risk/mortality. Sawada et al. evaluated Cd consumption's relationship at levels observed in a general population with all cancers' risk. Furthermore, many factors, including smoking, diet, other heavy metals, pollutants, and lifestyle, may mystify the results and amplify a positive relationship [45].
The mechanisms involved in Cd carcinogenesis are complex and only somewhat known. Some of these mechanisms may include induction of oxidative stress and DNA damage, enhanced proliferation and depressed apoptosis, altered DNA repair, and hormone-like actions in the prostate model [46,47].
Notably, Malignant transformation by chronic exposure to low Cd concentrations can also be induced in human immortalized prostate cells [48]. These cells behave as poorly-differentiated adenocarcinoma cells and acquire the ability to form colonies and grow in nude mice, a helpful model, to investigate the molecular mechanisms involved in Cd-induced carcinogenesis in humans' prostate. Several pieces of evidence indicate that both androgens and estrogens'
hormone-mimicking Cd's actions are highly suspected of playing a role in Cd-dependent prostate carcinogenesis through complex and not wholly understood mechanisms [49,50]. Long-term Cd accumulation can raise the possibility that the prostate may induce multiple mechanisms that may favor carcinogenesis [51].
-
Aluminium
Aluminum exhibits many applications in different areas, including food additives and cosmetics. It induced carcinogenesis as it has a binding ability to the estrogen receptor (ER) and imitates estrogen functions, therefore termed metalloestrogens (ME) [52]. Aluminum has an affinity for both cell surface estrogen receptors and nuclear estrogen receptors and ERE; this results in the activation of both ER signal transduction [53]. ME triggers the expression of genes that contain ERE (Estrogen Responsive Elements) on their promoters. ERE increases breast cells' proliferation in the mammary glands, increasing replication error in cancer-related genes [54]. This element is used in the antiperspirants that block the odor and sweat when applied on the skin around underarms and breast areas; when effectively not washed, continuous exposure of these salts of aluminum in that area enhances the risk of cancer [55].
In addition to breast cancer, estrogen can activate telomerase gene expression as a gene containing ERE in ER- alpha positive cell results in endometrial cancer [56]. The epigenetic effects of aluminium take place through the binding of trivalent (Al3+) to the phosphate groups of DNA under physiologic pH, thus changing DNA topology from B to Z in (CCG) 12 repeat regions [57]. The expansion of the triplet repeats is named "dynamic mutation." A minimum of 5-10 triplet-repeats increases the probability of hairpin formations, mainly in the lagging strand. These hairpin structures lead to replication slippage and genomic instability due to the inappropriate DNA polymerase movement, causing deletion mutations. Expansion of these repeats tends to form more than 200 copies of leads to excessive methylation of cytosines in the promoter of the FMR1 gene, resulting in fragile X syndrome [58].
-
Nickel
Nickel compounds are water-insoluble, including nickel sulfides, disulfide, and oxides permeable to the cell membrane are very potent carcinogens [59]. Drinking water is the primary nickel source [60]. It induces carcinogenesis through interruption of the biological process, including DNA hypermethylation, mutation, ROS generation, modification by inhibiting histone proteins (H1, H2, H3), a substantial increase of the ubiquitination of H2A, H2B and H4 acetylation, and converting protooncogenes to heterochromatin. Therefore, nickel plays a vital role in the suppression (silencing) of genes and the other genes that are involved in carcinogenesis pathways [61,62].
-
Chromium
The trivalent form of chromium is an epigenetic carcinogen factor since it can form stable compounds with
macromolecules such as DNA and cysteine of proteins and glutathione [63]. The trivalent form cannot pass through the cell membrane, but its hexavalent salts can pass the cell membrane. Hexavalent chromium (reduced form) interrupts the fundamentals process of replication by breaking the single strands, DNA inter-strands cross-links form (ICLs), and oxygen radicals, thus inducing apoptosis. Its carcinogenicity is imposed through hypermethylation of CYP1a1 promoters in lung epithelial cells and fibroblasts [64].
CYP1A1 plays an essential role in the metabolism of carcinogens such as polycyclic aromatic hydrocarbons (PAHs) and heterocyclic amines; they are widely distributed in our environment through automobile exhausts, charcoal- broiled cooking, cigarette smoke, and industrial waste. CYP1A1 inhibits PAH carcinogenesis. Thus, inhibition of CYP1A1 by chromium leads to the production of a PAH [65].
Poly Aromatic Hydrocarbons play an essential role in activating cytosolic ligand-activated transcription factors named aromatic hydrocarbon receptor (AhR) [66]. It forms the PAH-AhR complex, dissociation of complex inside nucleus and AhR binds with a nuclear partner (Arnt), this will interact with the DRE of CYP1A1 gene. CYP1A1 gene activates, and its expression is causing bioactivation of exogenous pro-carcinogens of both hepatocellular and lung carcinomas [67].
Cr induced apoptosis by phosphorylating the P53 gene at serine 3932, down-regulation of several anti-apoptotic genes from the Bcl2 family and bax, these events adversely destruct the mitochondria and release the cytochrome C protein [68,69]. Cr interferes with ATM regulation of apoptotic pathway and acts as the MAPK kinase in the MAPK kinase pathway, increasing survival/proliferation in a dose- dependent manner [70,71].
-
Selenium
It is an essential trace element with a narrow range between toxic and therapeutic doses. Therefore, its activity is highly dose-dependent. Selenium is detoxified by methylation through SAM (S-Adenosylmethionine) pathway as arsenic does. This increase in the competition between these two elements for methyl groups leads to increased arsenic retention in tissues [72]. SeC (Selenocysteine) have shown more anti-carcinogenicity in lung cancer model system than another inorganic compound. In contrast with selenomethionine, Selenocysteine decreases cellular reduced thiol agents like N- acetylcysteine (NAC) and GSH, thus increasing the ROS formation [73].
Proteins containing selenium elements induce apoptosis pathways through caspase activation. Another form of selenite and selenomethionine predominantly activates apoptosis through P53 activation and anti-apoptotic inactivation. They produce ROS (reactive oxygen species), ROS disturbs ATM and ATR apoptotic pathway and can activate p53 in MCF-7 human breast cancer cells and human prostate cancer [74]. The Se- MSC (Se-methyl
selenocysteine) shows its anti-carcinogenic activity; on the other hand, its up-regulation of some extracellular matrix proteins such as collagen type 6 alpha 1 (COL6A1) and collagen type 4 alpha 5 (COL4A5) genes in a human prostate cell line [75].
-
Zinc
Zn (essential trace element) is involved in vital bioprocesses. Zn's main source is diet (primary food sources are milk, flesh food, cereals, and vegetables), and its levels in animal products depend on the soil and water concentrations where the animals are cultivated. The recommended daily intake for men ranges from 5.2 to 16.2 mg/day [9]. Serum Zn levels in healthy individuals are maintained by homeostatic mechanisms within a narrow range (12-15 mmol/l, 78-98 mg/dL) [76].
The only biomarker of Zn status in the population can be drawn from the level of Zn concentration in serum, as recommended by WHO/UNICEF/IEA/IZiNCG, and reflects dietary zinc intake and predicts responses to zinc metal interventions in various biochemical processes.
It had been shown that there is a positive correlation between Zn dietary intake and prostate carcinogenesis in a large multicentre hospital-based case-control study conducted in Italy [77]. Several other factors should be considered as they alone or may affect serum Zn levels like nutritional status, race, clinical conditions, lifestyle, chronic stress, aging, inflammation [78,79].
Here, all the data currently available from various epidemiological studies aim to refine the correlation between Zn concentrations in various biological samples (dietary, serum, toenails, hairs) are inconclusive. Therefore, in the future, for conclusive results, we likely need more extensive prospective epidemiological studies with repeated collection overtime which better reflect long-term exposure [80,81].
Zn has a fundamental role in human metabolism. Beyond Iron, Zinc is the central metal ion present in the human body and has three major biological roles: structural, regulatory, and catalytic [82]. In mammalian biological systems, zinc is present in three forms: the first (bound to proteins is approximately 54% of the Zn pool and is the non- exchangeable and non-reactive part); the second (loosely bound to a ligand and is an exchangeable reactive pool of Zn (44.7%)); and the third (the reactive pool of Zn or the free Zn (less than 1%)). It stabilizes the structure of many proteins and DNA, RNA, and ribosomes, is a component of more than 3000 transcription factors, and is a cofactor of various enzymes [83].
Suggesting that Zn is essential for prostate functions, a study shows zinc concentration varies in different organs, but the highest concentration is found in the prostate tissue three times higher than in other mammalian cells and100 times higher than in plasma [84]. Some of the data correlate with the zinc's different intracellular content. High levels of Zn and citrate are essential constituents of prostatic fluid
principally produce by the human prostate gland. The epithelial cells of the gland's peripheral zone. Its import is regulated by the ZIP1 gene [85]. A plasma membrane transporter is localized in the basolateral and apical cell membrane. Since its uptake mainly determines total intracellular Zn from the extracellular fluid, the cells need to have functionally active ZIP1 transporters. ZIP1 expression is high in normal prostate cells, while it is downregulated in prostate cancer cells owing to epigenetic silencing of ZIP1 [86].
The cellular concentrations of zinc in prostate cells, maintained by the Zn influx, have necessary inference on cell metabolism in both standard and cancer prostate cells [87]. Undeniably, in normal prostate cells, the highest intracellular Zn concentrations have been observed in the intra- mitochondrial compartment. At this level, the inhibition of the mitochondrial aconitase. It is induced by zinc [76].
In contrast, in prostate cancer cells where the intracellular zinc concentrations are low, the conversion of citrate to isocitrate is fast and cannot accumulate, and this event is a more energy-efficient mitochondrial activity of prostate cancer cells. Zinc levels are much lower In androgen- independent prostate cancer than those in an androgen- dependent state [88].
-
Copper
Many essential enzyme systems require copper minerals to function correctly, and disruption of Cu homeostasis is linked with several complex diseases [89]. Good sources of dietary Cu are liver and other organ meats, seafood, nuts, and seeds. The median intake of Cu from the diet ranges from 1.0 to 3.0 mg/day for adults. Traditional Cu homeostasis indicators are serum, hair, and nails Cu levels [90]. Studies suggest that high Cu levels may increase prostate cancer risk because Cu's concentration for the cancer group was higher than that for the healthy group [91,92,93].
Copper is present as a cofactor for the essential enzymes such as cytochrome oxidase, superoxide dismutase, ascorbate oxidase, and tyrosinase [94]. Dyshomeostasis of Cu plays a significant role in human diseases, including cancer. Different Cu dysregulation mechanisms can exert toxicity through, including protein interference, proteasome inhibition, and displacing other functional metals (such as Zn and Fe). It has a strong inference in angiogenesis, a critical step in cancer progression and proliferation, and it participates as a cofactor of several angiogenic factors (VEGF, FGF, ILs) [95]. At the intracellular level, Cu regulates various signal transduction pathways associated with insulin-like growth factor-1 (IGF-1), mitogen-activated protein kinases (MAPK), protein ubiquitination, NF-kB, hypoxia, epidermal growth factor (EGF) [96,97,98]. Increased Cu ions have been detected in different cancer tissues, including prostate, breast, colon, lung, and brain. Some studies reported high Cu levels (2-6 fold) in prostate cancer patient cell lines (in vitro), and prostate cancer cells express increased levels of hCTR1 transporter, thus up- regulating Cu influx [99]. Increased Cu uptake in AR-
positive cancer cells has also been linked to the androgen receptor (AR) as androgen treatment [94].
-
Iron (Fe)
Iron is the most abundant transitional metal in the human body. Dietary Fe is found in heme (most easily absorbed) and ionic (non-heme) forms and the primary source is food intake, especially from meat and legumes. High dietary Fe intake can be a source of reactive oxygen species (ROS) and potential carcinogens, such as N-nitroso compounds (NOC), that adversely affect DNA and are linked to several cancers, including prostate cancer. These reports suggest that high levels of Fe in these biological samples may represent a risk factor for prostate cancer [100].
An increased requirement for Fe and Fe regulation proteins are often deregulated in cancer cells and play a significant role during the tumorigenesis [101]. Fe outflux from the cell may be reduced in prostate cancer cells [102], that govern through ferroprotein (a transmembrane transporter protein), and its intracellular decrement of Fe is associated with decreased prostate cancer cell differentiation and a high degree of malignancy. The expression level of ferroprotein increases in benign prostatic hyperplasia (BPH), confirming that ferroprotein plays a role in prostate carcinogenesis [103].
III. HEAVY METALS AS POTENTIAL PREVENTIVE AND THERAPEUTICAL AGENTS FOR PROSTATE
CANCER
-
Inactivation
The elimination and inactivation of metals can prevent deleterious effects. Alternatively, chelating agents can be efficiently administered for various human metal intoxication [104]. The main chelating agents used to treat metal intoxications are ethylene diamine tetraacetic acid (EDTA) [105], dimercaprol (BAL) and BALglucoside [106,107], D- penicillamine,23 and deferoxamine [108], dimercaptosuccinic acid (DMSA) and dimercaptopropionic sulfonate (DMPS) [109].
Despite that these chelating agents are helpful for the detoxification of heavy metals, they have many side effects (, for DMSA and DPMS: gastrointestinal discomfort, for EDTA: renal system toxicity, BAL: hypertension, tachycardia, thrombocytopenia, nephrotoxicity, for D- penicillamine: glomerulonephritis and hypersensitive allergic reactions, etc. Thus, it is prudential to analyze new compounds used explicitly to treat poisoning from toxic metals tolerable by the human body without side effects [106,109].
-
Zinc therapies
Several studies have demonstrated that zinc can be effectively used as a preventive and therapeutical agent in prostate cancer. Zn supplements were linked with a reduced risk of prostate cancer. Ranges of Zn supplements intake up to 100 mg/day were not associated with prostate cancer risk. The intake of supplemental Zn > 100 mg/day and
supplemental Zn for ten years had a more than doubled relative risk of advanced prostate cancer. These data may suggest that Zn's excessive supplementation may have an undesirable effect on prostate health [86,110].
Some of the preclinical studies suggest that Zn may also have complex effects on prostate cancer cells. Indeed, Zn ions elicit apoptotic effects in prostatic cancer cells for short- term and long-term exposure [111]. In prostate cancers, reduced Zn content is found; some studies have addressed the possibility that increasing intracellular influx of Zn ions via transporter ZIP1 could exert tumor-suppressing actions at early stages carcinogenesis [86]. Distressing Zn concentration homeostasis in prostate cancer cells, either by increasing Zn intracellular concentration with clioquinol, chloroquine, and possibly other Zn ionophores or by reducing Zn even further with chelators, deserve further studies in designing new therapies for castration-resistant prostate cancer [82,95].
B.Copper therapies
Interestingly, Cu ionophores seem to elicit selective toxicity against malignant prostate cells. Copper levels may sensitize prostate cancer cells to the action of ionophores, which increase the intracellular Cu and may constantly redistribute intracellular Cu into a cell pool. Therefore, several Cu-coordinating lipophilic compounds such as hydroxyquinolines, dithiocarbamates, and thiosemicarbazones have been proposed as potential drugs against prostate cancer [94].
V. CONCLUSIONS
Notably, some potential risk factors confounders such as exposure to various heavy metals or organic contaminants, dietary variables or nutrients, age, body mass index, alcohol consumption, and other factors such as cigarette smoking may also contain heavy metals trace elements with adverse health effects. At the same time, vitamin supplements may contain trace elements that affect cancer risk. The available evidence suggests that the positive association observed between high Cd exposure, Cu overload, high Fe intake, zinc uptake, arsenic in nails and urine may be a risk factor for prostate cancer. Finally, malignantly transformed prostate cancer cells show clear evidence of Zn and Cu's profound dyshomeostasis that may affect tumor growth, metabolism, and resistance to conventional therapies. The use of ionophores to increase Zn intracellular concentrations appears promising. Cu cell concentrations also appear critical for tumor progression. Both Cu deprivation with chelators and forced intracellular Cu influx may toxic for a prostate cancer cell; overall, the epidemiological studies linking exposure to heavy metals to prostate cancer incidence and mortality are somewhat inconclusive and sometimes contradictory. Nevertheless, overall, these data should be interpreted with caution, and more studies should be performed to confirm the results obtained so far.
REFERENCES
-
Parkin, D.M., Bray, F., Ferlay, J., Pisani, P., 2005. Global Cancer Statistics, 2002. CA: A Cancer Journal for Clinicians 55, 74108. https://doi.org/10.3322/canjclin.55.2.74
-
Kanavos, P., 2006. The rising burden of cancer in the developing world. Annals of Oncology 17, viii15viii23. https://doi.org/10.1093/annonc/mdl983
-
Mishra, S., Singh, R.B., Dwivedi, S.P., De Meester, F., Rybar, R., Pella, D., Fedacko, J., Juneja, L.R., 2009. Effects of Nutraceuticals on Genetic Expressions. TONUTRAJ 2, 7080. https://doi.org/10.2174/1876396000902010070
-
Mudgal, V., Madaan, N., Mudgal, A., Singh, R.B. and Mishra, S., 2010. Effect of toxic metals on human health. The Open Nutraceuticals Journal, 3(1).
-
Chasapis, C.T., Loutsidou, A.C., Spiliopoulou, C.A., Stefanidou, M.E., 2012. Zinc and human health: an update. Arch Toxicol 86, 521534. https://doi.org/10.1007/s00204-011-0775-1
-
Karavoltsos, S., Sakellari, A., Mihopoulos, N., Dassenakis, M., Scoullos, M.J., 2008. Evaluation of the quality of drinking water in regions of Greece. Desalination 224, 317329. https://doi.org/10.1016/j.desal.2007.06.013
-
Leivadara, S.V., Nikolaou, A.D., Lekkas, T.D., 2008. Determination of organic compounds in bottled waters. Food Chemistry 108, 277286.
https://doi.org/10.1016/j.foodchem.2007.10.031
-
Mishra, S., Dwivedi, S.P. and Singh, R.B., 2010. A review on epigenetic effect of heavy metal carcinogens on human health. The Open Nutraceuticals Journal, 3(1).
-
World Health Organization, 2003. Atrazine in drinking-water: background document for development of WHO guidelines for drinking-water quality (No. WHO/SDE/WSH/03.04/32). World Health Organization.
-
Bower, J.J., Leonard, S.S., Shi, X., 2005. Conference overview: Molecular mechanisms of metal toxicity and carcinogenesis. Mol Cell Biochem 279, 315. https://doi.org/10.1007/s11010-005- 8210-7
-
Jones, P.A., Baylin, S.B., 2002. The fundamental role of epigenetic events in cancer. Nat Rev Genet 3, 415428.
https://doi.org/10.1038/nrg816
-
Nikiforov, Y.E., Nikiforova, M.N., 2011. Molecular genetics and diagnosis of thyroid cancer. Nat Rev Endocrinol 7, 569580. https://doi.org/10.1038/nrendo.2011.142
-
Kinzler, Kenneth W.; Vogelstein, Bert (1997). Gatekeepers and caretakers. , 386(6627), 761
763. https://doi.org/10.1038/386761a0
-
Egger, G., Liang, G., Aparicio, A., Jones, P.A., 204. Epigenetics in human disease and prospects for epigenetic therapy. Nature 429, 457463. https://doi.org/10.1038/nature02625
-
Mohammadi, A., Gohar, A.V. and Shakibaie, M.R., 2008. Mutations in tumor suppressor TP53 gene in formalin-fixed, paraffin embedded tissues of squamous cell carcinoma (SCC) of lung cancer.
-
Myers, G.J., Davidson, P.W., Cox, C., Shamlaye, C.F., Palumbo, D., Cernichiari, E., Sloane-Reeves, J., Wilding, G.E., Kost, J., Huang, L.-S., Clarkson, T.W., 2003. Prenatal methylmercury exposure from ocean fish consumption in the Seychelles child development study. The Lancet 361, 16861692. https://doi.org/10.1016/S0140-6736(03)13371-5
-
Clarkson, T.W., Magos, L. and Myers, G.J., 2003. The toxicology of mercurycurrent exposures and clinical manifestations. New England Journal of Medicine, 349(18), pp.1731-1737.
-
Järup, L., Rogenfelt, A., Elinder, C.G., Nogawa, K. and Kjellström, T., 1983. Biological half-time of cadmium in the blood of workers after cessation of exposure. Scandinavian journal of work, environment & health, pp.327-331.
-
Hu, H., Shih, R., Rothenberg, S., Schwartz, B.S., 2007. The Epidemiology of Lead Toxicity in Adults: Measuring Dose and Consideration of Other Methodologic Issues. Environmental Health Perspectives 115, 455462.
https://doi.org/10.1289/ehp.9783
-
Heath, L.M., Soole, K.L., McLaughlin, M.L., McEwan, G.T.A., Edwards, J.W., 2003. Toxicity of Environmental Lead and the Influence of Intestinal Absorption in Children. Reviews on
Environmental Health 18. https://doi.org/10.1515/REVEH.2003.18.4.231
-
Weiss, B., Clarkson, T.W., Simon, W., 2002. Silent latency periods in methylmercury poisoning and in neurodegenerative disease. Environmental Health Perspectives 110, 851854. https://doi.org/10.1289/ehp.02110s5851
-
Guzzi, G., La Porta, C.A.M., 2008. Molecular mechanisms triggered by mercury. Toxicology 244, 112. https://doi.org/10.1016/j.tox.2007.11.002
-
Lynes, M.A., Kang, Y.J., Sensi, S.L., Perdrizet, G.A., Hightower, L.E., 2007. Heavy Metal Ions in Normal Physiology, Toxic Stress, and Cytoprotection. Annals of the New York Academy of Sciences 1113, 159172. https://doi.org/10.1196/annals.1391.010
-
Klaassen, C.D., Liu, J., Diwan, B.A., 2009. Metallothionein protection of cadmium toxicity. Toxicology and Applied Pharmacology 238, 215220.
https://doi.org/10.1016/j.taap.2009.03.026
-
Mann, K.V., Picciotti, M.A., Spevack, T.A. and Durbin, D.R., 1989. Management of acute iron overdose. Clinical pharmacy, 8(6), pp.428-440.
-
Salnikow, K., Zhitkovich, A., 2008. Genetic and Epigenetic Mechanisms in Metal Carcinogenesis and Cocarcinogenesis: Nickel, Arsenic, and Chromium. Chem. Res. Toxicol. 21, 2844. https://doi.org/10.1021/tx700198a
-
Patterson, T.J., Ngo, M., Aronov, P.A., Reznikova, T.V., Green, P.G., Rice, R.H., 2003. Biological Activity of Inorganic Arsenic and Antimony Reflects Oxidation State in Cultured Human Keratinocytes. Chem. Res. Toxicol. 16, 16241631. https://doi.org/10.1021/tx034146y
-
Alkahtani, S., 2009. Cell Death Induced in 1\/Iice Somatic Cells by Arsenic Tr ioxide. Journal ofBiological Sciences, 9(7), pp.721-729.
-
Suzuki, K., 2004. Distributions and chemical forms of arsenic after intravenous administration of dimethylarsinic and monomethylarsonic acids to rats. Toxicology and Applied Pharmacology 198, 336344.
https://doi.org/10.1016/j.taap.2003.10.029
-
Jensen, T.J., Wozniak, R.J., Eblin, K.E., Wnek, S.M., Gandolfi, A.J., Futscher, B.W., 2009. Epigenetic mediated transcriptional activation of WNT5A participates in arsenical-associated malignant transformation. Toxicology and Applied Pharmacology 235, 3946. https://doi.org/10.1016/j.taap.2008.10.013
-
Galanis, A., Karapetsas, A., Sandaltzopoulos, R., 2009. Metal- induced carcinogenesis, oxidative stress and hypoxia signalling. Mutation Research/Genetic Toxicology and Environmental Mutagenesis 674, 3135.
https://doi.org/10.1016/j.mrgentox.2008.10.008
-
Melnikova, V.O., Ananthaswamy, H.N., 2005. Cellular and molecular events leading to the development of skin cancer. Mutation Research/Fundamental and Molecular Mechanisms of Mutagenesis 571, 91106.
https://doi.org/10.1016/j.mrfmmm.2004.11.015
-
Ravanat, J.-L., Douki, T., Cadet, J., 2001. Direct and indirect effects of UV radiation on DNA and its components. Journal of Photochemistry and Photobiology B: Biology 63, 88102.
https://doi.org/10.1016/S1011-1344(01)00206-8
-
Rossman, T.G., Uddin, A.N., Burns, F.J., Bosland, M.C., 2001. Arsenite Is a Cocarcinogen with Solar Ultraviolet Radiation for Mouse Skin: An Animal Model for Arsenic Carcinogenesis. Toxicology and Applied Pharmacology 176, 6471.
https://doi.org/10.1006/taap.2001.9277
-
Rossman, T.G., Uddin, A.N., Burns, F.J., Bosland, M.C., 2002. Arsenite cocarcinogenesis: an animal model derived from genetic toxicology studies. Environmental Health Perspectives 110, 749 752. https://doi.org/10.1289/ehp.02110s5749
-
Fujihara, J., Fujii, Y., Agusa, T., Kunito, T., Yasuda, T., Moritani, T., Takeshita, H., 2009. Ethnic differences in five intronic polymorphisms associated with arsenic metabolism within human arsenic (+3 oxidation state) methyltransferase (AS3MT) gene. Toxicology and Applied Pharmacology 234, 4146.
https://doi.org/10.1016/j.taap.2008.09.026
-
Vahter, M., 2000. Genetic polymorphism in the biotransformation of inorganic arsenic and its role in toxicity. Toxicology Letters 112113, 209217. https://doi.org/10.1016/S0378- 4274(99)00271-4
-
Pan, J., Plant, J.A., Voulvoulis, N., Oates, C.J., Ihlenfeld, C., 2010. Cadmium levels in Europe: implications for human health. Environ Geochem Health 32, 112.
https://doi.org/10.1007/s10653-009-9273-2
-
Järup, L., Åkesson, A., 2009. Current status of cadmium as an environmental health problem. Toxicology and Applied Pharmacology 238, 201208.
https://doi.org/10.1016/j.taap.2009.04.020
-
Ju-Kun, S., Yuan, D.-B., Rao, H.-F., Chen, T.-F., Luan, B.-S., Xu, X.-M., Jiang, F.-N., Zhong, W.-D., Zhu, J.-G., 2016. Association Between Cd Exposure and Risk of Prostate Cancer: A PRISMA-Compliant Systematic Review and Meta-Analysis. Medicine 95, e2708. https://doi.org/10.1097/MD.0000000000002708
-
Hu, W.-Y., Shi, G.-B., Hu, D.-P., Nelles, J.L., Prins, G.S., 2012. Actions of estrogens and endocrine disrupting chemicals on human prostate stem/progenitor cells and prostate cancer risk. Molecular and Cellular Endocrinology 354, 6373.
https://doi.org/10.1016/j.mce.2011.08.032
-
Polkowska, ., Kozowska, K., Namienik, J., Przyjazny, A., 2004. Biological Fluids as a Source of Information on the Exposure of Man to Environmental Chemical Agents. Critical Reviews in Analytical Chemistry 34, 105119.
https://doi.org/10.1080/10408340490475911
-
Przybylowicz, A., Chesy, P., Herman, M., Parczewski, A., Walas, S., Piekoszewski, W., 2012. Examination of distribution of trace elements in hair, fingernails and toenails as alternative biological materials. Application of chemometric methods. Open Chemistry 10, 15901599. https://doi.org/10.2478/s11532-012-0089-z
-
Tête, N., Afonso, E., Crini, N., Drouhot, S., Prudent, A.-S., Scheifler, R., 2014. Hair as a noninvasive tool for risk assessment: Do the concentrations of cadmium and lead in the hair of wood mice (Apodemus sylvaticus) reflect internal concentrations? Ecotoxicology and Environmental Safety 108, 233241. https://doi.org/10.1016/j.ecoenv.2014.07.010
-
Sawada, N., Iwasaki, M., Inoue, M., Takachi, R., Sasazuki, S., Yamaji, T., Shimazu, T., Endo, Y. and Tsugane, S., 2012. Long- term dietary cadmium intake and cancer incidence. Epidemiology, pp.368-376.
-
Joseph, P., 2009. Mechanisms of cadmium carcinogenesis. Toxicology and Applie Pharmacology 238, 272279.
https://doi.org/10.1016/j.taap.2009.01.011
-
Luevano, J., Damodaran, C., 2014. A Review of Molecular Events of Cadmium-Induced Carcinogenesis. J Environ Pathol Toxicol Oncol 33, 183194.
https://doi.org/10.1615/JEnvironPatholToxicolOncol.2014011075
-
Achanzar, W.E., Diwan, B.A., Liu, J., Quader, S.T., Webber, M.M., Waalkes, M.P., 2001 Cadmium-induced Malignant Transformation of Human Prostate Epithelial Cells 5.
-
Bosland, M.C., 2013. A perspective on the role of estrogen in hormone-induced prostate carcinogenesis. Cancer Letters 334, 2833. https://doi.org/10.1016/j.canlet.2012.08.027
-
Lau, K.-M., To, K.-F., 2016. Importance of Estrogenic Signaling and Its Mediated Receptors in Prostate Cancer. IJMS 17, 1434. https://doi.org/10.3390/ijms17091434
-
Yasunaga, Y., Xu, L.L., Moul, J.W., Peehl, D.M., Srivastava, S., Rhim, J.S., 2002. Cadmium-induced neoplastic transformation of human prostate epithelial cells. INTERNATIONAL JOURNAL OF ONCOLOGY 5.
-
Darbre, P.D., 2005. Aluminium, antiperspirants and breast cancer. Journal of Inorganic Biochemistry 99, 19121919. https://doi.org/10.1016/j.jinorgbio.2005.06.001
-
Satoh, E., Yasuda, I., Yamada, T., Suzuki, Y., Ohyashiki, T., 2007. Involvement of NO Generation in Aluminum-Induced Cell Death. Biological & Pharmaceutical Bulletin 30, 13901394. https://doi.org/10.1248/bpb.30.1390
-
Sun, X., Fontaine, J.-M., Bartl, I., Behnam, B., Welsh, M.J., Benndorf, R., 2007. Induction of Hsp22 (HspB8) by estrogen and the metalloestrogen cadmium in estrogen receptor positive breast cancer cells. Cell Stress Chaper 12, 307. https://doi.org/10.1379/CSC-276.1
-
Stellman, S.D., Djordjevic, M.V., Britton, J.A., Muscat, J.E., Citron, M.L., Kemeny, M., Busch, E. and Gong, L., 2000. Breast cancer risk in relation to adipose concentrations of organochlorine pesticides and polychlorinated biphenyls in Long Island, New
York. Cancer Epidemiology and Prevention Biomarkers, 9(11), pp.1241-1249.
-
Harley, C.B., 2008. Telomerase and cancer therapeutics. Nat Rev Cancer 8, 167179. https://doi.org/10.1038/nrc2275
-
Lukusa, T., Fryns, J.P., 2008. Human chromosome fragility. Biochimica et Biophysica Acta (BBA) – Gene Regulatory Mechanisms 1779, 316.
https://doi.org/10.1016/j.bbagrm.2007.10.005
-
Dunnick, J.K., Elwell, M.R., Radovsky, A.E., Benson, J.M., Hahn, F.F., Nikula, K.J., Barr, E.B. and Hobbs, C.H., 1995. Comparative carcinogenic effects of nickel subsulfide, nickel oxide, or nickel sulfate hexahydrate chronic exposures in the lung. Cancer research, 55(22), pp.5251-5256.
-
Nielsen, G.D., Søderberg, U., Jørgensen, P.J., Templeton, D.M., Rasmussen, S.N., Andersen, K.E., Grandjean, P., 1999. Absorption and Retention of Nickel from Drinking Water in Relation to Food Intake and Nickel Sensitivity. Toxicology and Applied Pharmacology 154, 6775.
https://doi.org/10.1006/taap.1998.8577
-
Ke, Q., Ellen, T., Costa, M., 2008. Nickel compounds induce histone ubiquitination by inhibiting histone deubiquitinating enzyme activity. Toxicology and Applied Pharmacology 228, 190199. https://doi.org/10.1016/j.taap.2007.12.015
-
Lee, Y.-W., Broday, L., Costa, M., 1998. Effects of nickel on DNA methyltransferase activity and genomic DNA methylation levels. Mutation Research/Genetic Toxicology and Environmental Mutagenesis 415, 213218. https://doi.org/10.1016/S1383- 5718(98)00078-3
-
Zhitkovich, A., Voitkun, V., Costa, M., 1995. Glutathione and free amino acids form stable complexes with DNA following exposure of intact mammalian cells to chromate. Carcinogenesis 16, 907913. https://doi.org/10.1093/carcin/16.4.907
-
Schnekenburger, M., Peng, L., Puga, A., 2007. HDAC1 bound to the Cyp1a1 promoter blocks histone acetylation associated with Ah receptor-mediated trans-activation. Biochimica et Biophysica Acta (BBA) – Gene Structure and Expression 1769, 569578. https://doi.org/10.1016/j.bbaexp.2007.07.002
-
Wu, J.-P., Chang, L.W., Yao, H.-T., Chang, H., Tsai, H.-T., Tsai, M.-H., Yeh, T.-K., Lin, P., 2009. Involvement of Oxidative Stress and Activation of Aryl Hydrocarbon Receptor in Elevation of CYP1A1 Expression and Activity in Lung Cells and Tissues by Arsenic: An In Vitro and In Vivo Study. Toxicological Sciences 107, 385393. https://doi.org/10.1093/toxsci/kfn239
-
Nebert, D.W., Roe, A.L., Dieter, M.Z., Solis, W.A., Yang, Y., Dalton, T.P., 2000. Role of the aromatic hydrocarbon receptor and [Ah] gene battery in the oxidative stress response, cell cycle control, and apoptosis. Biochemical Pharmacology 59, 6585. https://doi.org/10.1016/S0006-2952(99)00310-X
-
Li, R., Shugart, Y.Y., Zhou, W., An, Y., Yang, Y., Zhou, Y., Zhang, B., Lu, D., Wang, H., Qian, J., Jin, L., 2009. Common genetic variations of the cytochrome P450 1A1 gene and risk of hepatocellular carcinoma in a Chinese population. European Journal of Cancer 45, 12391247.
https://doi.org/10.1016/j.ejca.2008.11.007
-
Ha, L., Ceryak, S., Patierno, S.R., 2003. Chromium (VI) Activates Ataxia Telangiectasia Mutated (ATM) Protein. Journal of Biological Chemistry 278, 1788517894. https://doi.org/10.1074/jbc.M210560200
-
Blankenship, L.J., Manning, F.C., Orenstein, J.M. and Patierno, S.R., 1994. Apoptosis is the mode of cell death caused by carcinogenic chromium. Toxicology and applied pharmacology, 126(1), pp.75-83.
-
Gao, N., Jiang, B.-H., Leonard, S.S., Corum, L., Zhang, Z., Roberts, J.R., Antonini, J., Zheng, J.Z., Flynn, D.C., Castranova, V., Shi, X., 2002. p38 Signaling-mediated Hypoxia-inducible Factor 1 and Vascular Endothelial Growth Factor Induction by Cr(VI) in DU145 Human Prostate Carcinoma Cells. Journal of Biological Chemistry 277, 4504145048. https://doi.org/10.1074/jbc.M202775200
-
Thomas, G., 1992. MAP kinase by any other name smells just as sweet. Cell 68, 36. https://doi.org/10.1016/0092-8674(92)90199- M
-
Xiang, N., Zhao, R., Song, G., Zhong, W., 2008. Selenite reactivates silenced genes by modifying DNA methylation and histones in prostate cancer cells. Carcinogenesis 29, 21752181. https://doi.org/10.1093/carcin/bgn179
-
Zou, Y., Niu, P., Yang, J., Yuan, J., Wu, T., Chen, X., 2008. The JNK signaling pathway is involved in sodium-selenite-induced apoptosis mediated by reactive oxygen in HepG2 cells. Cancer Biology & Therapy 7, 689696.
https://doi.org/10.4161/cbt.7.5.5688
-
Chen, T., Wong, Y.-S., 2009. Selenocystine induces caspase- independent apoptosis in MCF-7 human breast carcinoma cells with involvement of p53 phosphorylation and reactive oxygen species generation. The International Journal of Biochemistry & Cell Biology 41, 666676.
https://doi.org/10.1016/j.biocel.2008.07.014
-
Hurst, R., Elliott, R.M., Goldson, A.J., Fairweather-Tait, S.J., 2008. Se-methylselenocysteine alters collagen gene and protein expression in human prostate cells. Cancer Letters 269, 117126. https://doi.org/10.1016/j.canlet.2008.04.025
-
Gibson, R.S., Hess, S.Y., Hotz, C., Brown, K.H., 2008. Indicators of zinc status at the population level: a review of the evidence. Br J Nutr 99, S14S23. https://doi.org/10.1017/S0007114508006818
-
Gallus, S., Foschi, R., Negri, E., Talamini, R., Franceschi, S., Montella, M., Ramazzotti, V., Tavani, A., Dal Maso, L., La Vecchia, C., 2007. Dietary Zinc and Prostate Cancer Risk: A Case-Control Study from Italy. European Urology 52, 1052 1057. https://doi.org/10.1016/j.eururo.2007.01.094
-
Gumulec, J., Masarik, M., Adam, V., Eckschlager, T., Provaznik, I., & Kizek, R. (2014). Serum and Tissue Zinc in Epithelial Mlignancies: A Meta-Analysis. PLoS ONE, 9(6), e99790. https://doi.org/10.1371/journal.pone.0099790
-
Prasad, A.S., 1991. Discovery of human zinc deficiency and studies in an experimental human model. The American journal of clinical nutrition, 53(2), pp.403-412.
-
Vallee, B.L., 1959. Biochemistry, Physiology and Pathology of Zinc. Physiological Reviews 39, 443490.
https://doi.org/10.1152/physrev.1959.39.3.443
-
Wu, X., Tang, J., Xie, M., 2015. Serum and hair zinc levels in breast cancer: a meta-analysis. Sci Rep 5, 12249. https://doi.org/10.1038/srep12249
-
Rink, L., 2000. Zinc and the immune system. Proc. Nutr. Soc. 59, 541552. https://doi.org/10.1017/S0029665100000781
-
Magneson, G.R., Puvathingal, J.M. and Ray Jr, W.J., 1987. The concentrations of free Mg2+ and free Zn2+ in equine blood plasma. Journal of Biological Chemistry, 262(23), pp.11140- 11148.
-
Costello, L.C., Fenselau, C.C., Franklin, R.B., 2011. Evidence for operation of the direct zinc ligand exchange mechanism for trafficking, transport, and reactivity of zinc in mammalian cells. Journal of Inorganic Biochemistry 105, 589599.
https://doi.org/10.1016/j.jinorgbio.2011.02.002
-
Franklin, R.B., Ma, J., Zou, J., Guan, Z., Kukoyi, B.I., Feng, P., Costello, L.C., 2003. Human ZIP1 is a major zinc uptake transporter for the accumulation of zinc in prostate cells. Journal of Inorganic Biochemistry 96, 435442.
https://doi.org/10.1016/S0162-0134(03)00249-6
-
Zou, J., Milon, B.C., Desouki, M.M., Costello, L.C., Franklin, R.B., 2011. hZIP1 zinc transporter down-regulation in prostate cancer involves the overexpression of ras responsive element binding protein-1 (RREB-1): RREB-1 Represses hZIP1 Expression in Prostate. Prostate 71, 15181524.
https://doi.org/10.1002/pros.21368
-
Anirban Goutam Mukherjee, Uddesh Ramesh Wanjari, 2020. A Review on the Present and Future Aspects of Various Prokaryotic Pigments and Metabolites Demonstrating Anti-Cancerous Properties. IJERT V9, IJERTV9IS070578. https://doi.org/10.17577/IJERTV9IS070578
-
Shiina, H., Igawa, M., Ishibe, T., 1996. Estramustine-binding protein to dihydrotestosterone ratio in human prostatic carcinoma: a new marker for predicting disease progression. British Journal of Urology 77, 96101. https://doi.org/10.1046/j.1464- 410X.1996.81516.x
-
Järup, L., 2003. Hazards of heavy metal contamination. British Medical Bulletin 68, 167182.
https://doi.org/10.1093/bmb/ldg032
-
Cartwright, G.E., Markowitz, H., Shields, G.S., Wintrobe, M.M., 1960. Studies on copper metabolism XXIX. The American Journal of Medicine 28, 555563. https://doi.org/10.1016/0002- 9343(60)90150-9
-
Karimi, G., Shahar, S., Homayouni, N., Rajikan, R., Bakar, N.F.A., Othman, M.S., 2012. Association between Trace Element and Heavy Metal Levels in Hair and Nail with Prostate Cancer. Asian Pacific Journal of Cancer Prevention 13, 42494253. https://doi.org/10.7314/APJCP.2012.13.9.4249
-
Ozmen, H., Erulas, F.A., Karatas, F., Cukurovali, A., Yalcin, O., 2006. Comparison of the concentration of trace metals (Ni, Zn, Co, Cu and Se), Fe, vitamins A, C and E, and lipid peroxidation in patients with prostate cancer. Clinical Chemistry and Laboratory Medicine (CCLM) 44. https://doi.org/10.1515/CCLM.2006.032
-
Tan, C., Chen, H., 2011. Screening of Prostate Cancer by Analyzing Trace Elements in Hair and Chemometrics. Biol Trace Elem Res 144, 97108. https://doi.org/10.1007/s12011-011-9038- 5
-
Safi, R., Nelson, E.R., Chitneni, S.K., Franz, K.J., George, D.J., Zalutsky, M.R., McDonnell, D.P., 2014. Copper Signaling Axis as a Target for Prostate Cancer Therapeutics. Cancer Res 74, 58195831. https://doi.org/10.1158/0008-5472.CAN-13-3527
-
Cater, M.A., Haupt, Y., 2011. Clioquinol induces cytoplasmic clearance of the X-linked inhibitor of apoptosis protein (XIAP): therapeutic indication for prostate cancer. Biochemical Journal 436, 481491. https://doi.org/10.1042/BJ20110123
-
Chen, D., Cui, Q.C., Yang, H., Barrea, R.A., Sarkar, F.H., Sheng, S., Yan, B., Reddy, G.P.V., Dou, Q.P., 2007. Clioquinol, a Therapeutic Agent for Alzheimers Disease, Has Proteasome- Inhibitory, Androgen ReceptorSuppressing, Apoptosis-Inducing, and Antitumor Activities in Human Prostate Cancer Cells and Xenografts. Cancer Res 67, 16361644. https://doi.org/10.1158/0008-5472.CAN-06-3546
-
Benbrahim-Tallaa, L., Waterland, R.A., Dill, A.L., Webber, M.M., Waalkes, M.P., 2007b. Tumor Suppressor Gene Inactivation during Cadmium-Induced Malignant Transformation of Human Prostate Cells Correlates with Overexpression of de Novo DNA Methyltransferase. Environ Health Perspect 115, 14541459. https://doi.org/10.1289/ehp.10207
-
Benbrahim-Tallaa, L., Webber, M.M., Waalkes, M.P., 2007c. Mechanisms of Acquired Androgen Independence during Arsenic-Induced Malignant Transformation of Human Prostate Epithelial Cells. Environmental Health Perspectives 115, 243 247. https://doi.org/10.1289/ehp.9630
-
Cai, H., Wu, J. -s., Muzik, O., Hsieh, J.-T., Lee, R.J., Peng, F., 2014. Reduced 64Cu Uptake and Tumor Growth Inhibition by Knockdown of Human Copper Transporter 1 in Xenograft Mouse Model of Prostate Cancer. Journal of Nuclear Medicine 55, 622 628. https://doi.org/10.2967/jnumed.113.126979
-
Muzandu, K., Shaban, Z., Ishizuka, M., Kazusaka, A., Fujita, S., 2005. Nitric oxide enhances catechol estrogen-induced oxidative stress in LNCaP cells. Free Radical Research 39, 389398. https://doi.org/10.1080/10715760400029710
-
Mayne, S.T., Playdon, M.C., Rock, C.L., 2016. Diet, nutrition, and cancer: past, present and future. Nat Rev Clin Oncol 13, 504
515. https://doi.org/10.1038/nrclinonc.2016.24
-
Mukherjee, A.G., Wanjari, U.R., Balgote, P.J., n.d. A REVIEW ON THE USEFULNESS OF VARIOUS EUKARYOTIC PIGMENTS AND METABOLITES IN CANCER TREATMENT. World Journal of Pharmaceutical Research 9, 26.
-
Xue, D., Zhou, C.-X., Shi, Y.-B., Lu, H., He, X.-Z., 2015.
Decreased expression of ferroportin in prostate cancer. Oncology Letters 10, 913916. https://doi.org/10.3892/ol.2015.3363
-
Andersen, O., 1999. Principles and Recent Developments in Chelation Treatment of Metal Intoxication. Chem. Rev. 99, 2683 2710. https://doi.org/10.1021/cr980453a
-
Powell, J., 1999. Urinary excretion of essential metals following intravenous calcium disodium edetate: an estimate of free zinc and zinc status in man. Journal of Inorganic Biochemistry 75, 159165. https://doi.org/10.1016/S0162-0134(99)00054-9
-
Danielli, J.F., Danielli, M., Fraser, J.B., Mitchell, P.D., Owen, L.N., Shaw, G., 1947. BAL-INTRAV: a new non-toxic thiol for intravenous injection in arsenical poisoning*. Biochemical Journal 41, 325333. https://doi.org/10.1042/bj0410325
-
Mückter, H., Lieb, B., Reich, F.-X., Hunder, G., Walther, U., Fichtl, B., 1997. Are we ready to replace dimercaprol (BAL) as an arsenic antidote? Hum Exp Toxicol 16, 460465. https://doi.org/10.1177/096032719701600807
-
Cheney, K., Gumbiner, C., Benson, B. and Tenenbein, M., 1995. Survival after a severe iron poisoning treated with intermittent infusions of deferoxamine. Journal of Toxicology: Clinical Toxicology, 33(1), pp.61-66.
-
Aposhian, H.V., Maiorino, R.M., Gonzalez-Ramirez, D., Zuniga- Charles, M., Xu, Z., Hurlbut, K.M., Junco-Munoz, P., Dart, R.C., Aposhian, Mary.M., 1995. Mobilization of heavy metals by newer, therapeutically useful chelating agents. Toxicology 97, 2338. https://doi.org/10.1016/0300-483X(95)02965-B
-
Chasapis, C.T., Loutsidou, A.C., Spiliopoulou, C.A., Stefanidou, M.E., 2012. Zinc and human health: an update. Arch Toxicol 86, 521534. https://doi.org/10.1007/s00204-011-0775-1
-
Abubakar, 2010. Comparative transcriptional study of the effects of high intracellular zinc on prostate carcinoma cells. Oncol Rep23. https://doi.org/10.3892/or_00000789