
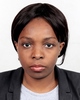
- Open Access
- Authors : Kenu E. Sarah , Uhunmwangho Roland , Okafor Ephraim N. C.
- Paper ID : IJERTV9IS070244
- Volume & Issue : Volume 09, Issue 07 (July 2020)
- Published (First Online): 18-07-2020
- ISSN (Online) : 2278-0181
- Publisher Name : IJERT
- License:
This work is licensed under a Creative Commons Attribution 4.0 International License
A Review of Solar Photovoltaic Technologies
Kenu E. Sarap, Prof. Uhunmwangho Roland1, Prof. Okafor Ephraim N. C2
-
University of Port Harcourt, Rivers State, Nigeria
-
Federal University of Technology, Owerri, Imo State.
Abstract:- Energy from the sun has sustained mans activities for thousands of years. It can be harnessed directly as light energy to generate electricity from Photovoltaic (PV) Cells, or as thermal energy for heating, cooking, drying and electricity generation from thermal systems. However, using PV cells has been the preferred method of solar electricity generation for several decades now. Selecting the appropriate PV cell for a particular region requires a proper understanding of the basic mechanisms and functions of various PV technologies that are commonly used. In this paper, we have reviewed the progressive development of solar PV technologies from the first generation to present day configurations. Discussion is also made on the various Solar PV production data across different regions, including relevant recommendations for improvements.
Keywords: Solar PV, Technologies, Monocrystalline, Multi-Si, Thin film, CIGS, CdTe, Nanotechnology
-
INTRODUCTION
The rise in electrical energy demand worldwide has led to the massive reliance on power generators which supply power to consumers. However, the negative environmental impact of burning fossil fuels to produce electricity has triggered the need for a reduction in greenhouse gas emissions (GHG) [1]. The outcome has been a shift towards cleaner forms of renewable energy generation like solar and wind technologies. Solar PV technology is a common renewable energy option which has the capacity to provide cleaner, stable, expandable and cost-effective electricity for years to come [2]. This has driven governments in different continents to build and implement solar PV technology. Production policies comprises production subsidies, technology transfer, publicly funded research and development (R&D), research implementation, and industry research association. On the other hand, government consumption policies comprise consumption subsidies, renewable portfolio standards, tax credits, and concessionary financing [3]. Different types of PV materials can be obtained globally. Some hundreds of manufacturers have partaken in building modules possessing diverse efficiencies and inherent shortcomings. Similarly, the cost of installing these systems depends on the module characteristics and the project at hand. This paper aims to review the old and recently published information about solar PVs in terms of materials and module efficiency, as well as the global PV application status.
-
SOLAR PV TECHNOLOGIES
A solar cell is a device with the primary function of transforming light energy directly into electricity through photovoltaic effect [4]. Its electrical characteristics which includes current, voltage, or resistance, differs with exposure to light energy from any source, whether natural or artificial. Solar cells form photovoltaic modules. The have a number
of applications. They are used in the Solar PV industry as the building blocks of solar modules; as photo detectors for automatic switching of streetlights and other light-sensitive devices (for example infrared detectors), and as light sensors for scientific research.
-
Wafer-based Solar Technology (First generation) Solar cells are either composed of one layer of light- absorbing substance (single-junction) or utilizes several physical setups (multi-junctions) so as to apply different absorption and charge separation mechanisms [5]. The first generation wafer-based cells comprises of crystalline silicon, example of which are polysilicon and monocrystalline silicon.
The upper surface of the cell is built with a thin layer of the p-type material to allow free light absorption (figure 1). The metal rings are positioned around p-type and n-type material serving as the positive and negative output terminals respectively. The semiconductor material forms the single unit of the PV cell [6].
Fig 1. Wafer-based Solar Technology (Circuit Globe (n.d.)) [7]
-
Monocrystalline Solar Cell
Mono crystalline solar cell (figure 2), are made using a single crystal of silicon (Si) by the Czochralski process [8]. The production of the Si crystals is usually done with high accuracy, from large ingots. Monocrystalline cells have been found to measure 16% in efficiency [8]. However, a higher efficiency of 19.8% has been achieved from an enhanced multicrystalline silicon solar cell, as well as a rise 24.4% for monocrystalline cells [7]. This multicrystalline cells enhancement emanated from covering multicrystalline surfaces with oxides formed thermally so as to limit their detrimental electronic processes and from isotropic etching, producing a hexagonally symmetric honeycomb surface texture
Fig 2. Monocrystalline Solar Panel (Sendy (2017)) [10]
Dobrzanski et al [11] presented a traditional technological activity through screen printed process of monocrystalline silicon solar cells manufacture. Schottky and Zener diodes provided protection for the device while the cells were linked to the PV modules to produce electricity [12]. The module found practical application in traffic lighting for pedestrian crossing.
-
Polycrystalline Silicon Solar Cell (Poly-Si or Mc- Si)
Polycrystalline PV modules (figure 3) are formed from various crystals, joined together in a unit cell [13]. The forming procedure is cost effective, involving cooling a graphite mould composed of molten silicon. When it hardens, it results in crystal structures of different sizes on whose border defects occur. These defects reduce the degree of efficiency with laboratory efficiency of 18% to 23%, and production range of 14% to 17% [14]. Polycrystalline Si solar cells represent the most common amongst others, making up 48% of the total produced in 2008 [15].
Fig 3. Polycrystalline Solar Panel (Indiamart (n.d.)) [16]
Zsiboracs et al. [17] discussed methods of cooling monocrystalline and polycrystalline solar modules with water vaporizing regarding the effects of temperature on performance and its economic relations. Results showed a connection between temperature efficiency changes of monocrystalline and polycrystalline solar modules (0.5%/1
°C). Effective application of solar module cooling systems was 1015% more expensive than the cost of systems without cooling. Khalis et al [18] concluded that the maximum power and efficiency of polycrystalline cells
decreased with cell temperature and the temperature coefficient of the efficiency, while the maximum output power was found to be negative.
-
-
Thin Film Technology
These represent the Second generation cells and are amorphous silicon, Cadmium Telluride (CdTe) and Copper Indium Gallium di-Selenide (CIGS) cells. They are commercially found and applied extensively in utility-scale photovoltaic power stations, as they have better performance under cloudy conditions [19].
-
Amorphous Silicon Solar Cell (A-Si)
This type of solar cell was the most commonly known amongst thin films, though it has been on the market for over 15 years [20]. It has been commonly utilized in pocket and desktop calculators, and more recently in solar panels for domestic and utility generation of electricity. Amorphous-Si modules are produced by placing a tiny film of silicon vapour (approximately 1 µm thick) on a substrate material like glass or metal. A transparent conducting oxde (TCO) is incorporated in the set up to help reduce the series resistance by providing a lateral conducting pathway for current which is illustrated in figure 4 [21]. The lateral distance that carriers have to travel in the doped ZnO can be fairly large; this is to allow a sufficient area to receive sunlight; therefore the resistivity of the doped ZnO must be low. If there was no TCO, but only metal fingers, a significant amount of current would be lost due to the series resistance resulting in a heavy efficiency loss. While crystalline silicon is about 18% efficient, amorphous solar cells yield stays in the 8.1% region [22]. This poor efficiency stems from the Staebler- Wronski effect, occurring in the first hours after the panels begins receiving sunlight. The principal edge amorphous silicon solar cells have over others lie in the much reduced cost of production.
Fig 4. Amorphous Silicon Solar Cell Composition (Yang T.C. (2016) [21]
Kabir et al. [23] discussed the gradual advancement of amorphous silicon (a-Si) and microcrystalline (c-Si:H) silicon-based cells. The efficiency of hydrogenated amorphous silicon (a-Si:H) thin film solar cells saw an enhancement from 2.4% to 15.2% (steady 13.0%). It was also noted that a-Si:H solar cells had multijunction device function, bandgap allocation, ease of production, scalable and huge optical absorption coefficient.
-
Cadmium Telluride (CdTe) Thin Film Solar Cell Cadmium telluride (CdTe) photovoltaics is formed from cadmium telluride, a thin semiconductor layer built to design to capture and convert sunlight to electricity (figure 5) [24]. Cadmium telluride PV is the sole thin film technology having less costs than traditional solar cells produced with crystalline silicon in multi-kilowatt [25]. Basol and McCandless [26] found that most outstanding enhancement of CdTe efficiencies occurred from 2013 to 2014 when small-area cell conversion efficiency improved to 20% range and a champion module efficiency of 17% was recorded [27]. Other noteworthy advantages of this technology are lower life-cycle greenhouse gas and heavy metal emissions, less carbon footprint, and short energy payback times.
Kato et al [28] did a lifecycle analysis on CdTe photovoltaic modules in order to determine the energy payback time (EPT) along with the life-cycle CO2 emissions of a residential rooftop PV system. It was noted that the major energy needed for the manufacture 1 m2 of the CdS/CdTe PV module was almost identical to a-Si PV module at annual production scale of 100 MW. EPT was evaluated at 1.7 year, much less than the lifetime of the PV system but resembling that of a-Si PV modules. The life-cycle CO2 emissions from finding was calculated to be 149 g-C/kWh, lower than utility-generated electrical power.
Fig 5. CdTe Thin Film Solar Cell Layers. (Dharmada et al. (2014)) [29]
-
Copper Indium Gallium Di-Selenide (CIGS) Solar Cells
CIGS is a direct band gap and quaternary compound semiconductor composed of: Copper, Indium, Gallium and Selenium (figure 6) [30]. The right side of figure 5 shows a transverse section through a scanning electron microscope of a real device. CIGS possesses a higher efficiency than CdTe (approximately 10% – 12% on average), with some achieving an efficiency of 22.8%, thus competing favourably with crystalline silicon (c-Si) wafer based solar cells [31].
Devi et al [32] designed and analysed the Cu (In,Ga) Se2 (CIGS) solar cell using Solar Cell Capacitance Simulator in One Dimension (SCAPS-1D) simulation software. They replaced the traditional model with a less complex one to achieve a simple fabrication requiring less cost and materials. This CIGS model thus became ITO/CIGS/OVC/CdS/Metal contact, with OVC being the ordered vacancy compound. The results obtained from this modified structure (even with low light) surpassed that from the more complex one.
Figure 6. CIGS Solar Cell Composition (Powalla et al. (2017)) [33]
-
-
Third Generation Solar Technologies
The newly arising thin film technologies are grouped under third generation solar technologies. Most of them are not yet commercially available but under R&D phases.
-
Nano Crystal Based Solar Cells
Nanocrystal based solar cells are commonly termed Quantum dots (QD) solar cells [34]. They include a semiconductor, popularly from transition metal groups in the size of nanocrystal range composed of semiconducting materials, and can achieve efficiencies reaching 16.6%. Typically, QDs have a structure consisting of a core on which layers of different compounds are deposited with the aim to improve efficiency by reducing interaction forces between the exciton and the surface of the nanoparticle.
Gur [35] presented worked on enhancing incorporation and stability in nanocrystal-based solar cells by means of progress made in material engineering and device structure. The establishment of 3-dimensional nanocrystals illustrated the capacity for enhancing movement and flow in hybrid solar cells and permitted novel fabrication methods for optimizing introduction in these systems. The findings showed proved that donor-acceptor charge transfer and directed carrier diffusion can be implemented in a configuration without organic parts, and that nanocrystals may function as foundation for efficient, reliable, and cheaper thin film solar cells.
Fig 7. Nano Crystal Based Solar Cells (Anthony (2011)) [36]
-
Polymer Solar Cells (PSC)
A PSC is built with serially linked thin functional layers lined atop a polymer foil. It operates basically as a mixture of donor (polymer) and an acceptor. Different substances are
applied to draw sunlight energy, comprising organic components like a conjugate/conducting polymer [37].
A typical organic bulk heterojunction device is shown in figure 8 and consists of layers of different materials, such as a transparent bottom electrode of indiumtin oxide (ITO), a hole transport layer (HTL) like, the donor polymer/accetor molecule blend as the active layer and a top electrode layer (usually metallic).
In a research by Abdurazzaq et al [38], polymeric nano- composites incorporating graphitic nanostructures were extensively investigated for the next generation of efficient and low-cost solar cells. The nano materials showed excellent electrical and mechanical properties, excellent carrier transport capabilities, and provided an efficient pathway to the dissociated charge carriers.
Luceno-Sanchez et al [39] did a review on the recent PV cell materials utilized for production and how they impacted on the efficiency and overall cost of manufacture. It was envisaged that upcoming fourth generation (4GEN) PSCs integrating carbon-based nano-materials would provide greater operation levels to compete with those of normal silicon-based cells. Polymer solar cells have an efficiency of 6.5%, and finds applications in diverse ways due to their lightweight and flexibility, such as solar windows, solar curtains, and so on.
Fig 8. Polymer Solar Cells Layers. (Gusain et al. (2019)) [40]
-
Dye Sensitized Solar Cells (DSSC)
In DSSC-based solar cells (figure 9), the various electrodes are separated by dye molecules [41]. The four elements that make up DSSCs are: semiconductor electrode (n-type TiO2 and p-type NiO), a dye sensitizer, redox mediator, and a counter electrode. When organic dyes are used, they usually are called organic solar cells. The DSSCs are attractive for its simplicity in production, flexibility and costing less to produce [42]. Because they can be used to create solar panels in different attractive colours, they are preferred for use in energy-efficient architectural applications like solar windows and glass walls of buildings.
Fig 9. Dye Sensitized Solar Panel Ccomposition (Wikipedia (2020)) [43]
The novelty of the DSSC solar cells results from the photosensitization of nano grained TiO2 coatings and the visible optically active dyes, boosting the efficiencyy to upwards of 10%[44]. Newer DSSCs are made up of a porous layer of titanium dioxide nanoparticles enveloped by a molecular dye which absorbs sunlight. The titanium dioxide is placed below a solution of electrolyte, with a platinum- based catalyst placed atop. Similar to standard alkaline batteries, an anode (the titanium dioxide) and a cathode (the platinum) are positioned on either side of a liquid conductor (the electrolyte). Sunlight penetrates the transparent electrode into the dye layer and excite the electrons which eventually moves into the titanium dioxide. This electron flow is harnessed to provide load power. When the movement in the external circuit is complete, they are re- incorporated into the cell via a metal electrode on the rear, going into the electrolyte. The electrolyte subsequently conveys the electrons back to the dye molecules.
-
Perovskite Solar Cells (PSCs)
-
Perovskite materials have become popular due to the cubic lattice-nested octahedral layered structures and the unique optical, thermal, and electromagnetic properties [45]. Those utilized in solar cells are a blend of organic-inorganic metal halide compound having the perovskite structure. The functions of perovskite materials has not been restricted to it being a light-absorbing layer, but it additionally operates as electron/hole transport layer because of its high extinction coefficient, huge charge mobility, extensive carrier lifetime, and wide carrier diffusion distance. Their photoelectric power conversion efficiency grew from 3.8% in 2009 to 20.9% in 2019, placing them in line to substitute conventional Si solar cells in coming years. PSCs are built based on two typical structures namely mesoporous and planar, unique to perovskite crystals. Figure 10((a) and (b)) gives a depiction of both types. The former contains a mesoporoustype metal oxide layer (TiO2 or Al2O3) enveloped using perovskite sensitizer. The latter comprises a perovskite film surrounded by electron and hole transporting layers.
Zhou et al. [46] obtained higher electron movement through the use of yttrium-doped TiO2 as the electron transport material and upgraded the ITO to limit the task of inserting the electrons to the ITO electrode from TiO2. The open-
circuit voltage and short-circuit current of the device saw a significant enhancement with the PCE reaching 19.3%.
The short circuit current is also obtained by taking VL to be zero. The current is thus:
Fig 10a. Mesoporous Structure PSCs [46]
This is basically because the series resistance Rse value is significantly small.
-
PRODUCTION AND PERFORMANCE OF SOLAR PV TECHNOLOGIES:
-
Performance and Efficiency of Solar PV Modules Solar cell performance is dependent on the Fill Factor (FF) and Conversion Efficiency [48]. The value of FF sits within 0 and 1. Mathematically, FF is defined as a ratio of the product of the maximum current value (Im) and voltage (Vm) to that of the product of short circuit current and open circuit voltage.
=
×
×
The conversion efficiency is:
=
Fig 10b. Planar Heterojunction Structure PSCs [46]
3. MATHEMATICAL MODEL OF SOLAR PHOTOVOLTAICS
The solar voltage and current characteristics exhibit an exponential behaviour [47]. The ideal equivalent circuit of a solar cell comprises of a current source placed parallel to a diode (figure 11). The practical equivalent model of solar cell has dual internal resistances (series and shunt).
Fig 11. Ideal (left) and Equivalent (right) Circuits of a Solar Cell
The series resistance Rse emanates from movement of electrons between the composite material and metal contact. The shunt resistance RSh is formed when electrons and hole pairs recombine just prior to supplying the load, thus impacting the load current. VL stands for the voltage across external load and IL is the load current. Whenever sunlight hits the solar cell top, the electrons in n side region are excited. The migration of electrons from n region to p region produces the photo current IPh. The current equation of solar cells is determined from Kirchhoffs Current Law as:
=
Diode current (ID) and voltage VD is defined by:
= ( 1)
= +
where q is the charge of electron, k is the Boltzmann constant and T is the temperature in oK.
For the open circuit voltage VOC, the load current IL is zero. Thus it is given as:
Data obtained from the solar efficiency tables showed that the efficiency of multi-junction cells and crystalline modules surpasses that of thin film and some newer solar modules (figures 12 and 13). Figure 10 shows that monocrystalline solar cell efficiency is greater than that of multicrystalline by about 4.3%, while CIGS is greater than CdTe by about 2.4%.
Figure 12. Solar PV Efficiencies by Technology (Graph: Fraunhofer ISE 2019)
Figure 13. Solar PV Efficiencies. (Graph: Fraunhofer ISE 2019)
=
(
+ 1)
Table 1 highlights the key characteristics and efficiency levels of the various solar photovoltaic technologies, as well as the pros and cons of implementing each.
-
Solar PV Production and Installation
By the end of 2018, China had the highest installed solar PV capacity, followed by Europe and North America (figure 14).
Figure 14. Global Cumulative PV Installation by Region (Data: IRENA. Graph: PSE GmbH 2019)
Table 1. Summary Highlights of Solar PV Technologies [49]-[57]
Solar Technology
Characteristics
Efficiency in 2019 (%)
Advantages
Drawbacks
Monocrystalline
Made from pure monocrystalline silicon that has a single, continuous, crystal lattice structure with little or no impurities.
26.7
Polycrystalline
Produced using numerous grains of monocrystalline silicon
22.3
CIGS
Uses semiconductor layers of CIGS to absorb sunlight and convert to electricity
23.4
1. Less efficient than monocrystalline panels
CdTe
Fabricated using a superstrate consisting of the successive layers of sodalime glass/ITO/CdS/CdTe/ back contact
21.0
Solar Technology
Characteristics
Efficiency in 2019 (%)
Advantages
Drawbacks
Amorphous Silicon
Composed of silicon atoms in a thin homogenous layer
13.4
1. Low efficiency
DSSC
Comprises of the electrode film layer, conductive transparent conductive oxide, counter electrode layer and the redox electrolyte layer
11.1
PSC
Utilizes ABX3 crystal structure known as perovskite structure as an active light harvesting layer
20.9
Polymer
Consists of a layered structure of a transparent front electrode, an active layer and a back electrode printed onto a plastic substrate
3-8
-
Longevity
-
low installation cost
-
Non hazardous
-
High cost
-
Fragility
-
Silicon is wasted in the Czochralski process
-
Simple production process
-
More heat tolerant than silicon based panels
-
Lower conversion efficiency than monocrystalline
-
Lower space efficiency
-
Uses little or no toxic material unlike CdTe
-
Have better resilience to heat than Si-based panels
-
Aborbs sunlight at shorter wavelength
-
Cost less to manufacture due to abundance of Cadmium material
-
Cadmium is very toxic
-
Disposal of old CdTe panels is a concern
-
Less efficient than C-Si
-
Multijunction device capacity
-
Easy fabrication
-
High optical absorption coefficient
-
Less toxic material than CIGS and CdTe
-
Flexible and less susceptible to cracks
-
Requires less cost
-
Functions in dim light and at wider angles
-
Long life and mechanical robustness
-
Electrolyte liquid comprises volatile organic solvents
-
Cannot be applied where large scale cases requiring higher cost and efficiency is a priority
-
Cost effective fabrication
-
Cheap to scale up
-
Less space required for installation
-
High efficiency
-
Materials breakdown fast from exposure to heat, moisture, snow.
-
Material is toxic in nature
-
Light weight and flexible, thus easy to size and shape
-
Ease of storage and transportation
-
Organic in nature, hence are environmentally friendly.
-
Very low efficiency
-
Faster degradation in outdoor situations.
The chart in figure 15 shows the percentage of global production of solar modules over a 20-year period. Europe, Japan and the US recorded a noticeable decline in production volume, while China invested massively in the production of solar modules with an outstanding increase in volume. Data
from the rest of the world indicates that there was a gradual increase in production volumes from the year 2004.
Figure 15. Global Cumulative PV Installation by Region (Data: Up to 2009: Navigant Consulting; since 2010: IHS. Graph: PSE GmbH 2018)
Figure 16 indicates that in the last seven years, China has been consistent in increasing solar PV production towards her goal of reducing annual carbon footprint and environmental pollution. This has been adopted in other Asia-Pacific and Central Asian regions too as shown in the chart.
During this period, India has reported annual increase in solar PV modules production, unlike the Middle East and African regions.
Figure 16. PV Module Production by Region (Graph: PSE GmbH 2019)
-
Recommendations
Multicrystalline efficiency has been improving largely because of the availability of silicon and its simple production procedure. The efficiency got to 20.4% and now at 22.3%, currently holding 80% of the PV market [47]. However, for PV to establish a major impact in the power sector the efficiency of other types of materials has to be boosted with no corresponding cost increase. To ensure an increase in the efficiency and global utilization of solar technologies, it is recommended that some improvements be made. For crystalline technologies, optimization of the cost of production is needed, as well as the implementation of new silicon materials. Though the production of thin film solar cells has drastically reduced over the years, introducing some improved deposition techniques and the application of advanced materials and concepts could help boost its efficiency. Furthermore, for the newer technologies such as dye sensitized solar cells, finding newer compounds and cell stability improvements could become beneficial in its future implementation.
-
-
-
CONCLUSION:
Solar PV technology has had a positive impact in terms of ensuring sustainability and providing clean electricity. Though
newer PV materials are being developed recently, the major determinant to the widespread application of the technology remains an increase in efficiency. From the review, data has shown that first generation solar technologies continually dominate the market with low cost solar cells and high efficiencies. Thin-film PV technologies have been utilized because of their low material and manufacturing costs, but are less mature than first generation PV and has recently been undergoing a declining market share. Emerging technologies on the other hand are yet to be commercialized on a larger scale but holds a promising future in the power industry due to its high efficiency, low cost and weight. Overall, there is need for more research and development funding in order to produce solar modules that are best suited to a fully decarbonized and environmentally safe economy.
REFERENCES
-
Energy Informative (n.d.). Best Thin Film Solar Panels Amorphous, Cadmium Telluride or CIGS? Retrieved October 20, 2019 from https://energyinformative.org/best-thin-film-solar-panels-amorphous- cadmium-telluride-cigs/
-
Tian, J. and Cao G. (2013). Semiconductor quantum dot-sensitized solar cells. Nano Reviews, 4, 22578. https://doi.org/10.3402/nano.v4i0.22578
-
Haley U.C.V. and Schuler D.A. (2011). Government Policy and Firm Strategy in the Solar Photovoltaic Industry. California Management Review, 54 (1), 18-20. Accessed December 12 2019 from https://www.researchgate.net/publication/263350965_Government_P olicy_and_Firm_Strategy_in_the_Solar_Photovoltaic_Industry
-
Sharma S., Jain K.K. and Sharma A. (2015). Solar Cells: In Research and ApplicationsA Review. Materials Sciences and Applications, 6, 1145-1155. http://dx.doi.org/10.4236/msa.2015.612113
-
Rathore N., Panwar N.L., Yettou F. and Gama A. (2019). A Comprehensive Review on Different Types of Solar Photovoltaic Cells and Their Applications. International Journal of Ambient Energy. DOI: 10.1080/01430750.2019.1592774
-
Zhao J., Wang A. and Green M.A. (1998). 19.8% Efficient Honeycomb Textured Multicrystalline And 24.4% Monocrystalline Silicon Solar Cells. Applied Physics Letters, 73(14). DOI: 10.1063/1.122345
-
Circuit Globe (n.d.). Photovoltaic or Solar Cell. Accessed April 27, 2020 from https://circuitglobe.com/photovoltaic-or-solar-cell.html
-
Zimmer T. (n.d.). Photovoltaic Cell Types. Retrieved October 15, 2019 from
https://www.labri.fr/perso/billaud/Helios2/resources/en06/Chapter_6
_UB_zimmer.pdf
-
Green, M.A. (2011). Radiative efficiency of state of the art photovoltaic cells. Progress in Photovoltaics: Research and Applications. DOI: 10.1002/pip.1147
-
Sendy A. (2017). Pros and Cons of Monocrystalline vs Polycrystalline Solar Panels. Retrieved October 31, 2019 from https://www.solarreviews.com/blog/pros-and-cons-of- monocrystalline-vs-polycrystalline-solar-panels
-
Dobrzanski L.A.,Drygala A., Giedroc M. and Macek M. (2012). Monocrystalline Silicon Solar Cells Applied In Photovoltaic System. Journal of Achievements in Materials and Manufacturing Engineering, 53(1), 1-12. Retrieved October 29, 2019 from https://www.researchgate.net/publication/289642017_Monocrystallin e_silicon_solar_cells_applied_in_photovoltaic_system
-
Tascioglu A., Taskin O. and Vardar A. (2016). A Power Case Study for Monocrystalline and Polycrystalline Solar Panels in Bursa City, Turkey. Retrieved October 29, 2019 from https://www.researchgate.net/publication/298432080_A_Power_Cas e_Study_for_Monocrystalline_and_Polycrystalline_Solar_Panels_in
_Bursa_City_Turkey
-
Kato T., Wu J., Hirai Y., Sugimoto H. and Bermudez V. (2019). Record Efficiency for Thin-Film Polycrystalline Solar Cells Up to 22.9% Achieved by Cs-Treated Cu(In,Ga)(Se,S)2. IEEE Journal of Photovoltaics, 9(1), 325-330. DOI:10.1109/JPHOTOV.2018.2882206
-
Dena German Energy Agency, "Information about German renewable energy, industries, companies and product(Federal Ministry of
Economics and Technology)", pp. 41, 2013-2014, ISIN:
B002MNZE4U
-
Saga, T. (2010) Advances in Crystalline Silicon Solar Cell Technology for Industrial Mass Production. NPG Asia Maerials, 2, 96-102. http://dx.doi.org/10.1038/asiamat.2010.82
-
Indiamart (n.d.). Tata 250 Watt Polycrystalline Solar Panel. Retrieved November 1, 2019 from https://www.indiamart.com/proddetail/250- watt-polycrystalline-solar-panel-16449465433.html
-
Zsiboracs H., Palyi B., Pinter G., Popp J., Balogh P., Gabni Z., Peto K., Farkas I., Baranyi N. H. and Bai A. (2016). Technical-Economic Study of Cooled Crystalline Solar Modules. Solar Energy, 140, 227- 235. https://doi.org/10.1016/j.solener.2016.11.009. https://iopscience.iop.org/article/10.1088/1742-6596/758/1/012001
-
Khalis M., Masrour R., Khrypunov G., Kirichenko M., Kudiy D. and Zazoui M. (2016). Effects of Temperature and Concentration Mono and Polycrystalline Silicon Solar Cells: Extraction Parameters. Journal of Physics: Conference Series.
-
Powalla M., Paetel S., Hariskos D., Wuerz R. Kessler F., Lechner P., Wischmann W. and Friedlmeier T.M (2017). Advances in Cost- Efficient Thin-Film Photovoltaics Based on Cu (In,Ga)Se2. Clean EnergyReview, 3(4), 445-451.
https://doi.org/10.1016/J.ENG.2017.04.015
-
Imamzai, M., Aghaei, M., Hanum Md Thayoob, Y. and Forouzanfar,
M. (2012) A Review on Comparison between Traditional Silicon Solar Cells and Thin-Film CdTe Solar Cells. Proceedings of National Graduate Conference (NatGrad 2012), Tenaga Nasional Universiti, Putrajaya Campus, 8-10 November 2012, 1-5. Retrieved October 20, 2019 from
https://www.scirp.org/(S(i43dyn45teexjx455qlt3d2q))/reference/Refe rencesPapers.aspx?ReferenceID=1636825
-
Yang T.C. (2016). Transparent Conducting Aluminium Doped Zinc Oxide for Silicon Quantum Dot Solar Cell Devices in Third Generation Photovoltaics. Doctoral Thesis. DOI: 10.13140/RG.2.2.13188.22407
-
Energy Informative (n.d.). Best Thin Film Solar Panels Amorphous, Cadmium Telluride or CIGS? Retrieved November 1, 2019 from https://energyinformative.org/best-thin-film-solar-panels-amorphous- cadmium-telluride-cigs/
-
Kabir M.I., Ibarahim Z., Bin Sopian K. and Amin N. (2011). A Review on Progress of Amorphous and Microcrystalline Silicon Thin-Film Solar Cells. Recent Patents on Electrical Engineering 2011, 4, 50-62.
DOI: 10.2174/1874476111104010050
-
Kaminski P. M., Lisco F., and Walls J.M. (2014). Multilayer broadband antireflective coatings for more efficient thin film CdTe solar cells, IEEE Journal of Photovoltaics, 4(1), 452456. DOI: 10.1109/JPHOTOV.2013.2284064
-
McCandless B. E. and Sites J. R. (2003). Cadmium telluride solar cells in Handbook of Photovoltaic Science and Engineering, A. Luque and S. Hegedus, Eds., pp. 617662, John Wiley & Sons Ltd., West Sussex, England.
-
Basol B.M. and McCandless (2014). Brief review of cadmium telluride-based photovoltaic technologies. Journal of Photonics for Energy, 4(1), 1-8. DOI: 10.1117/1.JPE.4.040996
-
Wild E (2014). First Solar sets thin film module efficiency world record of 17.0 percent. Retrieved October 25, 2019 from https://www.solarpowerworldonline.com/2014/03/first-solar-sets- cdte-thin-film-module-efficiency-world-record-17/
-
Kato K., Hibino T. and Komoto K. (2001). Life-cycle analysis on thin- film CdS/CdTe PV modules. Solar Energy Materials and Solar Cells 67(1-4):279-287. DOI: 10.1016/S0927-0248(00)00293-2
-
Dharmada I.M., Bingham P.A., Echendu O.K., Salim H.I., Drriffeel T., Dharmadasa R., Sumanasekera G.U., Dharmasena R.R., Dergacheva M.B., Mit K.A., Urazov K.A., Bowen L., Walls M. and Abba A. (2014). Fabrication of CdS/CdTe-Based Thin Film Solar Cells Using an Electrochemical Technique. Coatings 2014 (4), 380- 415. DOI:10.3390/coatings4030380
-
Ong K., Agileswari R., Maniscalo B., Arnou P., Kumar C, Bowers J. and Marsadek M. (2018). Review on Substrate and Molybdenum Back Contact in CIGS Thin Film Solar Cell. International Journal of Photoenergy Volume 2018, Article ID 9106269. https://doi.org/10.1155/2018/9106269
-
Ramanujam J. and Singh U. P. (2017). Copper Indium Gallium Selenide Based Solar Cells Review. Energy & Environmental Science 9(11), 1306-1319. DOI: 10.1039/C7EE00826K
-
Devi N, Aziz A. and Datta S. (2018). Numerical modelling of CIGS/CdS solar cell. AIP Conference Proceedings 1953, 100031 (2018); https://doi.org/10.1063/1.5032967
-
Powalla M., Paetel S., Hariskos D., Wuerz R., Kessler F., Lechner P., Wischmann W. and Friedlmeier T.M. (2017). Advances in Cost- Efficient Thin-Film Photovoltaics Based on Cu (In,Ga)Se2. Engineering, 3 (4), 445-551. Retrieved October 29, 2019 from https://www.sciencedirect.com/science/article/pii/S20958099173060 33
-
Sagadevan S. (2012). Recent Trends on Nanostructures Based Solar Energy Applications: A Review. Rev. Advance Material Sci. 34 (2013), 44-61. Retrieved October 27, 2019 from http://www.ipme.ru/e- journals/RAMS/no_13413/06_13413_suresh.pdf
-
Gur I. (2006). Nanocrystal Solar Cells. PhD Dissertation, University of California, Berkeley. Retrieved October 27, 2019 from https://www.osti.gov/servlets/purl/922721
-
Anthony S. (2011). Cheap, Ink-Based Printable Nanocrystal Solar Cells Become a Reality. Retrieved October 29, 2019 from https://www.extremetech.com/extreme/103477-cheap-ink-based- printable-nanocrystal-solar-cells-become-a-reality
-
Gusain A., Faria R.M and Miranda P.B. (2019). Polymer Solar Cells Interfacial Processes Related to Performance Issues. https://doi.org/10.3389/fchem.2019.00061
-
Abdulrazzaq O.A., Saini V., Bourdo S., Dervishi E. and Biris A.S. (2013). Organic Solar Cells: A Review of Materials, Limitations, and Possibilities for Improvement. Retrieved October 18, 2019 from https://www.researchgate.net/publication/263732376_Organic_Solar
_Cells_A_Review_of_Materials_Limitations_and_Possibilities_for_I mprovement
-
Luceno-Sanchez J.A., Diez-Pascual A.M. and Capilla R.P. (2019). Materials for Photovoltaics: State of Art and Recent Developments. International Journal of Molecular Sciences, 20 (976).
DOI:10.3390/ijms20040976
-
Gusain A., Faria R.M. and Miranda P.B. (2019). Polymer Solar CellsInterfacial Processes Related to Performance Issues. Frontiers in Chemistry. Retrieved January 19, 2019 from https://www.frontiersin.org/articles/10.3389/fchem.2019.00061/full
-
Papageorgiou N (2013). Ecole Polytechnique Federale De Lausanne News. Retrieved November 3, 2019 from https://actu.epfl.ch/news/dye-sensitized-solar-cells-rival- conventional-ce-2/
-
Hossam-Eldin A., Refaey M. and Farghly A. (2015). A Review on Photovoltaic Solar Energy Technology and its Efficiency. 17th International Middle-East Power System Conference (MEPCON'15), 1-8. Retrieved November 3, 2019 from https://www.researchgate.net/publication/287792329_A_Review_on
_Photovoltaic_Solar_Energy_Technology_and_its_Efficiency
-
Wikiedia (2020). (Dye-sensitized solar cell. Accessed April 8, 2020 from https://en.wikipedia.org/wiki/Dye-sensitized_solar_cell
-
Gong, J., Liang, J., Sumathy, K. (2012). Review on dye-sensitized solar cells (DSSCs): Fundamental concepts and novel materials. Renewable and Sustainable Energy Reviews, 16(8), 58485860. https://doi.org/10.1016/j.rser.2012.04.044
-
Zhou D., Zhou T., Tian Y., Zhu X. and Tu Y. (2018). Perovskite-Based Solar Cells: Materials, Methods, and Future Perspectives. Journal of Nanomaterials, 2018, 1-15. Retrieved April 30, 2020 from https://www.hindawi.com/journals/jnm/2018/8148072/
-
Zhou H., Chen Q. and Li G. (2014). Interface Engineering of Highly Efficient Perovskite Solar Cells. Science, 345(6196), 542546. DOI: 10.1126/science.1254050
-
Rodrigues E.M. Meelicio R., Mendes V.F. and Catalao J.P. (2011). Simulation of a Solar Cell Considering Single-Diode Equivalent Circuit Model. RE&PQJ, 1(9), 369-373. Retrieved November 11, 2019 from http://icrepq.com/icrepq%2711/339-rodrigues.pdf
-
Razykov T.M., Ferekides CS., Morel D., Stefanakos E., Ullal H.S.and Upadhyaya H.M. (2011). Solar Photovoltaic Electricity: Current Status and Future Prospects. Solar Energy 85, 15801608. Retrieved November 3, 2019 from https://doi.org/10.1016/j.solener.2010.12.002
-
AzoCleantech (2016). Solar (PV) Panel Comparison for Efficiency, Material, Voltage [online]. Retrieved April 30, 2020 from https://www.azocleantech.com/article.aspx?ArticleID=603
-
Bhati N., Nath A., Raman R., Rakshit J.K. and Sarkar M.B. (2019). A Review Paper on Recent Trends of Solar Cell Technology, Materials and Efficiencies. IC_WEB_BIMSTEC_2019. Retrrieved 5 May, 2020
from https://www.researchgate.net/publication/338036460_A_Review_Pa per_on_Recent_Trends_of_Solar_Cell_Technology_Materials_and_ Efficiencies
-
Amin N., Shahamadi S.A., Chelvanathan P. and Rahman K.S. (2017). Solar Photovoltaic Technologies: From Inception toward the Most Reliable Energy Resource. Encyclopedia of Sustainable Technologies, 3 (2017), 11-26. Retrieved May 2, 2020 from https://www.sciencedirect.com/science/article/pii/B97801240954891 00922?via%3Dihub
-
Solar Facts and Advice (n.d). Sale of CIGS Solar Cell Panels Expected to Reach $1 Billion by 2013. [online] Retrieved May 2, 2020 from https://www.solar-facts-and-advice.com/CIGS-solar-cell.html
-
Energysage (2019). Types of thin film solar panels [online]. Retrieved May 3, 2020 from https://www.energysage.com/solar/101/about- solar-panels/thin-film-solar-panels-amorphous-cadmium-telluride- and-cigs/
-
Mora-Sero I. (2018). How Do Perovskite Solar Cells Work? Joule, 2 (4), 585-587. Retrieved 2 May, 2020 from https://www.sciencedirect.com/science/article/pii/S25424351183013 51
-
Gaewdang T., Wongchareon N. and Wongchareon T. (2012). Characterisation of CdS/CdTe Heterojunction Solar Cells by Current- Voltage Measurements at Various Temperatures under Illumination. Energy Procedia, 15 (2012), 299-304. Retrieved May 2, 2020 from https://www.sciencedirect.com/science/article/pii/S18766102120037 12
-
Kumar P.M., Das A., Seban L. and Nair R.G. (2018). Perovskite Photovoltaics, Basic to Advanced Concepts and Implementation: Chapter 8 – Fabrication and Life Time of Perovskite Solar Cells. Academic Press, 231-287. Retrieved May 2, 2020 from