
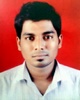
- Open Access
- Authors : Niranjan Dilip Patil
- Paper ID : IJERTV11IS010020
- Volume & Issue : Volume 11, Issue 01 (January 2022)
- Published (First Online): 13-01-2022
- ISSN (Online) : 2278-0181
- Publisher Name : IJERT
- License:
This work is licensed under a Creative Commons Attribution 4.0 International License
3d Finite Element Analysis of Dam using Direct Time History Method- A Review
Niranjan Dilip Patil1
1Assistant Professor,
Mahatma Gandhi College of Engineering and Technology, Navi Mumbai,University of Mumbai
Abstract:- The seismic crack propagation of concrete and gravity dams with initial cracks at the various places in the dams has rarely been studied during strong earthquakes, therefore it is ne- cessity to review the analysis of its performance. Many concrete gravity dams have been constructed throughout the world at hy- dropower, navigation and flood control projects. Some of these structures are located where the maximum credible earthquake motion is now considered to be quite strong. This is disconcerting as most concrete dams were designed for earthquake loading with a direct timehistory method analysis using a regional seismic coefficient. The finite element analysis can carried out on the tall- est monolith of the dam and can be assessed the structure under static and dynamic load cases for usual, unusual and extreme load combinations.Dynamic time-history analyses are used to as- sess earthquake performance of the highest monolith of the dam. The step-by-step time history analysis provides important insight into the dynamic behaviour of the dam, accounts for transitory nature of earthquake ground shaking, and helps in identifying potential modes of failure.
Key Words : Time history method,Finite Element Analysis,con- crete gravity dam,seismic performance,dynamic crack propogation.
-
INTRODUCTION
Performance of concrete dams is conducted based on stability and stress checks for various operating conditions including flood and seismic events. This report is an effort to analyse non overflow and overflow sections of a dam for all operating conditions mentioned in various national and international standards. A case study of an under-construction dam in Him- alayan region being developed on a tributary of river Ganga has been considered. Since, the dam has not been constructed yet, the name of the dam has not been mentioned to avoid any issues related ownership and design changes. Dam lies in high seismic zone which requires performance-based design and evaluation based on specified levels of seismic hazard. Tradi- tional design and evaluation procedures may be used for vari- ous components of the project but for critical facilities like dam, should be designed to prevent sudden collapse (even though the structure may suffer severe damage), to limit dam- age to a repairable level, or to maintain functionality immedi- ately after the earthquake
Dam blocks are confined between rock abutments on left and right banks of river. There are contraction joints without any gap between the blocks. Adequate water seals shall be pro- vided at the joint locations on the upstream face and at bottom of dam to avoid any seepages through them. There can be transfer of stresses from one block to another through these joints but dam shall be stable across the river flow due to the confinement. A separate analysis shall be carried out for the forces in valley direction(across river flow) to evaluate high
stresses zones in dam. The reinforcement shall be then com- puted where tensile stresses exceeds permissible limits. Per- missible tensile stresses are mentioned later in the report. This report deals with the stability of dam blocks for the all the static and dynamic seismic forces along the river flow. The analysis has been carried out to ensure that certain mandatory requirements mentioned later in the report are met and that the dam meets its safety requirement and achieves its performance objectives. The mandatory requirements ensure that the dam is safe against all imposed loading including the maximum earthquake and that the stresses are within permissible limits. Finite element analysis software MIDAS FEA NX has been used to carryout present analysis.
Fig. 1.1 Dam Plan
Fig1.2 Dam Body Upstream View
Fig1.3 Dam Body Sideview L Section
-
DAM OPERATING CONDITIONS
Dams in general are designed and evaluated for three basic op- erating conditions which can occur during the life span of dam: Usual, Unusual and Extreme. In the present analysis, the dam- spillway model has been subjected to these load combinations and the corresponding stress and displacement response of the structure has been obtained. Usual static loading conditions re- fer to all applicable static loads that may exist during the nor- mal operation of the structure. These loads normally comprise of dead loads, water pressure corresponding to full reservoir level(FRL) and normal tail water level, normal uplift. Unusual static loading conditions refer to all applicable static loads that may exist during the design flood condition with all gates op- erating. These loads would generally comprise of dead loads, water pressure corresponding to maximum water level (MWL) during flood and tail water corresponding to project maximum flood(PMF) condition, uplift pressure and applicable silt loads. Normal operating conditions along with blocked drain- age system (which maximizes the uplift effects) is also taken as Unusual static loading. Unusual dynamic loading implies Design basis earthquake(DBE) level earthquake loads acting on the dam along with Usual static loads. Extreme dynamic loading implies maximum credible earthquake(MCE) level earthquake loads acting on the dam along with Usual static loads. Load combinations corresponding to these conditions are specified in IS:6512 Part-I, 1984 :
-
Combination A Dam Completed but no reservoir im- pounding.
-
Combination B Reservoir at FRL (all gates closed), mini- mum tail water level, drains operative.
-
Combination C Reservoir at MWL, maximum tail water level, drains operative.
-
Combination D- Load Combination A with DBE Earth Quake
-
Combination E- Load Combination B with DBE Earth Quake
-
Combination F Reservoir at MWL full (all gates open),
maximum tail water level, drains in operative.
-
Combination G Load Combination E under Drains Inop- erative uplift condition A separate load case H has also been analysed for extreme dynamic loading.
-
PERMISSIBLE TENSILE STRESSES IN UNREINFORCED CONCRETE FOR STATIC LOAD
CONDITIONS
Small values of tension on the downstream face of dam may be permitted since it is very improbable that a fully constructed dam is kept empty and downstream cracks which are not ex- tensive and for limited depths from the surface may not be det- rimental to the safety of the structure. It is recommended to limit tension on downstream face to.5MPa.
If tension exceeds this limit, reinforcement shall be provided. When the induced tensile stresses on the upstream face are less than following permissible tensile stresses, there is no require- ment of reinforcement in concrete:
Load Combination A and B No tension Load Combination C – .01fc
Load combinations F – .02fc
-
MATERIAL PROPERTIES
Based on geological baseline report(GBR), elastic modulus of founding rock has been considered as 2.7Gpa. Cohesion be- tween rock and concrete is considered as 210 kN/m2 . Material damping has not been considered to get maximum response during dynamic seismic cases which shall be on conservative side. Rock has been considered as massless to get reactions due to weight of concrete only to be considered in stability analysis. Elastic modulus of rock mentioned in GBR is ob- tained from plate load test (jacking test). Foundation load dis- tribution can also be sensiive to the values selected for foun- dation modulus, which can affect foundation sliding stability calculations. Therefore, it is important to make good estimates of the potential range of foundation modulus values. Several methods should be used to establish these estimates.
Since we have deformation modulus of rock from Jacking test, 80% of Econcrete may be considered.
EF:Analysis_Dynamic = 80% of Econcrete i.e 20Gpa has been considered in the present analysis.
-
SOFTWARE MODELLING
MIDAS FEA NX, have been used to model the Left NOF Block structure. The mass concrete structure and surrounding rock has been modelled using Self-generating solid mesh in MIDAS. Mesh size of 2m has been used for dam body and coarser for surrounding rock in MIDAS model. After conduct- ing, various sensitivity studies it was found that mesh size of surround rock has no major impact on induced stresses of dam block. Hence, coarser mesh size has been adopted for rock to decreases analysis time. Finite element time history analysis consumes lot of computer memory and requires more analysis time than static analysis. Hence, reduced number of elements in model are used without compromising stress values and dis- tribution pattern. The various views of model have been shown below:
Fig5.1 Dam Block
Fig5.2 Dam Block with Meshing
For dynamic analysis, realistic results shall be obtained when mass of foundation is also considered along with material damping. Modelled depth below dam founding level where the seismic wave is acting plays an important role in getting close to actual results. However, getting these parameters precisely is another challenge. To be on safer side, foundation with no mass and 0% damping has been considered. It will result in higher induced stresses and reactions than actual for dynamic seismic loads.
-
Support Conditions
For dynamic analysis, it is recommended to use semi-un- bounded foundation and fluid domains with appropriate radi-
ation conditions at the domain boundaries to allow propaga- tion of outgoing waves. To simulate it, viscous boundary dampers can be provided in MIDAS. However, reactions to be used in stability analysis could not be obtained for these vis- cous restraints. There is another option in MIDAS i.e. grid forces which gives induced forces in each node of the model. The summation of these forces will give the total reaction. However, in ground motion time history analysis , to get reac- tions for each time step for all the nodes in model is near to impossible. A sensitivity study has been carried out consider- ing viscous boundaries and restraint ground boundaries for load combination D- End of construction + DBE earthquake. Induced major principal stresses in NOF block with viscous boundary:
Fig 5.3 Dam Block Support Conditions
Induced major principal stresses in NOF block with restraint ground boundary:
Fig 5.4 Dam Block Support Conditions Restraint.
-
Applied Loads
The following loads have been considered for 3D finite ele- ment analysis of the dam blocks.
Dead Load / Self Weight of the structure
Self-weight of structure automatically incorporated in MIDAS. Deformation behaviour of NOF block for selfweight.
Fig 5.5 Self weight applied
Fig 5.6 Deformation behaviour of OF block for self-weight
U/S Hydrostatic Loads
A linear distribution of pressure variation has been considered. The hydrostatic pressure is maximum at the base of the struc- ture and it gradually reduced to zero at the FRL/MWL. Hydrostatic Force -Upstream Face
Maximum Reservoir Level(FRL) = El 1267m Minimum elevation of base = El 1218m Depth of Water, y = 1267 1218 = 49m
Maximum pressure ordinate at upstream face, pmax = 10 x 49
= 490 kN/m2
Uplift & Normal Tail Water Level
Uplift forces are applied on bottom face of raft. It is corre- sponding to FRL on u/s face For drain operative condition, up- lift has been reduced to 1/3rd of the head difference between the upstream and downstream water levels at drainage gallery location. The uplift pressure is considered not affected by earthquake.
-
-
ANALYSIS
-
The finite element model described earlier has been subjected to various loads mentioned earlier in the report and a detailed analysis is carried out to determine resulting deformations and stresses within the dam. While the static loads are applied as one-time loading, the earthquake loads (both the DBE and the MCE) are applied in the form of appropriate time histories of horizontal and vertical ground acceleration. Deformation and stress results are obtained for each time step and maximum values are extracted to be combined with the corresponding values from the static load cases.
The following general postulates have been considered
-
The stress is proportional to strain and the material follows Hooke's law.
-
Concrete and foundation rock can be modelled as elastic, homogeneous and isotropic material.
-
Hydrostatic pressure acting on the dam is represented as sur- face pressure acting on the water face of the model.
-
The water-dam interaction has been modelled by the added mass concept recommended by Westergard.
-
The standard approach to account for the effects of founda- tion interaction has been adopted to analyse the combined dam-foundation system by including an appropriate region of
the rock in the finite element model.
-
For earthquake loads, a time-history dynamic analysis is performed using recommended time histories of horizontal and vertical ground acceleration for appropriate level of seis- mic event. The time step used in the dynamic analysis is 0.05sec. The selected time step meets the commonly used cri- terion for such analysis.
-
In case results of linear elastic analysis indicate significant damage to the structure (stresses significantly exceeding the permissible limits or exceeding in large regions) decision based upon rational engineering judgment will be taken to pro- vide a reasonable estimate of the expected inelastic behaviour or damage.
Time history analysis
Concrete hydraulic structures behave in a less ductile manner during earthquake as compared to RCC building. Such behaviour combined with complicated structure foundation and structure -water interaction requires time history analysis. Linear time history analysis calculates the solution to the dy- namic equilibrium equation for the structural behavior (dis- placement, element force etc.) at an arbitrary time using the dynamic properties of the structure and applied loading when a dynamic load is applied. The Modal superposition method and Direct method are used for linear time history analysis.
The mode superposition method assumes the structural displacement as a linear combination of orthogonal displace- ments. Using this, a more simplified time integral function can be used to calculate the dynamic response for a selected mode. The mode superposition method is used in many structural analysis programs and is an effective way to calculate the dy- namic response for the linear dynamic analysis of large struc- tures with little computational cost. However, the accuracy of the total response depends on the number of used natural modes and so, the number of modes used in the calculation need to be selected appropriately.
The direct method is a time history analysis that uses the DOF of the total analysis area as a variable. The dynamic equi- librium equation for the total DOF can be integrated gradually with time to find the solution. The solution is found for each time stage without any form change to the equilibrium equa- tion and various integration methods can be used. The direct integration method conducts the analysis for all time stages and the number or time stages is proportional to the analysis time.
The direct method has been adopted for time hitory anal- ysis in the present report. Time history analysis has been car- ried out for load cases D,E G and H.
Ground motion has been defined for 25 seconds with time increment of .05sec.
It infers total 500-time steps are considered in the analy- sis. Arrival time is considered as 0 seconds as acceleration val- ues in time history curve touches maximum in time period of 0 seconds to 25 seconds.
Primary objective of this report is to observe principal stresses in unreinforced mass concrete and check the stability at founding level. Reinforcement in piers, breastwall, stair- case, control building and other flexural components shall be evaluated seperately.
-
Time history of displacement
To assess the dynamic response of the dam, time histories of displacement and acceleration response of the structure are ob- tained for simultaneous application of horizontal and vertical ground motions corresponding to the DBE and the MCE. Time history of displacement
Fig no. 6.1 NOF section Maximum relative displacement along river flow (DBE)
Fig no. 6.2 Time history plot of u/s dam top node with maximum defor- mation of .6312mm: Max. deformation at 15 sec.
Fig no. 6.3 NOF section Maximum relative vertical displacement (DBE)
Fig no. 6.4 Time history plot of node with maximum deformation of .1907mm
-
Time history of displacement for OF Section
The maximum relative displacement distribution in case of DBE for OF section is shown below
Fig no. 6.5 OF section Maximum relative displacement along river flow (DBE)
Fig no. 6.6 Time history plot of dam top node with maximum deformation of 5.87mm
-
Time history of maximum acceleration
The maximum acceleration in river flow direction (NOF sec- tion) corresponding to DBE level of ground motion is:
Fig no. 6.7 NOF section Maximum acceleration along river flow (DBE)
Fig no. 6.8 Time history plot of node at dam top with maximum accelera- tion of 4.25 m/sec2
Time history of acceleration for OF Section
The maximum acceleration in river flow direction (OF sec- tion) corresponding to DBE level of ground motion is:
Fig no. 6.9 OF section Maximum acceleration along river flow (DBE)
Fig no. 6.10 Time history plot of dam top node with maximum acceleration of 11.55 m/sec2
The results of the analysis are presented in the form of dis- placements and stresses for individual loads or load combina- tions, as applicable. In case of dynamic analysis, the results are normally presented as envelopes of maximum stresses, time history of displacements, and time history of factor of safety against sliding. The results for various loadings/load combinations are discussed below. +ve induced stresses are tensile and -ve induced stresses are compressive. The results of the analysis are presented in the form of displacements and stresses for individual loads or load combinations, as applica- ble.
7. CONCLUSIONS
The finite element analysis has been carried out on the tallest monolith of the dam and assesses the structure under static and dynamic load cases for usual, unusual and extreme load com- binations. Dynamic time-history analyses are used to assess
earthquake performance of the highest monolith of the dam. The step-by-step time history analysis provides important in- sight into the dynamic behaviour of the dam, accounts for tran- sitory nature of earthquake ground shaking, and helps in iden- tifying potential modes of failure.
REFERENCES
-
Miles, J. W. : On the generation of surface waves by shear flows, J. Fluid Mech., Vol. 3, Pt. 2, pp. 185-204, 1957.
-
Miche, M. : Amortissement des houles dans le do-maine de leau peu profonde, La Houile Blanche, No. 5, pp. 726-745, 1956.
-
Gresho, P. M., Chan, S. T., Lee, R. L. and Upson, C. D. : A modified finite element method for solving the time- dependent incompressible Navier-Stokes equations, part 1, Int. J. Numer. Meth. Fluids, Vol. 4, pp. 557-598, 1984.
-
Shepard, F. P. and Inman, D. L. : Nearshore water circulation related to bottom topogrphy and wave refraction, Trans. AGU., Vol. 31, No. 2, 1950.
-
Smith, W. : Cellular phone positioning and travel times estimates, Proc. of 8th ITS World Congress, CD-ROM, 2000.
-
Direct-Finite-Element Method for Nonlinear Earthquake Analysis of Concrete Dams Including DamWaterFoundation Rock Interaction by A K Chopra and Arnkjell Løkke
-
State-of-Practice for the Nonlinear Analysis of Concrete Dams 2013,
U.S. Department of the Interior Bureau of Reclamation Technical Ser- vice Center Denver, Colorado
-
USACE (US Army Corps of Engineers, 1995) – Gravity Dam Design Report EM 1110-2-2200
-
USACE (US Army Corps of Engineers, 2003) – Time history Analysis of Concrete Hydraulic Structure EM 1110-2-6051
-
USACE (US Army Corps of Engineers, 2007) – Time history Analysis of Concrete Hydraulic Structure EM 1110-2-6053
-
IS 6512 Criteria for Design of Solid Gravity Dams, IS: 6512 – 2019
-
IS 1893 Criteria for Earthquake Resistant design of structures. IS: 1893:1984